the Creative Commons Attribution 4.0 License.
the Creative Commons Attribution 4.0 License.
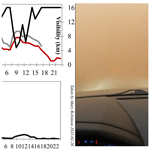
Spatial, temporal, and meteorological impact of the 26 February 2023 dust storm: increase in particulate matter concentrations across New Mexico and West Texas
Mary C. Robinson
Kaitlin Schueth
Karin Ardon-Dryer
The southwestern portions of the United States experience dust events frequently due to arid and semi-arid environments and close proximity to multiple deserts. On 26 February 2023, a dust event was initiated in New Mexico due to strong winds aloft mixing down to the surface. The dust intensified as it moved eastward into West Texas and developed into a dust storm (visibility <1 km) for multiple locations. This study examined the meteorological characteristics of this dust storm using 28 meteorological stations and examined the impacts on PM2.5 and/or PM10 (particulate matter with an aerodynamic diameter <2.5 and 10 µm) concentrations using 19 air quality stations. The dust event lasted up to ∼16 h; dust storm conditions lasted from 5 to 120 min. The highest wind speed and wind gust recorded during the dust episode were 27 and 37 m s−1, respectively. This dust had a strong impact on the air quality in the area, as very high PM values were recorded across the region, and nine of the PM stations exceeded the United States Environmental Protection Agency (EPA) daily threshold. The maximum hourly PM2.5 and PM10 concentrations recorded were 518 and 9983 µg m−3, respectively. These concentrations at the peak of the dust were an order of magnitude higher than the minimum hourly PM2.5 and PM10 concentrations recorded on the dust day. The highest hourly PM10–PM2.5 concentration recorded was 760 ± 1000 µg m−3, while the lowest hourly PM2.5 PM10 concentration measured was 0.05 ± 0.01.
- Article
(7107 KB) - Full-text XML
-
Supplement
(1415 KB) - BibTeX
- EndNote
Dust events are a meteorological phenomenon that occur when dust particles are suspended in the atmosphere by strong winds, and they reduce visibility. Visibility during dust events ranges between 1 and 10 km, while dust storms are classified as such when visibility drops below 1 km (WMO, 2019). Dust events are prominent in arid and semi-arid environments but can influence other types of environments (Middleton et al., 2019). The strength of the dust event is dependent on multiple factors. Strong winds are very important for the initiation of dust events and/or storms, which are generally caused by a synoptic or convective meteorological disturbance (Kelley and Ardon-Dryer, 2021; Robinson and Ardon-Dryer, 2024; Sandhu et al., 2024). Drought conditions (Arcusa et al., 2020) and vegetation cover (Stout, 2001) are also important factors that contribute to dust initiation.
There are multiple hazards associated with dust events. Lower visibility increases the chances of traffic and aviation accidents (Li et al., 2018; Al Kheder and Al Kandari, 2020; Tong et al., 2023). The blowing dust particles can cause abrasions and damage to crops (Middleton, 2019) and health complications for livestock (Mu et al., 2013). High particulate matter (PM) concentrations can result in poor air quality (Achakulwisut et al., 2017; Ardon-Dryer and Kelley, 2022; Ardon-Dryer et al., 2023a), leading to different health and well-being impacts. Exposure to dust particles during dust events can cause significant health problems such as respiratory issues (Toure et al., 2019; Herrera-Molina et al., 2024), cardiovascular issues (Goudarzi et al., 2017), stroke (Schweitzer et al., 2018), toxemia of pregnancy (Bogan et al., 2021), and Valley fever (Tong et al., 2022; Gorris et al., 2023) and can even lead to death (Pérez et al., 2008; Malig and Ostro, 2009). Therefore, the United States Environmental Protection Agency (EPA) and the World Health Organization (WHO) have set standards for PM10 and PM2.5 (particulate matter with an aerodynamic diameter below 10 and 2.5 µm, respectively) to determine poor air quality conditions. The EPA PM2.5 and PM10 daily standards are 35 and 150 µg m−3, respectively (EPA, 2023), while the updated WHO daily thresholds are 15 and 45 µg m−3 for PM2.5 and PM10, respectively (WHO, 2023).
Dust events and storms occur across the United States (Tong et al., 2023), mainly across the southwestern portions, due to their drier and warmer conditions with low soil moisture from desert regions (Achakulwisut et al., 2017). Among the states, the most susceptible to dust events are Arizona (Nickling and Brazel, 1984; Lei et al., 2016; White et al., 2023); southern California (Bach et al., 1996; Evan, 2019; Huang et al., 2022); Utah (Hahnenberger and Nicoll, 2012; Hennen et al., 2022); and those across the Great Plains, mainly the southern Great Plains area including New Mexico and Texas (Kandakji et al., 2020; Hennen et al., 2022; Ardon-Dryer et al., 2023b; Robinson and Ardon-Dryer, 2024). The multiple dust sources in the region, mainly cropland, contribute to the high number of dust events (Lee et al., 2012). In a recent study, Robinson and Ardon-Dryer (2024) found an average of 22 dust events annually (between 2000 and 2021) across four dust-prone regions in West Texas. Most of the dust events in the region occur in the spring to early-summer months, mainly due to synoptic disturbances, while a smaller percentage of dust events are formed by convective disturbances, and rarely are dust events formed by combinations of these two disturbances (Robinson and Ardon-Dryer, 2024).
The air quality across West Texas and New Mexico is good overall (Zanobetti and Schwartz, 2009; Kelley et al., 2020). Anthropogenic pollution such as industrial facilities and transportation emissions, which can lead to ozone, can be found mainly in the two large urban cities of El Paso, Texas, and Albuquerque, New Mexico (Gaffney et al., 1997; Chen et al., 2012; Kavouras et al., 2020; Craig et al., 2020; Karle et al., 2020; Van Pelt et al., 2020; Huang et al., 2023). The entire area is impacted by dust events and dust storms which lead to an increase in PM and degradation of the air quality (Tong et al., 2012; Stout, 2015; Herrera-Molina et al., 2021; Kelley and Ardon-Dryer, 2021; Ardon-Dryer et al., 2023b; Albuquerque-Bernalillo County, 2024). In Sunland Park, New Mexico, Li et al. (2005) found during dust events that PM2.5 and PM10 hourly concentrations were 170 and 2346 µg m−3, respectively, while daily averages were 12 ± 8 and 69 ± 72 µg m−3, respectively.
Kelley et al. (2020) analyzed PM2.5 concentrations in Lubbock, Texas, over 17 years (2001–2018) and found that the majority of the hourly PM2.5 concentrations were lower than 10 µg m−3 (80 %), but there were several days with high PM including 15 April 2003 and 15 December 2003, which had PM2.5 hourly values of 433 and 486 µg m−3, respectively. Rivera Rivera et al. (2009) also examined the impact of these two dust storms in El Paso and found, on 15 April 2003, hourly PM10 concentrations of 4724 µg m−3 with a daily PM10 concentration of 375 µg m−3, while the hourly PM10 concentrations on 15 December 2003 were >1200 µg m−3. Daily PM10 concentrations on 15 December 2003, for another site in Texas, was >160 µg m−3 (Tong et al., 2012). Yin et al. (2005) examined hourly PM2.5 and PM10 measurements from different stations across New Mexico and Texas during the same dust storm (15 December 2003). They found hourly PM10 concentrations in New Mexico >700 µg m−3, while PM2.5 hourly concentrations ranged from 12 to 36 µg m−3 (Yin et al., 2005). Both of these dust storms were caused by synoptic disturbances. In Lubbock, Texas, it was found that PM2.5 daily concentrations during synoptic dust events had slightly higher average levels compared to those during convective dust events. Ardon-Dryer and Kelley (2022) also found that synoptic dust events had higher PM2.5 and PM10 daily concentrations compared to convective dust events, but short-term observation (based on 10 min) showed that convective events had much higher PM concentrations. The impact of PM2.5 PM10 and PM10–PM2.5 values during dust events in the region were examined but only by a handful of studies. In New Mexico, PM2.5 PM10 values ranged from 0.05 to 0.58, and the PM2.5 PM10 ratio was extremely low (0.079 to 0.093) during dust events (Li et al., 2005). Measurements of daily PM2.5 and PM10 using multiple Interagency Monitoring of Protected Visual Environments (IMPROVE) stations in New Mexico and Texas also found a significant drop in the PM2.5 PM10 ratio during dust events, with daily means that ranged from 0.22 to 0.24 (Tong et al., 2012).
The dust storm of 26 February 2023 is one the strongest dust storms that has occurred in this region over the last 2 decades. This study aims to understand the meteorological conditions that initiated this dust storm and those measured during it using multiple meteorological stations across New Mexico and Texas, capturing the storm's spatial and temporal changes. The impact this dust storm had on air quality over the two states was of interest in understanding whether it had an impact on PM concentrations in the region and how significant this impact was and in evaluating its similarity to previous dust events in this region.
2.1 Automatic surface observation systems (ASOSs)
Automatic surface observation systems (ASOSs) are meteorological stations located at most airports across the United States that provide meteorological measurements released to the public via meteorological aerodrome reports (METARs). The meteorological measurements include 5 min to 1 h measurements of temperature, dew point, relative humidity, wind speed, wind direction, wind gust, pressure, visibility, and precipitation as well as “present weather code”. Some stations are continuously monitored by a contracted weather observer, while others are mostly automatic (Ardon-Dryer et al., 2023b). The purpose of the weather observer is to back up any instrumentation outages and augment any weather information that the ASOS station cannot automatically record. The present weather code (entered automatically by the station or manually by the on-duty observer) is an important aspect of METARs as it provides information on the current weather, such as thunderstorms, fog, hail, and dust events. The classification of the dust event in this study was based on the combination of present weather codes such as BLDU (blowing dust), VCBLDU (vicinity blowing dust), DU (widespread dust), DS (dust storm), and HZ (haze), with a reduction in horizontal visibility (<10 km) and increase in wind speed (>6 m s−1) but without precipitation; this classification is similar to the method used in Ardon-Dryer et al. (2023b) and Robinson and Ardon-Dryer (2024). The different present weather codes for dust are defined by the World Meteorological Organization (WMO) and the Federal Aviation Administration (FAA). BLDU represents a case when the dust is present in the atmosphere and visibility drops below 11 km, DU indicates that dust is present and gives distant objects a tan or gray tinge, DS represents dust reducing the visibility to 1 km or less, and VCBLDU refers to dust being present within 8 to 16 km of the station. Each of these codes can only be entered manually by a weather observer (WMO, 2019; FAA, 2021). It should be noted that 16.1 km is the maximum visibility that should be reported by the ASOS station (ASOS User's Guide, 1998). Many studies have used the present weather codes to identify dust events in this region (Kandakji et al., 2020; Herrera-Molina et al., 2021; Kelley and Ardon-Dryer, 2021; Robinson and Ardon-Dryer, 2024). METAR data from 28 ASOS stations across West Texas and New Mexico were downloaded from the Iowa University Mesonet (Iowa Mesonet, 2023) for February 2023. Table S1 in the Supplement provides information on each of the ASOS stations utilized in this study, while Fig. 1a shows their location. Additional ASOS stations (13 in total) from the region could not be used, since they either had missing measurements or did not have dust conditions on 26 February. It should be noted that there are some limitations to the use of ASOS stations, as there were only four stations with full-time weather observed, while the remaining were semi-/fully automated. In a recent study (Robinson and Ardon-Dryer, 2024), it was found that there could be mistakes in dust identifications. To make sure such will not happen, this study follows the guidance provided by Robinson and Ardon-Dryer (2024) to remove such cases. Another related issue is the fact that the automated stations can stop operating due to outages and in some cases, cannot be backed up, which has happened to some of the stations in this study limiting the ability to use the data. Regardless of these limitations, the usage of the ASOSs with the spatial and temporal coverage allowed examination into the development and movement of the dust event.
