the Creative Commons Attribution 4.0 License.
the Creative Commons Attribution 4.0 License.
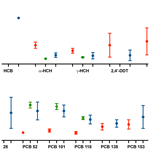
Levels of persistent organic pollutants (POPs) in the Antarctic atmosphere over time (1980 to 2021) and estimation of their atmospheric half-lives
Thais Luarte
Victoria A. Gómez-Aburto
Ignacio Poblete-Castro
Eduardo Castro-Nallar
Nicolas Huneeus
Marco Molina-Montenegro
Claudia Egas
Germán Azcune
Andrés Pérez-Parada
Rainier Lohmann
Pernilla Bohlin-Nizzetto
Jordi Dachs
Susan Bengtson-Nash
Gustavo Chiang
Karla Pozo
Cristóbal J. Galbán-Malagón
Persistent organic pollutants (POPs) are synthetic compounds that were intentionally produced in large quantities and have been distributed in the global environment, originating a threat due to their persistence, bioaccumulative potential, and toxicity. POPs reach the Antarctic continent through long-range atmospheric transport (LRAT). In these areas, low temperatures play a significant role in the environmental fate of POPs, retaining them for a long time due to cold trapping by diffusion and wet deposition, acting as a net sink for many POPs. However, in the current context of climate change, the remobilization of POPs that were trapped in water, ice, and soil for decades is happening. Therefore, continuous monitoring of POPs in polar air is necessary to assess whether there is a recent re-release of historical pollutants back to the environment. We reviewed the scientific literature on atmospheric levels of several POP families (polychlorinated biphenyls – PCBs, hexachlorobenzene – HCB, hexachlorocyclohexanes – HCHs, and dichlorodiphenyltrichloroethane – DDT) from 1980 to 2021. We estimated the atmospheric half-life using characteristic decreasing times (TD). We observed that HCB levels in the Antarctic atmosphere were higher than the other target organochlorine pesticides (OCPs), but HCB also displayed higher fluctuations and did not show a significant decrease over time. Conversely, the atmospheric levels of HCHs, some DDTs, and PCBs have decreased significantly. The estimated atmospheric half-lives for POPs decreased in the following order: 4,4' DDE (13.5 years) > 4,4' DDD (12.8 years) > 4,4' DDT (7.4 years) > 2,4' DDE (6.4 years) > 2,4' DDT (6.3 years) > α-HCH (6 years) > HCB (6 years) > γ-HCH (4.2 years). For PCB congeners, they decreased in the following order: PCB 153 (7.6 years) > PCB 138 (6.5 years) > PCB 101 (4.7 years) > PCB 180 (4.6 years) > PCB 28 (4 years) > PCB 52 (3.7 years) > PCB 118 (3.6 years). For HCH isomers and PCBs, the Stockholm Convention (SC) ban on POPs did have an impact on decreasing their levels during the last decades. Nevertheless, their ubiquity in the Antarctic atmosphere shows the problematic issues related to highly persistent synthetic chemicals.
- Article
(1674 KB) - Full-text XML
-
Supplement
(739 KB) - BibTeX
- EndNote
Persistent organic pollutants (POPs) are a group of toxic chemicals primarily produced and used by the agricultural, industrial, and household applications during the third industrial revolution (Safe, 1994; Qiu et al., 2004; Jayaraj et al., 2016). In the last 3 decades, studies have reported that POP levels have soared in the environment worldwide, as these chemicals are highly stable and resistant to degradation (Pennington, 2001). This persistence and their hydrophobicity result in POP bioaccumulation within organisms and biomagnification along food webs (Hop et al., 2002; Fisk et al., 2001a, b; Borga and Di Guardo, 2005), where they may elicit toxic effects, such as endocrine disruption, threatening the health of both wildlife and humans (Brown et al., 2014; Bourgeon et al., 2012). Given their detrimental effects, 35 POP substances are currently regulated internationally by the Stockholm Convention (SC), which seeks to reduce and eliminate POP production and use (UNECE, 1999; UNEP, 2001). However, despite regulatory action among SC signatory nations, considerable levels of POPs are still detected in water, atmosphere, biota, and sediments worldwide due to their persistence and potential for long-range transport, as well as their current emission sources (e.g., Vergara et al., 2019; Vasseghian et al., 2021; Avila et al., 2021; Die et al., 2021; Garcia-Cegarra et al., 2021). Of utmost concern is that these toxic pollutants are present in the environmental compartments of regions far from emission sources that have previously been considered pristine areas, including polar regions (Galbán-Malagón et al., 2013a, b, c; Pozo et al., 2017; Wu et al., 2020; Azcune et al., 2022; Xie et al., 2022).