2.2 Particulate matter
Hourly concentrations for PM10 and PM2.5 from six stations across West Texas were taken from the Texas Commission on Environmental Quality (TCEQ, 2023), while hourly PM10 and PM2.5 concentrations for 13 stations across New Mexico were taken from the New Mexico Environment Department (2023) or provided by Patrick Hudson, a senior environment health scientist for the City of Albuquerque. All of the PM sensors employ federal equivalent methods (FEMs). Each FEM instrument had a different resolution depending on the operated unit – some units ranged from 0.1 to 10 000 µg m−3 (T640, 2024) or from −15 to 10 000 µg m−3 (BAM 1022, 2024), while others had an upper limit of 5000 µg m−3 (EPA, 2024); see Table S2 in the Supplement for information on the instrument used at each location. The PM data included hourly measurements for February 2023. Calculations of the daily average were made for each day based on hourly measurements from midnight to 23:00 local time (LT). Table S2 in the Supplement outlines each of the PM sensors used in this study, while Fig. 1b shows the geographical spread of PM sensors. Six stations only measured PM2.5 (5ZS, 6Q, C320, C1028, C1014, and C37) concentrations, and four only measured PM10 (6ZK, 6ZL, 6ZM, and 6WM) concentrations, while the remaining nine stations had both PM2.5 and PM10 (6CM, Del Norte HS, Foothills, Jefferson, North Valley, San Jose, South Valley, C49, and C41). The majority of the stations across West Texas contain only PM2.5 measurements, while other locations including El Paso, Texas, and Albuquerque, New Mexico, contain both PM2.5 and PM10. Calculations of PM10–PM2.5 and PM2.5 PM10 were performed for stations that contained both PM2.5 and PM10. Almost all PM sensors had meteorological measurements such as hourly ambient temperature, wind direction, and wind speed. If these variables were not available, meteorological measurements from the nearest ASOS or PM station were used. For example, station 6Q in Las Cruces, New Mexico, did not have wind measurements, and therefore wind measurements from the closest station (6WM in West Mesa, which is 10.6 km away) were used to supplement the missing data. Additionally, station C320 in Amarillo, Texas, did not have wind measurements, and therefore wind measurements were taken from the Amarillo (AMA) ASOS station (11.8 km away). Lastly, the San Jose and North Valley (in Albuquerque, New Mexico) stations did not have wind measurements, and therefore wind measurements from the South Valley station (5.7 and 5.2 km away, respectively) were used. It should be noted (as shown in Fig. 1b) that there is a wide spatial gap between the PM sensors, as these are the only active sensors in the area. Also, most of the PM sensors in Texas (except those in El Paso) only provide PM2.5, meaning the impact of PM10 in West Texas will not be provided in this study.
2.3 Meteorology overview maps
The synoptic maps were made using the North American Rapid Refresh version 3 (RAPv3) with horizontal grid spacing of 13 km and 51 vertical levels (Benjamin et al., 2016). RAPv3 was selected to illustrate the meteorology due to its 1 h assimilation frequency and ability to provide one of the best forecasts in the rapidly changing atmosphere. Only the initialization hours were used in this study. Each synoptic map was made using the MetPy Python package (May et al., 2022), with several meteorological variables layered. The following variables were chosen to analyze the meteorology: geopotential heights (mid-level and surface), wind speed and direction (mid-level and surface), temperatures (mid-level), and dew point temperatures (surface).
The Geostationary Operational Environmental Satellites (GOES) imagery over eastern North America (GOES-East, also known as GOES-16) encompasses the research area and is updated every 5 min. The satellite data were pulled from Amazon Web Services and plotted with the GOES-2-Go Python package (Blaylock, 2022). The Dust RGB satellite product was utilized to highlight the progression of the dust throughout the dust event. The Dust RGB product, which consists of band differencing and an IR thermal channel, allows dust to be observed through satellite imagery during both the day and the night. The density of the dust particles was inferred by the range of the magenta-pink color. This method is commonly used to detect and identify dust events (Fuell et al., 2016; Ardon-Dryer et al., 2023b).
NEXRAD WSR-88D radars were also used to visualize thunderstorms and mesoscale boundaries. The data from various radars across the southern Great Plains (Lubbock, Midland–Odessa, Amarillo, Clovis, San Angelo, Dyess Air Force Base, and Frederick) were retrieved via Amazon Web Services to plot a mosaic radar image using a Py-ART Python package (Helmus and Collis, 2016).
3.1 The meteorological conditions resulted in the formation of the dust storm
During the morning and afternoon hours on 26 February 2023, a robust and slightly negatively tilted 500 mbar closed low was ejected eastward from southern California across the Four Corners region of the United States (Fig. 2a). The right exit region (Fig. 2b) of the nearly 51–62 m s−1 (100–120 kn) 500 mbar jet streak, associated with the upper low, entered the Chihuahuan Desert region of Mexico, Texas, and New Mexico around early afternoon to mid-afternoon. A stacked 700 mbar trough axis brought a 31–36 m s−1 (60–70 kn) jet axis over the area at the same time (data not shown). In conjunction with the approaching upper low, intense surface cyclogenesis developed along the leeward side of the Rocky Mountains before sliding eastward across the Oklahoma Panhandle and then pushing farther northeast into Kansas by midnight. Warm air advection from southerly surface winds led to sufficient daytime heating and mixing of the strong winds aloft to the surface. In addition, the south winds advected low-level moisture into the southern Great Plains with a weak dry line present and near 40 to 50° dew points east of the boundary (Fig. 2c). Instability in the atmosphere combined with this boundary led to thunderstorm development across the far southern Texas Panhandle through the afternoon hours (starting around 17:00 LT/23:00 UTC). As the upper-level closed low continued to swing eastward through the evening, so did the corresponding north–south-extending Pacific cold front. The front eventually caught up with the dry line just east of Lubbock, where additional storms were initiated along the colliding boundaries as shown in the radar reflectivity (Fig. 3a), along with both boundaries (dry line and Pacific cold front) at 17:00 central time for Texas (23:00 UTC). These thunderstorms created very strong wind gusts of up to 51 m s−1 across West Texas (shown in Fig. S1 in the Supplement).
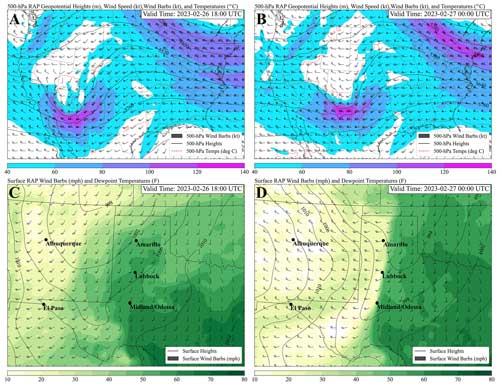
Figure 2The 500 mbar geopotential heights (m), wind speed (kn, denoted “kt” in the figure, shaded; 1 kn is 0.514 m s−1), wind barbs (kn, denoted “kt” in the figure), and temperature (°C) for 26 February 2023 at 18:00 UTC, 12:00 central time, when the dust event started (a) and for 27 February 2023 at 00:00 UTC, 18:00 central time, when the dust event intensified and turned into a dust storm across West Texas (b) and surface wind barbs (mph; 1 mph is 1.609 km h−1) and dew point temperature (°C, shaded) for 26 February at 18:00 UTC, 12:00 central time (c), and for 27 February 2023 at 00:00 UTC, 18:00 central time (d).
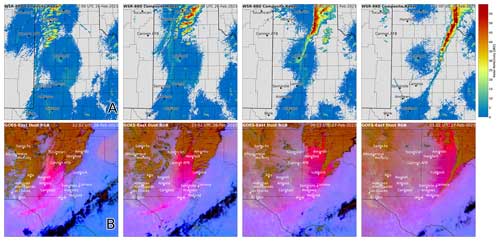
Figure 3Radar imagery (a) and GOES-East Dust RGB imagery (b) of the dust storm from 26–27 February at four different times (16:00, 17:00, 18:00, and 19:00 central local time/22:00, 23:00, 00:00, and 01:00 UTC, respectively).
The south-southwest winds began to increase through the morning hours and into the afternoon, with several severe wind gusts (>26 m s−1) reported across eastern New Mexico and West Texas. Multiple National Weather Service (NWS) offices across the southern High Plains and southern Great Plains highlighted the wind potential through products such as high-wind warnings and wind advisories. As the south-southwest surface winds increased, dust particles began to be lofted and started to cause a reduction in visibility. When the Pacific front began to move eastward across New Mexico, winds began to shift out of the west and continued to exhibit strong to severe wind speeds. Additional dust particles were suspended along the quickly moving boundary. Both radar reflectivity and satellite imagery (Fig. 3) reveal the evolution and intensity of the dust along this front during the afternoon hours across West Texas. One of the biggest factors that made this event an anomaly was the already-advected dust across a large area ( km2) prior to the frontal passage due to the strong to severe winds from the south-southwest during the morning hours, as shown in Fig. 3b. Some of the locations mentioned throughout this study (e.g., Lubbock, Texas) might experience both synoptic and convective disturbances, which may assist in the duration of high wind gusts along with the severity of dust in the region. The fact that some of the locations had both synoptic and convective disturbances (also known as combinations) is a rare aspect of this region, as only a handful of the dust events were caused by such conditions. For Lubbock, Texas, ∼15 % of the past DSs (2000–2021) were caused by a combination of disturbances (Robinson and Ardon-Dryer, 2024).
3.2 Meteorological observations during the dust storm
Observations of wind speed, wind gust, and visibility were collected from each of the 28 active ASOS units. ASOS units with on-duty observers (such as LBB for Lubbock, ELP for El Paso, and ABQ for Albuquerque) had present weather codes that represent dust (e.g., BLDU; VCBLDU; DS; and SS, sand storm), while the remaining automated ASOS units had a present weather code of haze (HZ). Some of the stations reported a dust weather code before the visibility was reduced (<10 km), but since this work follows the WMO (2019) dust event definition, these times were not included. The first observation of dust was when the visibility was reduced below 10 km, and the end of the dust event was when visibility first exceeded 10 km. Observations of visibility showed that the dust event started in New Mexico during the morning hours and was first observed in Texas around noon local time. The first report of dust across New Mexico in the present weather code was in Deming (DMN) at 10:00 LT, with HZ reported and visibility dropping below 10 km to 6.4 km, making it officially a dust event based on the World Meteorological Organization (WMO, 2019). At 10:21 LT in Albuquerque (ABQ), visibility was reduced to <10 km. In Texas, the first observations of dust were in El Paso (ELP) at 11:10 LT, when the visibility dropped to 8.0 km. The dust moved northeast and reached the West Texas region about an hour later. The last station that observed dust was Midland–Odessa (MDD). Despite the strong winds that started in the morning hours, the first reduction in visibility only happened at 18:35 LT. All three Midland–Odessa stations experienced the dust at a later time when the Pacific cold front and dry line moved across the area. The last report of dust was on 27 February at 04:55 LT at the LLN station in Levelland, Texas. The ASOS stations LBB and LUV, which are near LLN, also had reports of dust until after 03:00 LT. The presence of dust at some of the stations (based on duration with visibility <10 km) varies. On average the dust across all stations lasted for 5 h 30 min ± 3 h 30 min since some stations experienced dust for short durations, while others did so for long durations. The station with the shortest duration was MDD, where the dust lasted only 80 min. For the ASOS in New Mexico, the average duration of dust was 4 h 7 min, ranging from 1 h 40 min (0E0) to 6 h 27 min (HNM). In Texas, on average the reported dust was 6 h 42 min, with the shortest duration in MDD and the longest duration of 15 h 20 min in LUV.
The duration of the dust events on 26 February varied between stations over many hours from 1 h 20 min to 15 h 20 min, although overall this dust event lasted longer than previous dust events reported in the area (Doggett et al., 2002; Kelley and Ardon-Dryer, 2021). Shorter dust events were also reported in Arizona (Nickling and Brazel, 1984; Raman et al., 2014; Eagar et al., 2017) and in other locations around the world such as China (Wang et al., 2005; Guan et al., 2015) and Turkmenistan (Orlovsky et al., 2005). However, some of the durations reported by other studies were similar to those found in this study. For example, Novlan et al. (2007) found for El Paso that most dust events lasted on average 3–4 h, and the longest lasted 24 h. Robinson and Ardon-Dryer (2024) examined hundreds of dust events across West Texas and found that most of the dust events lasted less than 5 h, which is around the average duration found in this study. Although many of the dust events lasted less than 1 h, the longest dust event reported was even longer than the one reported here. Similar duration times have been reported in other locations; for example, in Mongolia, dust events last on average 2–6 h (Natsagdorj et al., 2003). However, long-lasting dust events (2 d and more) have been reported in other locations around the world such as in the Middle East (Birinci et al., 2023) and Europe (Sorribas et al., 2017).
The wind speed measured at the beginning of the dust event (based on the first visibility observation <10 km) ranged from 12 m s−1 (MAF) to 26 m s−1 (SRR at Alto, New Mexico) with wind gusts measured from 16 m s−1 (MAF) to 33.4 m s−1 (SRR at Alto, New Mexico). The highest wind speed and wind gust (27 and 37 m s−1, respectively) recorded during the dust event were in New Mexico (TCC at Tucumcari). Calculation of the average wind speed and wind gust for the duration of the dust event was performed for each station. The average wind speed during the duration of the dust events across all the ASOS stations was 17 ± 2 m s−1; these values vary from 12 ± 3 m s−1 to 22 ± 2 m s−1. The average wind gust reported across all the ASOS stations at the time of the dust was 23 ± 3 m s−1; these values vary from 17 ± 3 m s−1 to 29 ± 2 m s−1. Information from each ASOS station including the duration and the maximum wind speed and wind gust at the beginning of and during the dust event can be found in Table S3 in the Supplement. These wind speeds and wind gusts measured during the dust events were 3.2 times higher than the average wind speed and wind gust recorded in the month of February 2023 (shown in Table S3). The difference was much stronger for the strongest-recorded wind speed and wind gust, up to 5.9 times and 8.3 times higher, respectively, compared to the month of February. These big differences indicate how strong this dust event was. But looking at the overall meteorological conditions during this month, it seems that there were additional dust events during that month (e.g., 9 and 22 February), but they were not as strong as the one reported here (data not shown). Perhaps if these dust times had been removed from the monthly analysis, the differences among the meteorological conditions would have been stronger.
Most of the wind speeds at the beginning of the dust event were above the wind speed reported by Stout and Arimoto (2010) as a threshold for dust to be suspended. The wind speeds during this event were in the range of wind speeds reported in Hagen and Woodruff (1973) for dust events that occurred in the Great Plains in the 1950s. All ASOS locations examined showed wind speed values at the beginning of the dust event that were higher than the wind speed reported by Zobeck and Van Pelt (2006) during the March 2003 dust storms in the region. Similar maximum wind speed values were measured in the area during the 15 December 2003 dust storm (Lee et al., 2009). However, much higher wind speeds were measured in the July 2014 dust storm in Phoenix, Arizona (Eagar et al., 2017), perhaps since the one in Arizona was convective.
The reduction in visibility during the dust event varied from station to station; the average visibility for the duration of the dust event was 5.4 ± 1.5 km, with these values varying from 3.2 ± 2.3 to 9.2 ± 5.4 km. The average of the lowest visibility reported during the dust event was 1.5 ± 1.0 km. Although all the ASOS units in the region experienced dust event conditions, not all experienced dust storm conditions (visibility <1 km), as shown in Table S3. A total of 11 ASOS stations (6 in New Mexico and 5 in Texas) reported visibility below 1 km and therefore experienced dust storm conditions. The remaining stations had a reduction in visibility but not below 1 km (shown in Table S3). The visibility values of stations that experienced dust storm conditions ranged from 0.8 down to 0 km, as shown in Fig. 4. The duration of the dust storm conditions ranged from 5 min (INK) to 2 h (LLN). The ASOS station in Lubbock, Texas (LBB), reported 0 km visibility for a continuous 13 min, which highlights the severity of this dust storm. It should be noted that several of the West Texas stations that experienced DS conditions (including LBB, LLN, LUV, and INK) had fog conditions in the morning hours of this dust event day, which could explain the low visibility values in the morning hours (Fig. 4). Almost all stations showed a peak wind speed and wind gust at the time of minimum visibility, as shown in Fig. 4. The western stations (New Mexico) experienced dust storm conditions at around noon LT when wind speeds reached their maximum. Meanwhile, the eastern stations (mainly those in West Texas) experienced dust storm conditions in the late afternoon when the front collided with the dry line. Satellite observations from the GOES-East Dust RGB product (shown in Fig. 3b) highlight the high concentrations of dust particles during these times. Satellite observations from GOES-East showed that the dust particles from this dust storm made it to Oklahoma, Kansas, and Arkansas (data not shown). Previous studies also found that dust particles from this region can travel to neighboring states including Oklahoma (Park et al., 2007; Kandakji et al., 2020) and as far as the northeastern states, even into Canada (Doggett et al., 2002; Park et al., 2007) depending on the synoptic setup.
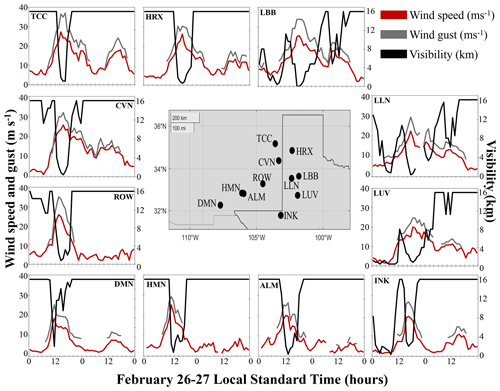
Figure 4Observations of wind speed (red) and wind gust (gray) as well as visibility (black) from ASOS stations that experienced dust storm conditions (visibility <1 km).
This region of New Mexico and West Texas is prone to dust events (Park et al., 2009; Kandakji et al., 2020; Kelley and Ardon-Dryer, 2021) due to the proximity to the Chihuahuan Desert and many agriculture fields (Rivera Rivera et al., 2009; Lee et al., 2012). Studies found that many of the dust events in this region occur during December–May, particularly in the springtime months (Stout, 2001; Novlan et al., 2007; Rivera Rivera et al., 2009). Severe dust storms have been observed in the past in this region (Lee and Tchakerian, 1995; Lee et al., 2009). Lee et al. (2009) analyzed the 15 December 2003 dust storm that started in New Mexico and moved eastward through West Texas. The dust storm was caused by an upper low-pressure system that brought in a cold front. During this dust storm, wind gusts in Lubbock, Texas, were over 28 m s−1 and visibility was reduced to 0.4 km. In El Paso, Texas, the minimum visibility reported was 2.8 km and wind gusts reached 23 m s−1 (Lee et al., 2009). The DS conditions during the event presented in this work were more severe compared to the DS on 15 December 2003, presented in Lee et al. (2009). The conditions of the current DS were also stronger (higher maximum wind gust and lower visibility values) than those reported in El Paso during the 15 April 2003 dust storm (Park et al., 2009; Rivera Rivera et al., 2009). Strong dust storms with similar meteorological conditions have been observed in other locations across the United States, including Arizona (Raman et al., 2014; Kim et al., 2017) and Utah (Nicoll et al., 2020). In Utah, Nicoll et al. (2020) examined a dust storm that occurred on 14–15 April 2015. An intense intermountain cyclone caused high wind gusts (up to 35 m s−1) and dust storm conditions, with visibility down to 0.4 km. In Arizona, dust storms are common (Lei et al., 2016; Ardon-Dryer et al., 2023b). One of the largest and most famous dust storms recorded in Arizona (near Phoenix and Tucson) occurred on 5 July 2011 (Raman et al., 2014; Vukovic et al., 2014; Lader et al., 2016). This dust storm developed due to thunderstorms (Lader et al., 2016) and had a peak wind gust of 29 m s−1 and visibility of 0 km (Raman et al., 2014; Vukovic et al., 2014), similar to the conditions presented in this work.
3.3 Impact of dust storm on PM concentrations and air quality
Nineteen air quality stations were active across the New Mexico and West Texas region during the dust event presented in this study. Each of the PM stations showed an increase in PM values during the dust event, but not all PM stations were strongly impacted by the dust event, as indicated by the varying hourly PM measurements across the region (Fig. 5). The maximum PM values at the peak of the dust for each station ranged from 14 µg m−3 (Foothills station in Albuquerque, New Mexico) to 518 µg m−3 (station C1028 in Lubbock, Texas) for PM2.5 and from 198 µg m−3 (Foothills station in Albuquerque, New Mexico) to 9983 µg m−3 (station 6ZM in Desert View, New Mexico) for PM10. Details for each station can be found in Table S4 in the Supplement. High PM concentrations during dust storms are common in this area (Ardon-Dryer et al., 2022a, b; Kelley and Ardon-Dryer, 2021), along with other locations across the United States (Hahnenberger and Nicoll, 2012; Lei et al., 2016; Achakulwisut et al., 2017) and around the world (Ardon-Dryer and Levin, 2014; Mamouri et al., 2016; Arhami et al., 2017; Milford et al., 2020).