The Antarctic continent is the most remote region from primary sources of POPs (Von Waldow et al., 2010). POPs reach Antarctica mainly through long-range atmospheric transport (LRAT), which generally occurs by the process known as “grasshopping”, consisting of successive atmospheric volatilization and depositions (Blais et al., 2007; Brown and Wania, 2008; Bengtson-Nash, 2011; Jurado and Dachs, 2008). Ocean currents also contribute to their transport processes, albeit at longer timescales since the Antarctic Circumpolar Current acts as a barrier limiting the oceanic transport of POPs to the Antarctic continent (Bengtson-Nash, 2011). The “barrier theory” has been questioned by Lozoya et al. (2022) for the South Shetland Islands, where the current experiences topographical forcing through the Drake Passage. Finally, another minor transport process is biological, mediated by migratory biota (Braune et al., 2005; Wild et al., 2022). In addition, there may be local sources of POPs, such as research stations and tourist hotspots, that can contribute to detectable and sometimes elevated concentrations of POPs. For example, PCBs have been reported in the vicinity of such local sources (Larsson et al., 1992; Risebrough et al., 1990; Hale et al., 2008). The low temperatures of Antarctica play an important role in the environmental fate of POPs, repressing re-volatilization processes and favoring cold trapping (Wania and Mackay, 1996; Casal et al., 2019), limiting any potential degradation, and enhancing bioaccumulation. In this context, several studies show that polar regions act as a net sink for many POPs; Antarctica is a vast continent covered in ice surrounded by the Southern Ocean, hence chemicals deposited through LRAT will first deposit in these compartments (Mackay and Wania, 1995; Kallenborn et al., 1998; Dickhut et al., 2005; Gioia et al., 2008; Cincinelli et al., 2009; Baek et al., 2011; Cabrerizo et al., 2017; Galbán-Malagón et al., 2012, 2013a, c; Montone et al., 2013). For example, there is evidence supporting oceanic sequestration by the biological pump during blooms that bury these compounds on the seafloor (Galbán-Malagón et al., 2013a, c) or by biodegradation due to the microbial loop (Galbán-Malagón et al., 2013d). In the context of rapid climate change experienced in polar regions, the remobilization of POPs previously trapped in water, ice, and soil for decades is expected (Nizzetto et al., 2010; Ma et al., 2011; Cabrerizo et al., 2013). The re-emission of POPs to the environment will affect global efforts to moderate human and environmental exposure to these toxic compounds (Bigot et al., 2016); therefore, continuous monitoring of POP levels in polar abiotic matrices is necessary to assess the extent to which such re-emissions to the atmosphere occur.
The detection of chemicals in remote regions serves as direct empirical evidence of a compound's persistence and potential for long-term environmental transport (Bengston-Nash et al., 2017). POPs were first reported in Antarctic biota in the 1960s (Sladen et al., 1966; Tatton and Ruzicka, 1967), sparking interest in studying the transport, fate, and levels present in different environmental compartments. Through the collation of decades of coordinated monitoring data of POPs in the Arctic atmosphere, studies have explored the fate, sources, and long-range transport of POPs in the Northern Hemisphere (Hung et al., 2010, 2016; Wu et al., 2010, 2011). A general downward trend of many airborne POPs has been demonstrated in the Arctic (Hung et al., 2010, 2016; Kong et al., 2014). However, continuous and consistent atmospheric measurements on POPs in Antarctica are limited, due to the remote geographical location and complex climatic conditions of this continent, which put logistical constraints on any monitoring program. These knowledge gaps make it difficult to understand the fundamental patterns of POPs in this area (Bengston-Nash, 2011) but also facilitate systematic comparison with studies conducted in the Arctic.
This paper presents the first systematic review of the most reported POPs in the Antarctic atmosphere, allowing us to summarize the data collected by the different studies and compare the concentrations recorded over the years and at the different sampling sites. Such a compilation allows the identification of temporal trends and calculate the atmospheric half-lives of the predominant POPs being monitored to provide insights into expected impacts of environmental remobilization under changing Antarctic conditions.
2.1 Compilation of bibliographical data
We reviewed all published studies on atmospheric levels of the most reported POP families in the Antarctic atmosphere (polychlorinated biphenyls (PCBs), hexachlorobenzene (HCB), hexachlorocyclohexane (HCH), and dichlorodiphenyltrichloroethane (DDT) and its degradation products) from 1980 to 2021. An exhaustive search was performed in the Web of Science and Scopus databases using the words “Persistent Organic Pollutants”, “atmospheric”, and “Antarctica”, including only articles written in English; excluding from the analysis references that do not refer to a good quality assurance and quality control measures. Thus, studies not reporting information about blank samples, limits of detection, limits of quantification, and/or instrumental detection limits, and referring to previous works reporting the quality criteria used, were not included in the present study. This is important because the reported concentrations are at very low levels, and to avoid bias, it is necessary to be sure about the quality measures of sample collection and analysis. A total of 34 publications were found, from which we retrieved data on the levels reported, the year in which the samples were collected, and the sampling sites (Tables 1, 2 and 3). We worked exclusively with the levels of the target compounds in the gas phase, obtained from active and passive sampling. Furthermore, compounds scarcely reported in the Antarctic atmosphere, such as polybrominated diphenyl ethers (PBDEs), polycyclic aromatic hydrocarbons (PAHs), and per- and polyfluoroalkyl substances (PFASs), were excluded.
Table 1HCB and HCH levels (pg m−3) in the Antarctic atmosphere from 1980 to present. ∑n indicates the number of isomers included in the study. The notation “n.d” indicates “not detected”.
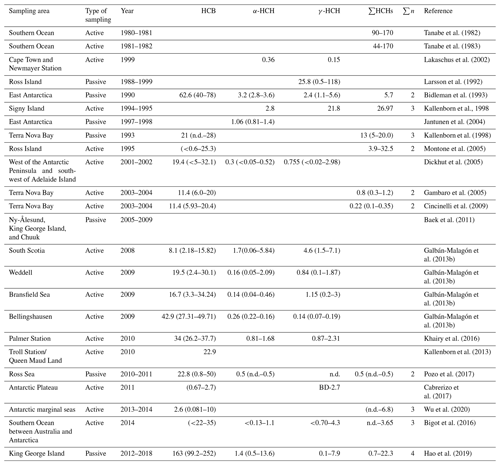
2.2 Statistical analysis
To evaluate the differences between the levels present in West Antarctica and East Antarctica, a non-parametric Mann–Whitney U variance analysis was conducted. To estimate the trend in the change of concentrations, a linear regression was performed between the natural logarithm of the concentrations for each year studied. Finally, a generalized linear model (GLM) was performed to elucidate whether the variability in the atmospheric POP levels reviewed is due to the different types of sampling used by the different studies (active or passive sampling) or the time variable. All the analyses were performed using the R statistical software (R Core Team, 2022).
2.3 Estimation of characteristic decreasing times (TD)
Atmospheric half-lives were estimated by deriving the e-folding or characteristic decreasing times (TD), following the methodology of Galbán-Malagón et al. (2013a). The half-live is defined as the time needed to decrease the atmospheric concentration by 35 % (e−1) of its initial concentration, which is given by 0.69 TD. First, only the studies that reported all the values recorded for each sample were used (Tables S1 and S2). These studies were ordered by year of sampling, and their respective TD was calculated by least squares adjusting the concentrations to Eq. (1):
where kd is the inverse of the e-folding time TD (in years), t is the time in years, and b is the independent term; TD was not calculated for β-HCH, due to the limited data available.
3.1 Organochlorine pesticides (OCPs)
Organochlorine pesticides (OCPs) represent most of the POPs listed in the Stockholm Convention. These organic compounds have been widely produced and commercialized since the 1950s for agricultural use and vector control (UNECE, 1999; UNEP, 2001). The application of technical HCH in agriculture has been banned since the early 1980s, while DDT, Lindane (γ-HCH), and HCB were banned in the 1990s (UNECE, 1999; UNEP, 2001). The OCPs were first reported in Antarctic marine biota in the late 1960s by Sladen et al. (1966) and Tatton and Ruzicka (1967). To date, their levels in different environmental compartments continue to be reported (e.g., Vergara et al., 2019; Wu et al., 2020; Krasnobaev et al., 2020; Xie et al., 2022).
3.1.1 Atmospheric levels of organochlorine pesticides (OCPs)
In the Arctic atmosphere, HCB concentrations are the highest of any OCPs (De March et al., 1998). Similarly, atmospheric concentrations of HCB reported from the Antarctic have been observed to be higher than the other target OCPs (Tables 1 and 2), being the most frequently detected and abundant POP in the Antarctic atmosphere (Kallenborn et al., 2013; Wang et al., 2018; Hao et al., 2019; Wu et al., 2020). Temporal patterns of atmospheric HCB concentrations in the Antarctic show significant interannual fluctuations with low but significant decreasing trend (p<0.001, See Table 1), with a higher variability over time,specially in the last decade (Fig. 1a). A clear decrease in concentrations is shown until about 2010; thereafter, a large variability of data is shown where the trend seems to be changing. However, there is a lack of sufficient data to be able to confirm this trend. The maximum values were reported by Hao et al. (2019) during the 2012–2018 sampling period on King George Island (Table 1). Such increases in HCB gaseous levels could be mainly associated with re-emission from environmental surfaces (water, soil, and snow) shifting from a reservoir to a secondary source of this compound on the Antarctic continent. The HCB is the most persistent OCP chemical assessed here, as suggested before (Galbán-Malagón et al., 2013b). In addition, there may still be an important influence of transport from current primary sources (i.e., combustion and thermal processes) on a global scale and unintentional formation during thermal processing or combustion of chlorine-containing materials (Barber et al., 2005). The trend shown in Fig. 1a points out that concentrations of HCB in the Antarctic atmosphere may be regionally dependent and may be high due to the climate and/or environmental change processes occurring in different Antarctic regions.
The reported atmospheric concentrations of ΣHCHs in Antarctica from 1980 to 2019 show a decreasing trend over time (Table 1; Fig. 1b and c), with significant differences in interannual levels (P<0.05). The maximum concentration of HCHs was 170 pg m−3, reported in 1980–1982 (Tanabe et al., 1982, 1983), and progressively lower concentrations reaching values under detection levels and below 1 pg m−3 are reported from 2003 to 2019 (Gambaro et al., 2005; Cincinelli et al., 2009; Baek et al., 2011; Galbán-Malagón et al., 2013b; Kallenborn et al., 2013; Pozo et al., 2017; Cabrerizo et al., 2017; Wu et al., 2020; Bigot et al., 2016; Hao et al., 2019). The γ-HCH isomer was found at high concentrations in Antarctica between 1989 and 1990, with a maximum atmospheric concentration of 118 pg m−3 in 1988 at Ross Island, by Larsson et al. (1992) (Fig. 1c, Table S1). Decreasing concentrations are then reported for γ-HCH in 2000, which is unsurprising if fresh sources have been removed, given the lower volatility and higher water solubility of this isomer. On the other hand, the α-HCH isomer, has been increasing since 2006 (Baek et al., 2011; Galbán-Malagón et al., 2013b; Hao et al., 2019), compared to the concentrations recorded during 2001–2004 by Dickhut et al. (2005) and Cincinelli et al. (2009).
Published studies reporting gaseous levels for DDT and their isomers from 1988–2021 were lower than the rest of the target OCPs, and like HCHs, the DDTs showed a decreasing trend over the years (Table 2, Fig. 2), with significant interannual differences (p<0.05) for compounds 4,4'-DDT, 4,4'-DDE, 2,4'-DDT, and 2,2'-DDE, and non-significant annual differences (p>0.05) for compounds 4,4'-DDD and 2,4'-DDD.