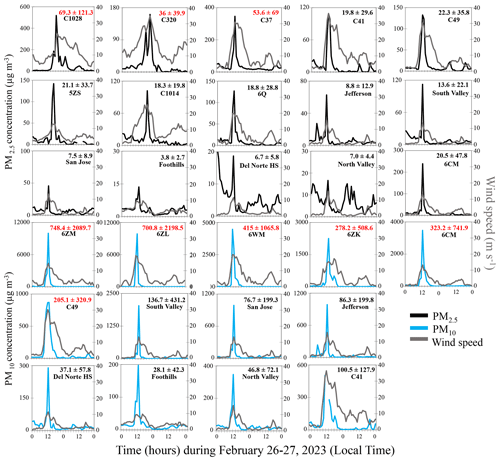
Figure 5Changes in PM2.5 (black) and PM10 (blue) with wind speeds (gray) measured during the dust storm. The name of the station and daily average ± SD values for 26 February are presented in black. Daily average ± SD values for stations that exceeded the EPA daily standards are presented in red.
The duration of dust particles being in the air was based on the time from the first increase in PM to the decrease in PM values. This duration was similar to the duration of the reduction in visibility, mentioned in Sect. 3.2. These durations based on PM values varied; some stations had an increase in PM values for a duration of 2 h, while others had an increase for up to 12 h. Despite the reduced visibility to 1.6 km during the dust event at the Albuquerque ASOS (ABQ), most of the PM stations in the area witnessed a small increase in PM2.5 but a more significant increase in PM10 concentrations (as can be seen in Fig. 5 and Table S4). A spatial impact of the dust was also observed in Albuquerque, as stations in the southern part of Albuquerque had higher PM concentrations (with a stronger increase) compared to those located in the northern part of Albuquerque. When calculating the increased ratio of PM, which is indicated by the ratio of PM concentrations at the peak of the dust compared to the PM concentrations right before the dust, results showed an increase in PM across the region, even across Albuquerque. PM2.5 concentrations during the dust event were on average 12.8 times higher compared to the time before the dust event (ratios vary from 3.0 to 36.3), while PM10 concentrations during the dust event were on average 216.9 times higher compared to before the dust event (ratios vary from 11 to 1426). When we examined the same ratio aspect for the lowest PM concentrations recorded on 26 February (shown in Table S4), the differences were much higher; PM2.5 and PM10 concentrations at the peak of the dust were higher by more than an order of magnitude (on average) than the minimum daily PM concentration recorded on the same day.
Higher hourly PM2.5 concentrations for Lubbock, Texas (518 µg m−3 at peak of dust), were measured during this dust storm compared to those measured during the past dust storm of 15 December 2003, when PM2.5 concentrations reached 486 µg m−3 (Lee et al., 2009; Park et al., 2009). The hourly PM2.5 concentration measured in Lubbock was higher than any hourly PM2.5 concentration recorded over the last 20 years. In El Paso, Texas, during the same 15 December 2003 dust event, the hourly PM10 concentration was >1200 µg m−3 (Rivera Rivera et al., 2009), which was higher compared to the hourly PM10 concentration measured in El Paso (999 µg m−3 by station C41) during this dust storm. However, several New Mexico PM stations measured concentrations >9868 µg m−3 (PM stations 6ZM and 6ZL). Most of the maximum hourly PM10 concentrations in this study were higher compared to the hourly maximum PM10 concentration measured during three previous dust events in 2019 in Lubbock, Texas (Ardon-Dryer and Kelley, 2022). Many of the hourly maximum PM2.5 concentrations measured by Ardon-Dryer and Kelley (2022) were higher than the hourly PM2.5 concentrations presented in this study. Observations of hourly PM2.5 and PM10 concentrations in this study were in a similar range to PM concentrations for other dust storms measured across the United States. For example, Nicoll et al. (2020) examined a dust storm that occurred across the Great Basin region of Utah in April 2015, where PM2.5 and PM10 hourly concentrations reached 298 and 890 µg m−3, respectively. Measurements of PM concentrations during various dust storms in Arizona also showed similar values in comparison to the results of this dust event (Raman et al., 2014; Kim et al., 2017). There were some dust events in Arizona with much higher PM hourly concentrations (Eagar et al., 2017; Hyde et al., 2018) compared to this study. For example, Raman et al. (2014) reported high PM values in Phoenix, Arizona, during a convective dust event, with hourly maximum PM2.5 and PM10 concentrations of 907 and 1974 µg m−3, respectively. These different PM values could be attributed to the differences in regions, along with the cause of the dust event.
Observations of the changes in PM concentrations and wind speed as shown in Fig. 5 indicate a relationship between the two variables. Some studies found a correlation between wind speed and PM concentrations (Karami et al., 2017; Kim et al., 2017), while others could not find a strong relationship between the two (Kelley and Ardon-Dryer, 2021). Calculations of regression (linear and polynomial) were made based on hourly PM concentrations and wind speeds from the PM stations with measurements from 26 February, and they showed a low linear correlation for most of the stations (Table S4). For stations that measured PM2.5 concentrations, R2 values for linear regression were not significant and ranged from 0.01 (North Valley station in Albuquerque, New Mexico) to 0.47 (station 5ZS in Hobbs, New Mexico, and station C49 in El Paso, Texas). For stations that measure PM10 concentrations, R2 values ranged from 0.3 (Jefferson station at Albuquerque, New Mexico) to 0.6 (station C49 in El Paso, Texas). Only three PM10 stations (station C49 in El Paso, Texas; station 6CM in Anthony, New Mexico; and North Valley station in Albuquerque, New Mexico) had high linear correlation values (R2≥0.5). Other regression models were also examined to potentially find a better regression value between wind speed and PM values. The polynomial regression (with a second-degree polynomial) presented much higher R2 values compared to those of a linear regression, with R2 values that ranged from 0.37 to 0.9 for PM2.5 and from 0.18 to 0.9 for PM10. Of the PM2.5 and PM10 stations, 73.3 % and 84.6 %, respectively, had R2≥0.5 (see R2 values in Table S4).
The calculations of PM concentrations during the time of dust were performed for each station. The PM concentrations during the time of dust, which varied per station, were on average 70 ± 50 µg m−3 for PM2.5 and 686 ± 689 µg m−3 for PM10. The PM2.5 concentrations during the time of dust ranged from 8.4 ± 4.0 µg m−3 in the Foothills station in Albuquerque, New Mexico, to 154 ± 135 µg m−3 at station C1028 in Lubbock, Texas. The PM10 concentrations during the time of dust ranged from 104 ± 65 µg m−3 at the Foothills station in Albuquerque, New Mexico, to 2354 ± 3745 µg m−3 at station 6ZL in Desert View, New Mexico (values for each sensor are shown in Table S4). The PM2.5 and PM10 concentrations during the time of dust were 2.0 and 3.3 times lower (for PM2.5 and PM10, respectively) compared to those measured at the peak of the dust. Daily values for each station (calculated for 26 February from midnight to 23:00 LT for each sensor) showed a wide range of values. PM2.5 daily concentrations ranged from 3.8 ± 2.7 µg m−3 (Foothills station in Albuquerque, New Mexico) to 69 ± 121 µg m−3 (station C1028 in Lubbock, Texas), while PM10 daily concentrations ranged from 28 ± 42 µg m−3 (Foothills station in Albuquerque, New Mexico) to 748 ± 2090 µg m−3 (station 6ZM in Desert View, New Mexico). On average the PM concentrations during the time of the dust were 3.2 times higher compared to the daily concentrations (for both PM2.5 and PM10); the difference ranged from 1.4 to 5.7 for PM2.5 and from 2.0 to 7.6 for PM10, as shown in Table S4. The concentrations at the peak of the dust were also higher compared to daily values. The peak of the dust had concentrations 6.1 and 10.5 times higher (average for PM2.5 and PM10, respectively) compared to the daily average, and the difference ranged from 2.4 (for North Valley) to ∼12 (for 6CM) for PM2.5 and from 4.0 (for North Valley) to 21 (for San Jose) for PM10. Similar differences of higher PM concentrations at the peak of dust compared to daily values are shown in Hahnenberger and Nicoll (2012) for Utah dust storms, where the daily concentrations were 3.8 and 12 times lower (for PM2.5 and PM10, respectively) compared to those measured at the peak of the dust.
To examine the impact of the 26 February 2023 dust event on the overall PM concentrations, daily PM concentrations were calculated for each PM sensor for each day during February 2023 (shown in Fig. S2 in the Supplement). The daily average for 26 February seems high (for most sensors) compared to the other February days' daily averages, it also seems to have much higher SD values compared to many of the other days. The lowest impact seems to be in the Albuquerque stations, perhaps since the area is also impacted by anthropogenic pollution. The southern part of New Mexico and many of the stations in West Texas seem to have experienced a bigger impact because of this dust event, as daily values for the dust day (26 February) were on average 12 times higher compared to the overall daily PM2.5 concentrations and 28 times higher compared to the PM10 daily concentrations. These differences could have been higher, but it seems there were additional pollution events (other dust events, as indicated above) in some of the locations, which increased the daily PM concentration for some days in some of the stations. Observations of daily PM2.5 concentrations from the different Albuquerque PM stations show that the dust was not as strong as it was for other locations such as southern New Mexico and West Texas. Next, the monthly PM2.5 and PM10 concentrations for the entire month of February were calculated (for each sensor), without 26 February PM concentrations (Table S4). The monthly PM2.5 and PM10 concentrations were on average 4.0 and 9.3 times lower, respectively, compared to the daily concentrations measured on 26 February. The monthly PM concentrations were 14 and 27 times lower compared to the PM2.5 and PM10 concentrations (respectively) measured during the time of the dust and 26 and 105 times lower (for PM2.5 and PM10 concentrations, respectively) compared to the PM concentrations at the peak of the dust. These large differences between the concentrations of PM2.5 and PM10 during the dust and those over the month indicate that while the background PM across the region might be low (except for Albuquerque), dust events in this region can have a significant impact on both PM2.5 and PM10 concentrations in the region which will impact the air quality and people's health.