To date, atmospheric concentrations of HCB, α-HCH, β-HCH, γ-HCH, 2,4'-DDTs, 4,4'-DDTs, and 2,4' DDD isomers have been studied over much of the Antarctic continent, both in West Antarctica (Kallenborn et al., 1998; Montone et al., 2005; Dickhut et al., 2005; Baek et al., 2011; Galbán-Malagón et al., 2013c; Khairy et al., 2016; Hao et al., 2019) and in East Antarctica (Tanabe et al., 1982, 1983; Lakaschus et al., 2002; Larsson et al., 1992; Jantunen et al., 2004; Bidleman et al., 1993; Gambaro et al., 2005; Cincinelli et al., 2009; Kallenborn et al., 2013; Pozo et al., 2017; Cabrerizo et al., 2017; Wu et al., 2020; Bigot et al., 2016). The detected concentrations of HCB, α-HCH, and 4,4'-DDT indicate significant spatial differences (P<0.05), with higher atmospheric concentrations in West Antarctica than in East Antarctica (Table S4). The γ-HCH and 2,4'-DDT isomers did not show spatial differences between the two zones (P>0.05) (Table S4), but the usage of these compounds decreased in a similar way from 1990 to 2000 (Vijgen, 2006). This can be explained by two causes together. The first is the greater proximity of South America to the Antarctic Peninsula. The proximity itself has to do with the possibility of transport of these compounds from southern South America where it is suggested that air samples influenced by the continent are capable of transporting pollutants from South America to Antarctica (Dickhut et al., 2005) such as Heptachlor epoxide. However, when looking to usage reported in South America compared to Africa (Li, 1999), this could influence the abundance of α-HCH in the western Antarctic area. Examining previous information for both HCB and 4,4'-DDT, there is not a great deal of information about the use of these compounds in areas near Antarctica, but the proximity to South America could explain these variations in conjunction with the paucity of data in East Antarctica. On the other hand, the Mann–Whitney U variance analysis was not performed for the β-HCH and 2,4'-DDD isomers, because all levels reported in East Antarctica were below the detection limit. In addition, the results of the generalized linear model indicate that the variability of atmospheric OCPs is mainly due to the year variable, with no significant differences (p>0.05) between the atmospheric levels obtained from active and passive sampling (see Table S5).
3.1.2 Atmospheric half-lives of organochlorine pesticides (OCPs)
The half-life was estimated for all OCP compounds with significant interannual differences (p<0.05 or lower). The estimated half-lives decreased in the following order: 4,4'-DDT (17.2 years) > 2,4'-DDT (14.4 years) > α-HCH (14.3 years) > HCB (14.0 years) > γ-HCH (10.1 years) (more details are given in Table 4). The higher atmospheric half-life values estimated in this study for DDTs isomers, compared to the values estimated for HCHs and HCB, might be related to the years in which these compounds were banned, since DDTs were banned approximately 10 years after HCHs isomers. It may also be due to continuous production and use of DDTs in some parts of the world due to exemptions to the Stockholm Convention. The estimated values are higher than the atmospheric half-lives reported by other authors, such as Atkinson (1986); Howard (1991); Mortimer and Connel (1995); and Kelly et al. (1994), whose estimated and published values do not exceed 1 year. However, the methodologies employed differ from the one used in the present study where Atkinson (1986); Howard (1991); and Mortimer and Connel (1995) were based on rate constant of gas-phase reaction with OH radical for trichlorobiphenyls, while Kelly et al. (1994) were based on atmospheric transformation lifetime. On the other hand, if we compare studies with similar methodology, the study by Venier and Hites (2010) in Great Lakes shows that the half-life estimates for α-HCH and γ-HCH are in a similar range to our estimates, while the one obtained for 4,4'-DDT is slightly lower (Fig. 4a). Similarly, according to the half-life estimates by Wong et al. (2021), HCB shows higher values than those reported by us, but they report similar values than ours for α-HCH, γ-HCH, 2,4'-DDT, and 4,4'-DDT (Fig. 4a).
Polar areas are often considered to be a net sink for POPs. Studies have documented that α-HCH and γ-HCH exchanges preferentially from air to water, with this diffusion being the predominant atmospheric deposition mechanism (Galbán-Malagón et al., 2013a, c; Dickhut et al., 2005; Cincinelli et al., 2009; Jantunen et al., 2004; Lohmann et al., 2009; Xie et al., 2011; Zhang et al., 2012; Huang et al., 2013). Once deposited onto surface waters, they are susceptible to sequestration by the biological pump (Galbán-Malagón et al., 2013a, c), as well as to degradation driven by hydrolysis and biodegradation to a minor extent (Harner et al., 2000; Helm et al., 2002; Galbán-Malagón et al., 2013c). These processes minimize the opportunity for re-entry into the atmosphere through volatilization. The lower half-life values for HCHs may be related to their lower Henry's law constant (HLC) when compared to other POPs. On the contrary, to our knowledge, no degradation processes have been documented for HCB in surface water, and, furthermore, conditions close to air–water equilibrium have been reported for this compound (Cincinelli et al., 2009; Galbán-Malagón et al., 2013c). Similarly, DDTs are more hydrophobic with much higher KOW values than HCHs (Table S3), so they are rapidly removed from seawater as particles sink (Lohmann et al., 2007). Thus, it is possible that the high half-lives estimated for DDTs and their metabolites DDD and DDE may be due to unknown current primary and secondary sources (Voldner and Li, 1995; Channa et al., 2012, Li et al., 2020).
3.2 Polychlorinated biphenyls (PCBs)
Like OCPs, polychlorinated biphenyls (PCBs) were among the first groups of POPs to be listed under the Stockholm Convention and are characterized by their high chemical stability. Prior to their regulatory control in the 1970s, commercial mixtures of PCBs were widely used in many industrial applications, such as fluids in transformers and capacitors, hydraulic fluids, lubricating oils, and as additives in pesticides, inks and paints, flame retardants, plasticizers, sealants for wood and cement surfaces, among others (Kennish, 2017; FAO/UNEP 1992).
The PCBs were first reported in Antarctica in the 1960s and 1970s (Risebrough et al., 1968, 1976), and since then, numerous studies have reported their levels in air, water, sediments, snow, and biota on the Antarctic continent (e.g., Kallenborn et al., 1998; Fuoco et al., 1995; Gupta et al., 1996; Weber et al., 2003; Kim et al., 2015). Here, we selected seven indicator PCB congeners (28, 52, 101, 118, 138, 153, and 180) considering that they are the most reported PCB congeners worldwide, including Antarctica.
3.2.1 Atmospheric levels of polychlorinated biphenyls (PCBs)
The atmospheric concentrations of Σ7PCBs reported by the reviewed studies were below those of the target OCPs (Table 3). Overall, the levels of Σ7PCBs reported from 1980 to 2021 showed a decreasing trend over time (Tables 3 and 4, Fig. 3), with significant differences in their levels (p<0.05). Congeners 28 and 52 recorded the highest concentrations on King George Island, with values of 69.9 pg m−3 in 1995, and 33.2 pg m−3 in 1996, reported by Montone et al. (2005, 2003) (Fig. 3a and b, Table S2). In contrast, the lowest concentrations of all target PCBs were reported for congener 180, ranging from not detected (n.d.) to 3.4 pg m−3 (Fig. 3g, Table S2).
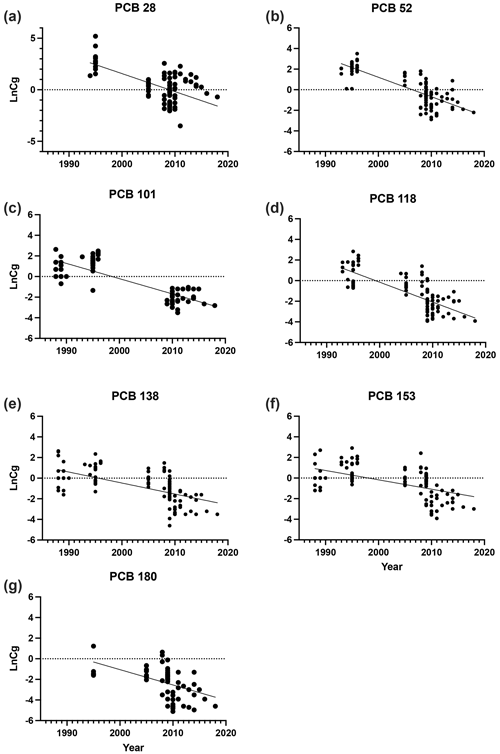
Figure 3Atmospheric levels (pg m−3) of (a) PCB-28, (b) PCB-52, (c) PCB-101, (d) PCB-118, (e) PCB-138, (f) PCB-153, and (g) PCB-180 over time.
Like OCPs, atmospheric concentrations of the seven PCB congeners have been reported over most of the Antarctic zone, covering the zone in West Antarctica (Montone et al., 2003, 2005; Kallenborn et al., 1998; Baek et al., 2011; Galbán-Malagón et al., 2013c; Li et al., 2012a, b; Khairy et al., 2016; Wang et al., 2017; Hao et al., 2019; Wu et al., 2020) and East Antarctica (Larsson et al., 1992; Gambaro et al., 2005; Kallenborn et al., 2013; Pozo et al., 2017; Cabrerizo et al., 2017). Significant spatial differences (p<0.05) were observed in the atmospheric concentrations of congeners 28, 52, 101, and 138, with higher concentrations in West Antarctica than East Antarctica, while there was no significant difference among sites for congeners 101, 118, and 153 (p>0.05). These differences are consistent with the different atmospheric patterns over the Antarctic peninsula regions, with entrance of air masses from the north, and more permanent wet deposition events by snow and rain, increasing the regional concentrations of POPs (Casal et al., 2019; Casas et al., 2021). On the other hand, it is essential to highlight that the variability of PCBs reported in this study is substantially due to the time variable (p<0.05), with no significant differences (p>0.05) between the atmospheric levels of PCBs obtained from active and passive sampling (see Table S5).
3.2.2 Atmospheric half-lives of polychlorinated biphenyls (PCBs)
The estimated atmospheric half-lives for target PCBs decreased in the following order: PCB 153 (7.6 years) > PCB 138 (6.5 years) > PCB 101 (4.7 years) > PCB 180 (4.6 years) > PCB 28 (3.9 years) > PCB 52 (3.7 years) > PCB 118 (3.6 years) (Table 4). The estimated half-lives were directly proportional to the congener's Henry's law constant (HLC) values. (Table S3). Studies by Atkinson (1986) and Sinkkonen and Paasivirta (2000) reported half-lives lower than those estimated in the present work, where none of the estimated half-lives for these compounds exceeded 1 year. However, the methodology of both studies differs from that of the present study, calculating the half-lives of the compounds by means of the rate constant of gas-phase reaction with the OH. Regarding studies using a similar methodology, the atmospheric half-lives estimated by Venier and Hites (2010) in the Great Lakes (United States and Canada) and by Wong et al. (2021) in the Arctic were higher relative to our results for PCBs 28, 52, 101, and 118. They were in a similar range for PCBs 138, 153, and 180 (Fig. 4b).