Since EPA and WHO refer to air quality levels based on daily values, the daily concentrations for both PM2.5 and PM10 on 26 February were examined and compared to the EPA and WHO daily threshold. Only nine PM stations (three PM2.5 and six PM10) exceeded the EPA daily thresholds (35 µg m−3 for PM2.5 and 150 µg m−3 for PM10), indicated by the red daily average values in Fig. 5. Five of them were in southern New Mexico (6ZK, 6ZM, 6ZL, 6CM, and 6WM), and the remaining four were in West Texas (Lubbock, Amarillo, and two in El Paso). The stations where PM10 daily values exceeded the EPA daily threshold ranged from 205 ± 321 µg m−3 (station C49 in El Paso, Texas) to 748 ± 2090 µg m−3 (station 6ZM in Desert View, New Mexico). PM2.5 daily values for stations that exceeded the EPA daily values ranged from 36 ± 40 µg m−3 (station C320 at Amarillo, Texas) to 69 ± 121 µg m−3 (station C1028 at Lubbock, Texas). Analysis based on the new WHO thresholds for PM2.5 (daily values of 15 µg m−3) and PM10 (daily values of 45 µg m−3) showed that 9 of the PM2.5 stations (60 %) and 11 of the PM10 stations (85 %) were above the WHO thresholds, meaning these locations experienced a low air quality level. Many studies use daily averages to represent the PM concentration during dust events (Tong et al., 2012; Ardon-Dryer and Levin, 2014; Achilleos et al., 2016; Reynolds et al., 2016; Milford et al., 2020), which makes sense when the dust lasts for many hours or even longer than a day (Krasnov et al., 2016; Sugimoto et al., 2016). However, many of the dust events across the United States last for only a short period of a few hours or less (Claiborn et al., 2000; Kelley et al., 2020; Joshi, 2021; Robinson and Ardon-Dryer, 2024). As shown in this analysis and suggested in Ardon-Dryer et al. (2023a), the daily values underestimate and mask the concentration of both PM2.5 and PM10 concentrations. It is important to have hourly concentration measurements, as studies during dust events from this region (El Paso and Lubbock) have shown that maximum daily PM concentrations can lead to significant increases in hospitalizations on the day of dust and the following days. Different health impacts observed were related to respiratory diseases, asthma, mental health illnesses, stroke, and many other diseases (Herrera-Molina et al., 2021, 2024).
Next, the impact of this dust event on the presence of coarse particles (PM10–PM2.5) and the ratio between PM2.5 PM10 was examined. Across the study area, there were only nine stations that measured both PM2.5 and PM10. The majority (six) were in Albuquerque, while the remaining three were around the El Paso area. Calculations of PM2.5 PM10 and PM10–PM2.5 were made for each sensor for every hour. High PM10–PM2.5 values indicate the presence of coarse dust particles in the air. In Albuquerque, coarse particle concentrations were present in the air for 3–5 h, with concentrations that ranged from 37 to 2031 µg m−3. The southern part of Albuquerque was more strongly impacted by the dust storm as the stations (San Jose, Jefferson, and South Valley) showed higher PM10–PM2.5 values, with maximum values ranging from 923 to 2031 µg m−3 at the peak of the dust. In contrast, a small increase in PM2.5 and PM10 was found for the northern Albuquerque stations, indicating that dust was not that strong in that area. In the El Paso area, the PM10–PM2.5 values during the dust event had a slightly wider range from 53 to 3236 µg m−3. At the peak of the dust, PM10–PM2.5 values ranged from 871 to 3236 µg m−3 (sensor C41 is missing 2 h of data during the peak of the dust). The presence of coarse particles in the El Paso area (stations C41, C49, and 6CM) lasted for 8–10 h, as shown in Fig. 6. Daily PM10–PM2.5 values for 26 February were lower (from 3.8 to 7.3 times lower, average of 5.8 times lower) compared to the PM10–PM2.5 calculated during the time of dust (Table S5 in the Supplement). Also, PM10–PM2.5 at the peak of the dust was 16.6 times higher compared to the daily values. Calculations based on PM10–PM2.5 for each station for February showed that most stations had a low impact of coarse particles (except for San Jose and 6CM, which had higher monthly PM10–PM2.5 values, most likely due to the other dust events earlier that month). The PM10–PM2.5 values during the time of dust were higher (3.1 to 19 times higher, 12 times higher on average) than the PM10–PM2.5 monthly values. Even the daily PM10–PM2.5 averages for 26 February were higher (on average 2.2 times higher) than the monthly values. The hourly PM10–PM2.5 values from this study were higher, for most stations, compared to values measured in three different dust events in Lubbock, Texas, perhaps because this dust event was stronger (Ardon-Dryer and Kelley, 2022). The PM10–PM2.5 values were higher than those reported in the Rocky Mountains (Reynolds et al., 2016) and Utah (Hahnenberger and Nicoll, 2012). Similar ranges of PM10–PM2.5 values were measured during dust storms in Israel (Krasnov et al., 2016). The daily values here were lower compared to those measured in Israel, although the values at the peak of the dust were in the same range (Ardon-Dryer and Levin, 2014). However, the peak PM10–PM2.5 values were lower compared to the average PM10–PM2.5 values measured during multiple dust storms in China (Shao and Mao, 2016). Daily PM10–PM2.5 values in the dust event of the present study (for some of the stations) were in a range similar to that measured by Tong et al. (2012), who examined multiple dust events in the same area as the one in this study.
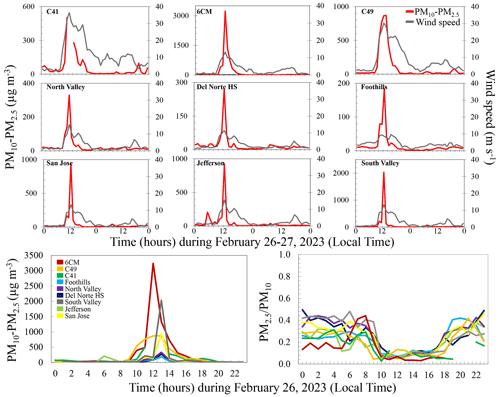
Figure 6Measurements of PM10–PM2.5 concentrations (in red) and wind speed (in gray) for each station. PM10–PM2.5 concentrations and the ratios of PM2.5 PM10 from stations; each color represents a different station.
Observations based on PM2.5 PM10 were also performed. The PM2.5 PM10 ratio is an important indicator used to characterize the underlying atmospheric processes within the local environment, which allows for the identification of the source of the particles (Yu and Wang, 2010). Higher PM2.5 PM10 ratios (>0.6) are generally associated with anthropogenic pollution, while lower ratios are associated with dust events (Jugder et al., 2014; Sugimoto et al., 2016; Jaafari et al., 2018; Fan et al., 2021; Ardon-Dryer et al., 2022b). PM2.5 PM10 values across the nine sensors decreased during the dust event mainly between 11:00 and 18:00 LT (Fig. 6). PM2.5 PM10 values across the nine stations ranged from 0.03 to 0.13, with an average of 0.07 ± 0.02 across all stations and times. These ratios were lower compared to the values reported by Tong et al. (2012), which were 0.22–0.24. Since Tong et al. (2012) PM2.5 PM10 values were based on daily values, calculations of daily values for each sensor were made (Table S5). The daily PM2.5 PM10 values (0.24–0.3) were in the same range as (and even slightly higher than) those in Tong et al. (2012). However, observations of these ratios during the time of dust (which were shorter in duration than the length of the day, as discussed above) were lower, with an average PM2.5 PM10 value of 0.07 (values across all stations ranged from 0.05 to 0.09). These values were similar to those measured by Li et al. (2005) during dust events in the El Paso region. The hourly PM2.5 PM10 values at the peak of the dust were lower compared to those measured at the peak in multiple dust storms in Utah (Hahnenberger and Nicoll, 2012; Nicoll et al., 2020). In Washington State, a similar range of daily PM2.5 PM10 values were measured (Claiborn et al., 2000). The daily PM2.5 PM10 values were in a similar range to those measured during dust events around the world (Alghamdi et al., 2015; Malaguti et al., 2018; Sugimoto et al., 2016; Jaafari et al., 2018).
Observations of the monthly PM2.5 PM10 values for February (without 26 February, shown in Table S5) ranged from 0.1 ± 0.08 (for 6CM) to 0.43 ± 0.24 (for C41). Most of the stations had lower monthly values compared to the daily PM2.5 PM10 values; some stations had similar values of ∼1. The 26 February daily PM2.5 PM10 values were on average 3.6 times lower than the monthly values, while the PM2.5 PM10 values at the peak of the dust were on average 6.2 times lower. The difference was slightly higher when monthly PM2.5 PM10 values were calculated without all the other suspected dust events (as mentioned in Sect. 3.2).
It seems that in some of the locations, the contribution of coarse particles was more crucial than those of fine particles, as shown by the low PM2.5 and high PM10 concentrations and by the high PM10–PM2.5 values and low PM2.5 PM10 ratios (at least for the stations that had measurements for both PM2.5 and PM10). However, several of the stations showed higher PM2.5 concentrations during the dust events, even values that were 5 times higher (as C1028, in Lubbock). Lubbock and many of the other locations only contain measurements of PM2.5, leading to speculation about whether the lower contribution for PM2.5 would be found across the region or just in the sites examined (the majority of them were urban sites). Additional studies are needed during dust events and dust storms across the region to provide measurements for both PM10 and PM2.5. Additional measurements of particle size distribution are important, as such information will give critical knowledge related to health impacts (because of inhalation of particles into the respiratory system), as well as to radiation and perhaps to cloud formation and precipitation processes.
On 26 February 2023, an upper-level, low-pressure system with a strong jet streak aided in the mixing of strong winds down to the surface, which resulted in the formation of a dust storm over portions of New Mexico and West Texas. The dust was first initiated in New Mexico during the morning hours and intensified as it moved eastward into West Texas. The average wind speed at the beginning of the dust storm was 15.6 m s−1, and during the dust storm wind speeds reached up to 26.2 m s−1 with wind gusts of up to 37 m s−1. Similar wind speeds were measured during different dust storms across the Great Plains, yet lower wind speeds were measured during several dust storms in Arizona. Visibilities ranged from 4 down to 0 km, meaning the event was defined as a dust storm (visibility <1 km). A total of 11 ASOS stations reported dust storm conditions for about 5 to 120 min, and the Lubbock ASOS reported zero visibility for 13 min. This dust storm had a big impact on the air quality in the area. Daily PM concentrations that exceeded the EPA daily threshold ranged from 36 ± 40 µg m−3 to 69 ± 121 µg m−3 for PM2.5 and from 205 ± 321 µg m−3 to 748 ± 2090 µg m−3 for PM10. Nine PM stations exceeded the EPA daily threshold. High hourly PM2.5 and PM10 concentrations during the dust storm reached a maximum of 518 and 9983 µg m−3, respectively. PM10–PM2.5 at the time of the dust, based on nine stations ranged from 96 ± 61 µg m−3 to 760 ± 1000 µg m−3, which is approximately 6 times higher than the daily PM10–PM2.5 values and 12 times higher than monthly PM10–PM2.5 values. PM2.5 PM10 during the dust time ranged from 0.05 ± 0.01 to 0.09 ± 0.03, which was 3.6 times lower than the daily and monthly PM2.5 PM10 values. The PM stations in the region, especially in West Texas, are spaced and far apart, meaning that higher PM concentrations than those measured could have occurred but were not reported. Dust particles were present in the air for approximately 16 h, impacting millions of citizens across eastern New Mexico and West Texas. In some locations (e.g., Lubbock), this dust storm was the strongest ever reported, as it had the highest PM2.5 concentrations recorded since the station became operational in 2001 and the lowest visibility recorded during a dust storm since 2003. Perhaps the meteorological disturbances that initiated the dust for Lubbock (synoptic with convective) led to these high PM concentrations. Additional studies across the region are needed to understand how meteorological disturbances that initiate dust events might impact the PM concentrations, as such information could be critical for prediction purposes to help alert the public. Such information could determine whether long-term effects such as land usage and climate change will affect the frequency and intensity of dust storms in this region.