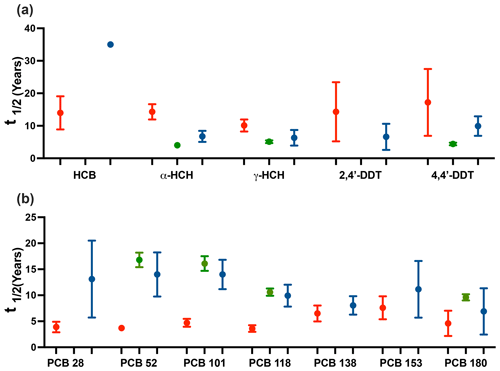
Figure 4Comparison among the estimated atmospheric half-lives obtained in the present work (green) compared with similar estimations from the Great Lakes (red; Venier and Hites, 2010) and the Arctic (blue; Wong et al., 2021) for (a) organochlorine pesticides (HCB, α-HCH, γ-HCH, 2,4'-DDT,and 4,4'-DDT) and (b) polychlorinated biphenyls (28, 52, 101, 118, 138, 153, and 180).
Studies have documented that the biological pump is highly efficient for PCBs with high hydrophobicity, i.e., high KOW values (Table S3) (Dachs et al., 2002; Galbán-Malagón et al., 2012, 2013a), thus reducing their re-volatilization. The estimated atmospheric half-lives, however, do not reflect lower values for the compounds with higher KOW (e.g., PCB 138, 153 and 180), so other factors may be influencing the high estimated half-lives of the more hydrophobic PCBs. One of these factors could be the presence of local sources of certain PCB congeners, since it has been reported that higher PCB concentrations are monitored near the research stations compared to sites farther away from these stations, specifically PCB congeners 28, 52, 56, and 101 (Li et al., 2012b; Montone et al., 2003). Furthermore, remobilization of PCBs stored in soils and ice (Cabrerizo et al., 2013; Casal et al., 2019) could be another factor modulating the surface, and thus atmospheric, concentrations of POPs.
3.3 Influence of global climate change on the dynamics of POPs in the Antarctic continent
Over the past decades, global climate changes and the effects of increasing temperatures have been observed in the Northern and Southern hemispheres (Hung et al., 2022). Increases in ambient temperature can influence physical and chemical processes and ecosystem changes. For example, it has been reported that increasing ambient temperature will affect the dynamics and exchange of POPs between different environmental matrices. Some studies have exposed the relationship between climate change and POP concentrations (Vorkamp et al., 2022; Potapowicz et al., 2018), describing how POPs are temporarily stored in sediments/soils and can be released into the environment with thawing permafrost (Potapowicz et al., 2018) and that there is an increase in POP availability following iceberg calving (Vorkamp et al., 2022) or increased soil remobilization by up to 45 % (Cabrerizo et al., 2013). In addition, several studies show that seawater, snow, and presumably Antarctic soil are becoming critical secondary sources for POP remobilization (Cabrerizo et al., 2012, 2013; Klanová et al., 2008; Casal et al., 2019).
On the other hand, the mean annual air temperature along the western Antarctic Peninsula has been reported to have increased by as much as 3.4 ∘C. In addition, the mid-winter temperature increased by 6.0 ∘C over the past 50 years, making the region one of the most critically affected by climate change (Vaughan et al., 2003; Turner et al., 2005). However, evidence from field sampling indicated that, to date, there is no relationship between atmospheric ambient temperature and atmospheric concentrations of HCH and HCB in East Antarctica (Bengtson-Nash et al., 2017). On the other hand, increasing ambient temperature leads to decreased snow cover, nutrient runoff from land to sea, and increased bioavailability of nutrients on land, causing an increase in primary producers on land and sea (Wasley et al., 2006). In this context, it has been demonstrated in aquatic systems that an increase in primary productivity is vital in the sedimentation processes of POPs from the surface to the aquatic bottom through the biological pump (Larsson et al., 2000; Galbán-Malagón et al., 2018). This process contributes mainly to the removal of POPs from the environment, despite the adverse effects of an increase in primary productivity in ecosystems (e.g., increase in organic matter). Thus, global warming in Antarctica not only implies a temperature change but also leads to multiple processes that can affect the biogeochemical dynamics of POPs, which plays an essential role in the environmental fate of POPs. Depending on the future effects of climate change, Antarctica can act as a secondary source of POPs through the re-volatilization of these compounds or as a sink, contributing to the decrease of their environmental levels. Therefore, future exploration of the impact of climate change is necessary and establishes the importance of establishing long-term monitoring networks.