Automatic surface observation system (ASOS) data are available from the Iowa Environmental Mesonet (https://www.mesonet.agron.iastate.edu/request/download.phtml?network=TX_ASOS, Iowa Mesonet, 2023). PM measurements for Texas were retrieved from the Texas Commission on Environmental Quality (https://www.tceq.texas.gov/cgi-bin/compliance/monops/monthly_summary.pl?cams=1028, TCEQ, 2023), while PM measurements from New Mexico were downloaded from the New Mexico Environment Department (https://aqi.air.env.nm.gov/, New Mexico Environmental Department, 2023). PM for Albuquerque was provided by Patrick Hudson, a senior environment health scientist for the City of Albuquerque's air quality program monitoring section. All measurements are available from the authors upon request.
The supplement related to this article is available online at: https://doi.org/10.5194/acp-24-13733-2024-supplement.
KS performed the dust event meteorological overview. MCR performed the analysis of the meteorological data from ASOS and the PM analysis. KAD designed the study and coordinated the different aspects of the paper. All authors were actively involved in interpreting the results and in discussions on the manuscript.
The contact author has declared that none of the authors has any competing interests.
Publisher's note: Copernicus Publications remains neutral with regard to jurisdictional claims made in the text, published maps, institutional affiliations, or any other geographical representation in this paper. While Copernicus Publications makes every effort to include appropriate place names, the final responsibility lies with the authors.
This research did not receive any specific grant from funding agencies in the public, commercial, or not-for-profit sectors. The authors would like to thank Texas Tech University for the support of Mary Robinson's scholarship and Patrick Hudson, a senior environment health scientist for the City of Albuquerque, for providing us with the Albuquerque PM2.5, PM10, and wind speed measurements.
This paper was edited by Barbara Ervens and reviewed by Allison C. Aiken and one anonymous referee.
Achakulwisut, P., Shen, L., and Mickley, L. J.: What controls springtime fine dust variability in the western United States? Investigating the 2002–2015 increase in fine dust in the U.S. Southwest, J. Geophys. Res., 122, 12449–12467, https://doi.org/10.1002/2017JD027208, 2017.
Achilleos, S., Wolfsona, M. J., Ferguson, S. T., Kanga, C. M., Hadjimitsis, D. G., Hadjicharalambous, G., Achilleos, C., Christodoulou, A., Nisanzti, A., Papoutsa, C., Themistocleous, K., Spyros, S., Perdikou, S., and Koutrakis, P.: Spatial variability of fine and coarse particle composition and sources in Cyprus, Atmos. Res., 169, 255–270, https://doi.org/10.1016/j.atmosres.2015.10.005, 2016.
Albuquerque-Bernalillo County: High Wind Fugitive Dust Mitigation Plan, https://www.cabq.gov/airquality/regulation-development/abq-bc-fugitive-dust-mitigation-plan-final-draft-1.pdf (last access: 25 July 2024), 2024.
Alghamdi, M. A., Almazroui, M., Shamy, M., Redal, M. A., Alkhalaf, A. K., Hussein, M. A., and Khoder, M. I.: Characterization and Elemental Composition of Atmospheric Aerosol Loads during Springtime Dust Storm in Western Saudi Arabia, Aerosol Air Qual. Res., 15, 440–453, https://doi.org/10.4209/aaqr.2014.06.0110, 2015.
Al Kheder, S. and Al Kandari, A.: The impact of dust on Kuwait International Airport operations: a case study, Int. J. Environ. Sci. Te., 17, 3467–3474, https://doi.org/10.1007/s13762-020-02710-3, 2020.
Arcusa, S. H., McKay, N. P., Carrillo, C. M., and Ault, T. R.: Dust-drought nexus in the southwestern United States: A proxy-model comparison approach, Paleoceanogr. Paleoclimatol., 35, e2020PA004046, https://doi.org/10.1029/2020PA004046, 2020.
Ardon-Dryer, K. and Kelley, M. C.: Particle size distribution and particulate matter concentrations during synoptic and convective dust events in West Texas, Atmos. Chem. Phys., 22, 9161–9173, https://doi.org/10.5194/acp-22-9161-2022, 2022.
Ardon-Dryer, K. and Levin, Z.: Ground-based measurements of immersion freezing in the eastern Mediterranean, Atmos. Chem. Phys., 14, 5217–5231, https://doi.org/10.5194/acp-14-5217-2014, 2014.
Ardon-Dryer, K., Chmielewski, V., Burning E., and Xueting X.: Changes of Electric Field, Aerosol, and Wind Covariance in Different Blowing Dust Days in West Texas, Aeolian Res., 54, 100762, https://doi.org/10.1016/j.aeolia.2021.100762, 2022a.
Ardon-Dryer, K., Kelley, M. C., Xueting, X., and Dryer, Y.: The Aerosol Research Observation Station (AEROS), Atmos. Meas. Tech., 15, 2345–2360, https://doi.org/10.5194/amt-15-2345-2022, 2022b.
Ardon-Dryer, K., Clifford, K. R., and Hand, J. L.: Dust under the radar: Rethinking how to evaluate the impacts of dust events on air quality in the United States, GeoHealth, 7, e2023GH000953, https://doi.org/10.1029/2023GH000953, 2023a.
Ardon-Dryer, K., Gill, T. E., and Tong, D. Q.: When a dust storm is not a dust storm: Reliability of dust records from the Storm Events Database and implications for geohealth applications, GeoHealth, 7, e2022GH000699, https://doi.org/10.1029/2022GH000699, 2023b.
Arhami, M., Hosseini, V., Shahne, M. Z., Bigdeli, M., Lai, A., and Shauer, J. J.: Seasonal trends, chemical speciation and source apportionment of fine PM in Tehran, Atmos. Environ., 153, 70–82, https://doi.org/10.1016/j.atmosenv.2016.12.046, 2017.
ASOS (Automatic Surface Observation System) User's Guide: https://apps.dtic.mil/sti/pdfs/ADA354716.pdf (last access: 11 January 2023), 1998.
Bach, A. J., Brazel, A. J., and Lancaster N.: Temporal and spatial aspects of blowing dust in the Mojave and Colorado deserts of Southern California, 1973–1994. Analytic Serial, Phys. Geogr., 17, 329–353, https://doi.org/10.1080/02723646.1996.10642589, 1996.
BAM 1022: Met One Instruments: BAM 1022 Beta Attenuation Mass Monitor, https://metone.com/products/bam-1022/, last access: 25 July 2024.
Blaylock, B. K.: GOES-2-go: Download and display GOES-East and GOES-west data, Github [code], https://github.com/blaylockbk/goes2go, 2022.
Benjamin, S. G., Weygandt, S. S., Brown, J. M., Hu, M., Alexander, C., Smirnova, T. G., Olson, J. B., James, E., Dowell, D. C., Grell, G. A., Lin, H., Peckham, S. E., Smith, T. L., Moninger, W. R., Kenyon, J. S., and Minikan, G. S.: A North American hourly assimilation and model forecast cycle: The rapid refresh, Mon. Weather Rev., 144, 1669–1694, https://doi.org/10.1175/MWR-D-15-0242.1, 2016.
Birinci, E., Özdemir, E. T., and Deniz, A.: An investigation of the effects of sand and dust storms in the North East Sahara Desert on Turkish airports and PM10 values: 7 and 8 April, 2013 events, Environ. Monit. Assess., 195, 708, https://doi.org/10.1007/s10661-023-11288-5, 2023.
Bogan, M., Al, B., Kul, S., Zengin, S., Oktay, M., Sabak, M., Gumusboga, H., and Bayram, H.: The effects of desert dust storms, air pollution, and temperature on morbidity due to spontaneous abortions and toxemia of pregnancy: 5 year analysis, Int. J. Biometeorol., 65, 1733–1739, https://doi.org/10.1007/s00484-021-02127-8, 2021.
Chen, L. W. A., Tropp, R. J., Li, W. W., Zhu, D., Chow, J. C., Watson, J. G., and Zielinska, B.: Aerosol and Air Toxics Exposure in El Paso, Texas: A Pilot Study, Aerosol Air Qual. Res., 12, 169–179, https://doi.org/10.4209/aaqr.2011.10.0169, 2012.
Claiborn, C. S., Finn, D., Larson, T. V., and Koenig, J. Q.: Windblown Dust Contributes to High PM2.5 Concentrations, J. Air Waste Manage., 50, 1440–1445, https://doi.org/10.1080/10473289.2000.10464179, 2000.
Craig, K., Erdakos, G., Chang, S. Y., and Baringer, L.: Air quality and source apportionment modeling of year 2017 ozone episodes in Albuquerque/Bernalillo County, New Mexico, J. Air Waste Manage., 70, 1101–1120, 2020.
Doggett IV, A. L., Gill, T. E., Peterson, R. E., Bory, A. J.-M., and Biscaye, P. E.: Meteorological characteristics of a severe wind and dust emission event, Southwestern, USA, 6–7 April 2001, 21st AMS Conference on Severe Local Storms, August, American Meteorological Society, Boston, MA, San Antonio, TX, 2002.
Eagar, J., Herckes, P., and Hartnett, H.: The characterization of haboobs and the deposition of dust in Tempe, Arizona from 2005 to 2014, Aeolian Res., 24, 81–91, https://doi.org/10.1016/j.aeolia.2016.11.004, 2017.
EPA: NAAQS Table, https://www.epa.gov/criteria-air-pollutants/naaqs-table, last access: 30 December 2023.
EPA: Sampling Methods for PM2.5 Speciation parameters, https://aqs.epa.gov/aqsweb/documents/codetables/methods_speciation.html, last access: 25 July 2024.
Evan, A. T.: Downslope Winds and Dust Storms in the Salton Basin, Mon. Weather Rev., 147, 2387–2402, https://doi.org/10.1175/MWR-D-18-0357.1, 2019.
FAA: Air traffic organization policy JO 7900.5E, https://www.faa.gov/documentLibrary/media/Order/Order_JO_7900.5E.pdf, last access: 10 May 2021.
Fan, H., Zhao, C., Yang, Y., and Yang, X.: Spatio-Temporal Variations of the PMPM10 Ratios and Its Application to Air Pollution Type Classification in China, Front. Environ. Sci., 9, 692440, https://doi.org/10.3389/fenvs.2021.692440, 2021.
Fuell, K. K., Guyer, B. J., Kann, D., Molthan, A. L., and Elmer, N.: Next generation satellite RGB dust imagery leads to operational changes at NWS Albuquerque, J. Operational Meteor., 4, 75–91, https://doi.org/10.15191/nwajom.2016.0406, 2016.
Gaffney, J. S., Marley, N. A., Martin, R. S., Dixon, R. W., Reyes, L. G., and Popp, C. J.: Potential Air Quality Effects of Using Ethanol–Gasoline Fuel Blends: A Field Study in Albuquerque, New Mexico, Environ. Sci. Technol., 31, 3053–3061, https://doi.org/10.1021/es9610388, 1997.