3.4 Potential sources of bias
As presented here, several factors can be considered as sources of bias from historical data analysis. First, in the time frame of this study (1980–2021), analytical instrumentation and laboratory techniques exhibited dramatic change, particularly with the advent of advanced mass spectrometry (MS) over electron capture detection (ECD) or novel calibration techniques based on isotopically labeled standards (Azcune et al., 2022). Therefore, recent data are generated by more sophisticated techniques and modern laboratory QA/QC criteria. On the other hand, we also included studies using active and passive sampling, but no major differences in the values obtained were observed (See Tables 1, 2, and 3) for the whole compounds which agrees with intercalibration experiments conducted in other areas comparing passive and active sampling together (Prats et al., 2022). The published information from Antarctica is reduced to a group of individual experiences in different geographical locations of international teams working in the field under different conditions and levels of competence that are difficult to obtain and analyze. One might expect that studies that show a strong track record in Antarctic research, reporting POP levels over a time series, might have greater validity due to constancy and consistency in both sampling and the types of analyses used (e.g., Larsson et al., 1992; Baek et al., 2011; Hao et al., 2019).
In summary, the source of bias, related to the technological advancement of the analyses of the collected samples, could have relevance in the observed variability of the historical trends of HCB and HCH (see Fig. 1a to c). In these cases, it is suggested to continue with dedicated monitoring of these POPs in the coming years to obtain robust observations and conclusions on the degradation of POPs in the Antarctic atmosphere.
In the present review, a clear trend of decreasing concentrations of PCBs and most targeted OCPs in the Antarctic atmosphere from 1980 to 2021 is documented. This is in response to the hypothesis raised historically about the decrease in atmospheric levels of historical POPs (Vecchiato et al., 2015). However, it opens the door to study new families of pollutants for which there is already analytical capacity that was not available in previous decades. In the case of HCH isomers, DDT, and PCB congeners, high atmospheric concentrations were reported for the 1990–1999 decade, but these compounds were highly restricted since the 1970s. After that date, a strong decrease was observed in the Antarctic atmosphere, which shows that the Stockholm Convention ban on POPs did have the intended impact on the (atmospheric) concentrations over time. However, these compounds are still ubiquitous in the Antarctic atmosphere with atmospheric half-lives of more than 3 years. On the other hand, the revised atmospheric levels of HCB show a decrease in the decade of its prohibition (1990). However, from the year 2000 onwards, they show strong fluctuations in the literature, with values even higher than those reported in 1990. It is noteworthy to consider that a decrease of the atmospheric concentrations does not imply a decrease of the total POPs in Antarctica, an issue that will require future work. In fact, the re-emission of HCB and other POPs from environmental surfaces, such as water, soil, and snow, is a product of its high stability in the environment that has not been deeply studied and could represent a potential source of bias for future works. Studies to date in Antarctica do not allow conclusions to be drawn about the influence of temperature on the environmental fate of POPs on the Antarctic continent. This is due to the lack of consistent time series data as historically conducted in the Arctic. Moreover, our results point to the importance of periodic monitoring and the need to establish monitoring networks with continuous sampling campaigns, not only with aim of monitoring the legacy of POPs but also to identify new pollutants that have the potential to reach Antarctica (e.g., new flame retardants, per- and polyfluoroalkyl substances (PFAS), and polycyclic aromatic hydrocarbons (PAHs), among others). There is increasing evidence of the presence of emerging compounds in different environmental matrices in Antarctica. However, the current surveillance of atmospheric pollutants is related to specific research groups, instead of coordinated efforts between countries with Antarctic presence, where continuous monitoring networks could be generated with the inclusion of various persistent toxic chemicals, as analogous to the efforts done by the Arctic Monitoring and Assessment Program (AMAP) or the Integrated Atmospheric Deposition Network (IADN) in the Great Lakes. In this sense, to establish a monitoring program for assessment of POP levels in the Antarctic atmosphere will depend on the capabilities and the facilities, since an active sampling strategy will benefit from a higher resolution in the assessment of POP trends in the monitoring points, but on the other hand, the use of passive sampling strategy could represent a high spatial coverage to monitor trends but lower time resolution. However, there is need to establish a bigger coordinated monitoring network in the future following the previous experience gained from AMAP.
All the data used in the present study are reported in the Supplement.
The supplement related to this article is available online at: https://doi.org/10.5194/acp-23-8103-2023-supplement.
TL and CJGM designed the research, retrieved the data, performed the analysis, and drafted the original version of the paper. VAGA, IPC, ECN, NH, MMM, CE, GA, APP, RL, PBN, JD, SBN, GC, and KP participated in the writing of the submitted version of the paper, commenting during the whole process. All the authors participated in the writing of the final version of the paper.
The contact author has declared that none of the authors has any competing interests.
Publisher's note: Copernicus Publications remains neutral with regard to jurisdictional claims in published maps and institutional affiliations.
This study was funded by ANID/FONDECYT/Iniciación 11150548, ANID/FONDECYT/Regular 1161504, ANID/FONDECYT/Regular 1210946, ANID/PCI REDI170292, ANID-PIA-INACH-ACT192057, INACH REGULAR RT_12_17 (Cristóbal Galbán-Malagón). Thais Luarte acknowledges the funding of the Universidad Andrés Bello office of Doctorate Program Support through the PhD Grant Program and INACH DG_02_21.
Cristobal J. Galbán-Malagón received funding from ANID through the ANID grants FONDECYT 11150548, 1210946, ANID-PCI-REDI170292, ANID-PIA Anillo-INACH-ACT192057, and INACH grant INACH-REGULAR RT_12_17. Thais Luarte's PhD grant was provided by the “Vicerrectoria de Investigación, Universidad Andrés Bello” and INACH grant DG_02_21. Claudia Egas is funded by the INACh grant DG_03_21. Victoria A. Gómez-Aburto is funded by ANID through the grant ANID FONDECYT POSTDOC 3230076.
This paper was edited by Ralf Ebinghaus and reviewed by two anonymous referees.