Gorris, M. E., Ardon-Dryer, K., Campuzano, A., Castañón-Olivares, L. R., Gill, T. E., Greene, A., Hung, C.-Y., Kaufeld KA., Lacy, M., and Sánchez-Paredes, E.: Advocating for coccidioidomycosis as a nationally reportable disease in the United States and encouraging disease surveillance across North and South America, J. Fungi, 9, 83, https://doi.org/10.3390/jof9010083, 2023.
Goudarzi, G., Daryanoosh, S. M., Gidini, H., Hopke, P. K., Sicard, P., De Marco, A., Rad, H. D., Harbizadeh, A., Jahedi, F., Mohammadi, M. J., Savari, J., Sadeghi, S., Kaabi, Z., and Omidi Khaniabadi, O.: Health risk assessment of exposure to the Middle-Eastern Dust storms in the Iranian megacity of Kermanshah, J. Public Health, 148, 109–116, https://doi.org/10.1016/j.puhe.2017.03.009, 2017.
Guan, Q., Yang, J., Zhao, S., Pan, B., Liu, C., Zhang, D., and Wu, T.: Climatological analysis of dust storms in the area surrounding the Tengger Desert during 1960–2007, Clim. Dynam., 45, 903–913, https://doi.org/10.1007/s00382-014-2321-3, 2015.
Hagen, L. J. and Woodruff, N. P.: Air Pollution from Dustorms in the Great Plains, Atmos. Environ., 7, 323–332, 1973.
Hahnenberger, M., and Nicoll, K.: Meteorological Characteristics of Dust Storm Events in the Eastern Great Basin of Utah, U.S.A., Atmos. Environ., 60, 601–612, https://doi.org/10.1016/j.atmosenv.2012.06.029, 2012.
Helmus, J. J. and Collis, S. M.: The Python ARM Radar Toolkit (Py-ART), a library for working with weather radar data in the Python programming language, United States, J. Open Res. Softw., 4, https://doi.org/10.5334/jors.119, 2016.
Hennen, M., Chappell, A., Edwards, B. L., Faist, A. M., Kandakji, T., Baddock, M. C., Wheeler, B., Tyree, G., Treminio, R., and Webb, N. P.: A North American dust emission climatology (2001–2020) calibrated to dust point sources from satellite observations, Aeolian Res., 54, 100766, https://doi.org/10.1016/j.aeolia.2021.100766, 2022.
Herrera-Molina, E., Gill, T. E., Ibarra-Mejia, G., and Jeon, S.: Associations between dust exposure and hospitalizations in El Paso, Texas, USA, Atmosphere-Basel, 12, 1413, https://doi.org/10.3390/atmos12111413, 2021.
Herrera-Molina, E., Gill, T. E., Ibarra-Mejia, G., Jeon, S., and Ardon-Dryer, K.: Associations Between Dust Exposure and Hospitalizations in a dust-prone city, Lubbock, Texas, USA, Air Qual. Atmos. Hlth., 17, 1091–1105, https://doi.org/10.1007/s11869-023-01489-9, 2024.
Huang, H., Qian, Y., Liu, Y., He, C., Zheng, J., Zhang, Z., and Gkikas, A.: Where does the dust deposited over the Sierra Nevada snow come from?, Atmos. Chem. Phys., 22, 15469–15488, https://doi.org/10.5194/acp-22-15469-2022, 2022.
Huang, Y., Gong, X., Liu, L., Luo, L., Leng, S., and Lin, Y.: Maternal exposure to metal components of PM2.5 and low birth weight in New Mexico,USA, Environ. Sci. Pollut. R., 30, 98526–98535, https://doi.org/10.1007/s11356-023-29291-1, 2023.
Hyde, P., Alex Mahalov, A., and Li, J.: Simulating the meteorology and PM10 concentrations in Arizona dust storms using the Weather Research and Forecasting model with Chemistry (Wrf-Chem), J. Air Waste Manage., 68, 177–195, https://doi.org/10.1080/10962247.2017.1357662, 2018.
Iowa Mesonet: Iowa Environmental Mesonet (IEM) ASOS-AWOS-METAR Data, Iowa State University [data set], https://www.mesonet.agron.iastate.edu/request/download.phtml?network=TX_ASOS (last access: 30 December 2023), 2023.
Jaafari, J., Naddafi, K., Yunesian, M., Nabizadeh, R., Hassanvand, M. S., Ghozikali, M. G., Nazmara, S., Shamsollahi, H. R., and Yaghmaeian, K.: Study of PM10, PM2.5, and PM1 levels in during dust storms and local air pollution events in urban and rural sites in Tehran, Hum. Ecol. Risk Assess., 24, 482–493, https://doi.org/10.1080/10807039.2017.1389608, 2018.
Joshi, J. R.: Quantifying the impact of cropland wind erosion on air quality: A high-resolution modelling case study of an Arizona dust storm, Atmos. Environ., 263, 118658, https://doi.org/10.1016/j.atmosenv.2021.118658, 2021.
Jugder, D., Shinoda, M., Kimura, R., Batbold, A., and Amarjargal, D.: Quantitative analysis on windblown dust concentrations of PM10 (PM2.5) during dust events in Mongolia, Aeolian Res., 14, 3–13, https://doi.org/10.1016/j.aeolia.2014.04.005, 2014.
Kandakji, T., Gill, T. E., and Lee, J. A.: Identifying and characterizing dust point sources in the southwestern United States using remote sensing and GIS, Geomorphology, 353, 107019, https://doi.org/10.1016/j.geomorph.2019.107019, 2020.
Karami, S., Ranjbar, A., Mohebalhojeh, A. R., and Moradi, M.: A rare case of haboob in Tehran: Observational and Numerical study, Atmos. Res., 185, 169–185, https://doi.org/10.1016/j.atmosres.2016.10.010, 2017.
Karle, N. N., Mahmud, S., Sakai, R. K., Fitzgerald, R. M., Morris, V. R., and Stockwell, W. R.: Investigation of the Successive Ozone Episodes in the El Paso–Juarez Region in the Summer of 2017, Atmosphere-Basel, 11, 532, https://doi.org/10.3390/atmos11050532, 2020.
Kavouras, I. G., DuBois, D. W., Nikolich, G., and Etyemezian, V.: Monitoring, Source Identification and Health Risks of Air Toxics in Albuquerque, New Mexico, U.S.A., Aerosol Air Qual. Res., 15, 556–571, https://doi.org/10.4209/aaqr.2014.04.0075,2020.
Kelley, M. C. and Ardon-Dryer, K.: Analyzing Two Decades of Dust Events on the Southern Great Plains Region of West Texas, Atmos. Pollut. Res., 12, 101091, https://doi.org/10.1016/j.apr.2021.101091, 2021.
Kelley, M. C., Brown, M. M., Fedler, C. B., and Ardon-Dryer, K.: Long-term measurements of PM2.5 concentrations in Lubbock, Texas, Aerosol Air Qual. Res., 20, 1306–1318, https://doi.org/10.4209/aaqr.2019.09.0469, 2020.
Kim, D., Chin, M., Kemp, E. M., Tao, Z., Peters-Lidard, C. D., and Ginoux, P.: Development of high-resolution dynamic dust source function – A case study with a strong dust storm in a regional model, Atmos. Environ., 159, 11–25, https://doi.org/10.1016/j.atmosenv.2017.03.045, 2017.
Krasnov, H., Kloog, I., Friger, M., and Katra, I.: The Spatio-Temporal Distribution of Particulate Matter during Natural Dust Episodes at an Urban Scale, PLoS One, 11, e0160800, https://doi.org/10.1371/journal.pone.0160800, 2016.
Lader, G., Raman, A., Davis, J. T., and Waters, K.: Blowing dust and dust storms: one of Arizona's most underrated weather hazards, NOAA Tech. Memor. NWS-WR-290, https://www.weather.gov/media/wrh/online_publications/TMs/TM-290.pdf (10 August 2023), 2016.
Lee, J., Gill, T., Mulligan, K., Acosta, M., and Perez, A.: Land use/land cover and point sources of the 15 December 2003 dust storm in southwestern North America, Geomorphology, 105, 18–27, https://doi.org/10.1016/j.geomorph.2007.12.016, 2009.
Lee, J., Baddock, M., Mbuh, M., and Gill, T.: Geomorphic and land cover characteristics of Aeolian dust storces in West Texas and eastern New Mexico, USA, Aeolian Res., 3, 459–466, https://doi.org/10.1016/j.aeolia.2011.08.001, 2012.
Lee, J. A. and Tchakerian, V. P.: Magnitude and Frequency of Blowing Dust on the Southern High Plains of the United States, 1947–1989, Ann. Am. Assoc. Geogr., 85, 684–693, https://doi.org/10.1111/j.1467-8306.1995.tb01820.x, 1995.
Lei, H., Wang, J. X. L., Tong, D. Q., and Lee, P.: Merged dust climatology in Phoenix, Arizona based on satellite and station data, Clim. Dynam., 47, 2785–2799, https://doi.org/10.1007/s00382-016-2997-7, 2016.
Li, J., Kandakji, T., Lee, J. A., Tatarko, J., Blackwell, J., Gill, T. E., and Collins, J. D.: Blowing Dust and Highway Safety in the Southwestern United States: Characteristics of Dust Emission “Hotspots” and Management Implications, Sci. Total Environ., 621, 1023–1032, https://doi.org/10.1016/j.scitotenv.2017.10.124, 2018.
Li, W.-W., Cardenas, N., Walton, J., Trujillo, D., and Morales, H.: PM Source Identification at Sunland Park, New Mexico, Using a Simple Heuristic Metrological and Chemical Analysis, J. Air Waste Manage., 55, 352–364, https://doi.org/10.1080/10473289.2005.10464623, 2005.
Malaguti, A., Mircea, M., La Torretta, T. M., Telloli, C., Petralia, E., Stracquadanio, M., and Berico, M.: Chemical Composition of Fine and Coarse Aerosol Particles in the Central Mediterranean Area during Dust and Non-Dust Conditions, Aerosol Air Qual. Res., 15, 410–425, https://doi.org/10.4209/aaqr.2014.08.0172, 2018.
Malig, B. J. and Ostro, B. D.: Coarse particles and mortality: evidence from a multi-city study in California, J. Occup. Environ. Med., 66, 832–839, https://doi.org/10.1136/oem.2008.045393, 2009.
Mamouri, R.-E., Ansmann, A., Nisantzi, A., Solomos, S., Kallos, G., and Hadjimitsis, D. G.: Extreme dust storm over the eastern Mediterranean in September 2015: satellite, lidar, and surface observations in the Cyprus region, Atmos. Chem. Phys., 16, 13711–13724, https://doi.org/10.5194/acp-16-13711-2016, 2016.
May, R. M., Goebbert, K. H., Thielen, J. E., Leeman, J. R., Camron, M. D., Bruick, Z., Bruning, E. C., Manser, R. P., Arms, S. C., and Marsh, P. T.: MetPy: A meteorological python library for data analysis and visualization [Software], B. Am. Meteorol. Soc., 103, E2273–E2284, https://doi.org/10.1175/BAMS-D-21-0125.1, 2022.
Middleton, N., Tozer, P., and Tozer, B.: Sand and dust storms: underrated natural hazards, Disasters, 43, 390–409, https://doi.org/10.1111/disa.12320, 2019.
Milford, C., Cuevas, E., Marrero, C. L., Bustos, J. J., Gallo, V., Rodríguez, S., Romero-Campos, P. M., and Torres, C.: Impacts of Desert Dust Outbreaks on Air Quality in Urban Areas, Atmosphere-Basel, 11, 23, https://doi.org/10.3390/atmos11010023, 2020.
Mu, H., Otani, S., Shinoda, M., Yokoyama, Y., Onishi, K., Hosoda, T., Okamoto, M., and Kurozawa, Y.: Long-Term Effects of Livestock Loss Caused by Dust Storm on Mongolian Inhabitants: A Survey 1 Year after the Dust Storm, Yonago Acta Med., 56, 39–42, 2013.
Natsagdorj, L., Jugder, D., Chung, Y. S.: Analysis of dust storms observed in Mongolia during 1937–1999, Atmos. Environ., 37, 1401–1411, https://doi.org/10.1016/S1352-2310(02)01023-3, 2003.
New Mexico Environmental Department: Current Air Quality, State of New Mexico [data set], https://aqi.air.env.nm.gov/ (last access: 5 January 2023), 2023.
Nickling, W. G. and Brazel, A. J.: Temporal and spatial characteristics of Arizona dust storms (1965–1980), J. Climatol., 4, 645–660, https://doi.org/10.1002/joc.3370040608, 1984.
Nicoll, K., Hahnenberger, M., and Goldstein, H. L.: “Dust in the wind” from source-to-sink: analysis of the 14–15 April 2015 storm in Utah, Aeolian Res., 46, 100532, https://doi.org/10.1016/j.aeolia.2019.06.002, 2020.
Novlan, D. J., Hardiman, M., and Gill, T. E.: A synoptic climatology of blowing dust events in El Paso, Texas from 1932–2005, 16th Conference on Applied Climatology, Am. Meteorol. Soc., San Antonio, TX, https://www.weather.gov/media/epz/research/elp07-2.pdf (last access: 10 August 2023), 2007.
Orlovsky, L., Orlovsky, N., and Durdyev, A.: Dust storms in Turkmenistan, J. Arid Environ., 60, 83–97, https://doi.org/10.1016/j.jaridenv.2004.02.008, 2005.
Park, S. H., Gong, S. L., Zhao, T. L., Vet, R. J., Bouchet, V. S., Gong, W., Makar, P. A., Moran, M. D., Stroud, C., and Zhang, J.: Simulation of entrainment and transport of dust particles within North America in April 2001 (“Red Dust Episode”), J. Geophys. Res.-Atmos., 112, D20209, https://doi.org/10.1029/2007JD008443, 2007.
Park, S. H., Gong, S. L., Gong, W., Makar, P. A., Moran, M. D., Stroud, C. A., and Zhang, J.: Sensitivity of surface characteristics on the simulation of windblown dust source in North America, Atmos. Environ., 43, 3122–3129, 2009.
Pérez, L., Tobias, A., Querol, X., Kunzli, N., Pey, J., Alastuey, A., Viana, M., Valero, N., Gonzalez-Cabre, M., and Sunyer, J.: Coarse particles from Saharan dust and daily mortality, Epidemiology, 19, 800–807, https://doi.org/10.1097/ede.0b013e31818131cf, 2008.
Raman, A., Arellano Jr., A., and Brost, J.: Revisiting haboobs in the southwestern United States: An observational case study of the 5 July 2011 Phoenix dust storm, Atmos. Environ., 89, 179–188, https://doi.org/10.1016/j.atmosenv.2014.02.026, 2014.
Reynolds, R. L., Munson, S. M., Fernandez, D., Goldstein, H. L., and Neff, J. C.: Concentrations of mineral aerosol from desert to plains across the central Rocky Mountains, western United States, Aeolian Res., 23, 21–35, https://doi.org/10.1016/j.aeolia.2021.100766, 2016.
Rivera Rivera, N. I., Gill, T. E., Gebhart, K. A., Hand, J. L., Bleiwess, M. P., and Fitzgerald, R. M.: Wind modeling of Chihuahuan Desert, Atmos. Environ., 43, 347–354, https://doi.org/10.1016/j.atmosenv.2008.09.069, 2009.
Robinson, M. C. and Ardon-Dryer, K.: Characterization of 21 Years of Dust Events Across Four West Texas Regions, Aeolian Res., 67–69, 100930, https://doi.org/10.1016/j.aeolia.2024.100930, 2024.
Sandhu, T., Kelley, M., Rawlins, E., and Ardon-Dryer, K.: Identification of dust events in the greater Phoenix area, Atmos. Pollut. Res., 15, 102275, https://doi.org/10.1016/j.apr.2024.102275, 2024.
Schweitzer, M., Calzadilla, A., Salamo, O., Sharifi, A., Kumar, N., Holt, G., Campos, M., and Mirsaeidi, M.: Lung health in era of climate change and dust storms, Environ. Res., 163, 36–42, https://doi.org/10.1016/j.envres.2018.02.001, 2018.
Shao, J. and Mao, J.: Dust particle size distributions during spring in Yinchuan, China, Adv. Meteorol., 2016, 6940502, https://doi.org/10.1155/2016/6940502, 2016.
Sorribas, M., Adame, J. A., Andrews, E., and Yela, M.: An anomalous African dust event and its impact on aerosol radiative forcing on the Southwest Atlantic coast of Europe in February 2016, Sci. Total Environ., 583, 269–279, https://doi.org/10.1016/j.scitotenv.2017.01.064, 2017.
Stout, J. E.: Dust and environment in the southern high Plains of North America, J. Arid Environ., 47, 425–441, https://doi.org/10.1006/jare.2000.0732, 2001.
Stout, J. E.: Diurnal patterns of blowing dust on the Llano Estacado, J. Arid Environ., 122, 85–92, https://doi.org/10.1016/j.jaridenv.2015.06.013, 2015.
Stout, J. E. and Arimoto, R.: Threshold wind velocities for sand movement in the Mescalero Sands of Southeastern New Mexico, J. Arid Environ., 74, 1456–1460, https://doi.org/10.1016/j.jaridenv.2010.05.011, 2010.
Sugimoto, N., Shimizu, A., Matsui, I., and Nishikawa, M.: A method for estimating the fraction of mineral dust in particulate matter using PM2.5-to-PM10 ratios, Particuology, 28, 114–120, https://doi.org/10.1016/j.partic.2015.09.005, 2016.
T640: Teledyne API: Model T640 PM Mass Monitor, https://www.teledyne-api.com/prod/Downloads/SAL000090E%20-%20T640.pdf, last access: 25 July 2024.
TCEQ (Texas Commission on Environmental Quality): Air Quality and Monitoring, https://www.tceq.texas.gov/cgi-bin/compliance/monops/monthly_summary.pl?cams=1028 (last access: 30 December 2023), 2023.
Tong, D. Q., Dan, M., Wang, T., and Lee, P.: Long-term dust climatology in the western United States reconstructed from routine aerosol ground monitoring, Atmos. Chem. Phys., 12, 5189–5205, https://doi.org/10.5194/acp-12-5189-2012, 2012.
Tong, D. Q., Gorris, M. E., Gill, T. E., Ardon-Dryer, K., Wang, J., and Ren, L.: Dust storms, Valley fever, and public awareness, GeoHealth, 6, e2022GH000642, https://doi.org/10.1029/2022GH000642, 2022.
Tong, D., Feng, I., Gill, T. E., Shepenski, K., and Wang, J.: How Many People Were Killed by Windblown Dust Events in the United States?, B. Am. Meteorol. Soc., 104, 1067–1084, https://doi.org/10.1175/BAMS-D-22-0186.1, 2023.
Toure, N. O., Gueye, N. R.-D., Diokhane, A. M., Jenkins, G. S., Li, M., Drame, M. S., Coker, K. R., and Thiam, K.: Observed and modeled seasonal air quality and respiratory health in Senegal during 2015 and 2016, GeoHealth, 3, 423–442, https://doi.org/10.1029/2019GH000214, 2019.
Van Pelt, R. S., Shekhter, E. G., Barnes, M. A. W., Duke, S. E., Gill, T. E., and Pannell, K. H.: Spatial and temporal patterns of heavy metal deposition resulting from a smelter in El Paso, Texas, J. Geochem. Explor., 210, 1–8, https://doi.org/10.1016/j.gexplo.2019.106414, 2020.
Vukovic, A., Vujadinovic, M., Pejanovic, G., Andric, J., Kumjian, M. R., Djurdjevic, V., Dacic, M., Prasad, A. K., El-Askary, H. M., Paris, B. C., Petkovic, S., Nickovic, S., and Sprigg, W. A.: Numerical simulation of “an American haboob”, Atmos. Chem. Phys., 14, 3211–3230, https://doi.org/10.5194/acp-14-3211-2014, 2014.
Wang, S., Wang, J., Zhou, Z., and Shang, K.: Regional characteristics of three kinds of dust storm events in China, Atmos. Environ., 39, 509–520, https://doi.org/10.1016/j.atmosenv.2004.09.033, 2005.
White, J. R., Balling, R. C., and Cerveny, R. S.: Trajectory analysis of central Sonoran Desert dust storms, J. Arid Environ., 219, 105077, https://doi.org/10.1016/j.jaridenv.2023.105077, 2023.
WHO (World Health Organization): Global air quality guidelines, https://iris.who.int/bitstream/handle/10665/345329/9789240034228-eng.pdf, last access: 30 December 2023.
WMO (World Meteorological Organization): WMO Technical Regulations Annex II Manual on Codes International Codes, I.1, https://community.wmo.int/en/activity-areas/wis/volume-i1 (last access: 10 August 2023) 2019.
Yin, D., Nickovic, S., Barbaris, B., Chandy, B., and Sprigg, W. A.: Modeling windblown desert dust in the southwestern United States for public health warning: a case study, Atmos. Environ., 39, 6243–6254, https://doi.org/10.1016/j.aeolia.2024.100930, 2005.
Yu, H. L. and Wang, C. H.: Retrospective prediction of intraurban spatiotemporal distribution of PM2.5 in Taipei, Atmos. Environ., 44, 3053–3065, https://doi.org/10.1016/j.atmosenv.2010.04.030, 2010.
Zanobetti, A. and Schwartz, J.: The effect of fine and coarse particulate air pollution on mortality: a national analysis, Environ. Health Persp., 117, 898–903, https://doi.org/10.1289/ehp.0800108, 2009.
Zobeck, T. M. and VanPelt, R. S.: Wind induced dust generation and transport mechanics on a bare agriculture field, J. Hazard. Mater., 132, 26–38, https://doi.org/10.1016/j.jhazmat.2005.11.090, 2006.