Atkinson, R.: Kinetics and mechanisms of the gas-phase reactions of the hydroxyl radical with organic compounds under atmospheric conditions, Chem. Rev., 86, 69–201, 1986.
Avila, B. S., Mendoza, D. P., Ramírez, A., and Peñuela, G. A.: Occurrence and Distribution of Persistent Organic Pollutants (POPs) in the Atmosphere of the Andean City of Medellin, Colombia, Chemosphere, 307, 135648, https://doi.org/10.1016/j.chemosphere.2022.135648, 2021.
Azcune, G., Griffero, L., Pareja, L., Ríos, J. M., Galbán-Malagón, C., and Pérez-Parada, A.: Trends in the monitoring of legacy and emerging organic pollutants in protected areas, Trends Environ. Anal. Chem., 34, e00165, https://doi.org/10.1016/j.teac.2022.e00165, 2022.
Baek, S. Y., Choi, S. D., and Chang, Y. S.: Three-year atmospheric monitoring of organochlorine pesticides and polychlorinated biphenyls in polar regions and the South Pacific, Environ. Sci. Technol., 45, 4475–4482, https://doi.org/10.1021/es1042996, 2011.
Barber, J. L., Sweetman, A. J., Thomas, G. O., Braekevelt, E., Stern, G. A., and Jones, K. C.: Spatial and temporal variability in air concentrations of short-chain (C10–C13) and medium-chain (C14–C17) chlorinated n-alkanes measured in the U.K. atmosphere, Environ. Sci. Technol., 39, 4407–4415, https://doi.org/10.1021/es047949w, 2005.
Bengtson-Nash, S.: Persistent organic pollutants in Antarctica: current and future research priorities, J. Environ. Monit., 13, 497–504, https://doi.org/10.1039/C0EM00230E, 2011.
Bengtson-Nash, S. M., Wild, S. J., Hawker, D. W., Cropp, R. A., Hung, H., Wania, F., Xiao, H., Bohlin-Nizzetto, P., Bignert, A., and Broomhall, S.: Persistent organic pollutants in the East Antarctic atmosphere: inter-annual observations from 2010 to 2015 using high-flow-through passive sampling, Environ. Sci. Technol., 51, 13929–13937, https://doi.org/10.1021/acs.est.7b04224, 2017.
Bidleman, T. F., Walla, M. D., Roura, R., Carr, E., and Schmidt, S.: Organochlorine pesticides in the atmosphere of the Southern Ocean and Antarctica, January–March 1990, Mar. Pollut. Bull., 26, 258–262, https://doi.org/10.1016/0025-326X(93)90064-Q, 1993.
Bigot, M., Muir, D. C., Hawker, D. W., Cropp, R., Dachs, J., Teixeira, C. F., and Bengtson Nash, S.: Air–seawater exchange of organochlorine pesticides in the Southern Ocean between Australia and Antarctica, Environ. Sci. Technol., 50, 8001–8009, https://doi.org/10.1021/acs.est.6b01970, 2016.
Blais, J. M., Macdonald, R. W., Mackay, D., Webster, E., Harvey, C., and Smol, J. P.: Biologically mediated transport of contaminants to aquatic systems, Environ. Sci. Technol., 41, 1075–1084, https://doi.org/10.1021/es061314a, 2007.
Borgå, K. and Di Guardo, A.: Comparing measured and predicted PCB concentrations in Arctic seawater and marine biota, Sci. Total Environ., 342, 281–300, https://doi.org/10.1016/j.scitotenv.2004.12.043, 2005.
Bourgeon, S., Leat, E. H., Magnusdottir, E., Fisk, A. T., Furness, R. W., Strøm, H., and Gabrielsen, G. W.: Individual variation in biomarkers of health: influence of persistent organic pollutants in Great skuas (Stercorarius skua) breeding at different geographical locations, Environ. Res., 118, 31–39, https://doi.org/10.1016/j.envres.2012.08.004, 2012.
Braune, B. M., Outridge, P. M., Fisk, A. T., Muir, D. C. G., Helm, P. A., Hobbs, K., Hoekstra, P. F., Kuzyk, Z. A., Kwan, M., Letcher, R. J. Lockhart, W. L., Norstrom, R. J., Stern, G. A., and Stirling, I.: Persistent organic pollutants and mercury in marine biota of the Canadian Arctic: an overview of spatial and temporal trends, Sci. Total Environ., 351, 4–56, https://doi.org/10.1016/j.scitotenv.2004.10.034, 2005.
Brown, F. R., Whitehead, T. P., Park, J. S., Metayer, C., and Petreas, M. X.: Levels of non-polybrominated diphenyl ether brominated flame retardants in residential house dust samples and fire station dust samples in California, Environ. Res., 135, 9–14, https://doi.org/10.1016/j.envres.2014.08.022, 2014.
Brown, T. N. and Wania, F.: Screening chemicals for the potential to be persistent organic pollutants: A case study of Arctic contaminants, Environ. Sci. Technol., 42, 5202–5209, https://doi.org/10.1021/es8004514, 2008.
Cabrerizo, A., Dachs, J., Barceló, D., and Jones, K. C.: Cclimatic and biogeochemical controls on the remobilization and reservoirs of persistent organic pollutants in Antarctica, Environ. Sci. Technol., 47, 4299–4306, https://doi.org/10.1021/es400471c, 2013.
Cabrerizo, A., Larramendi, R., Albar, J. P., and Dachs, J.: Persistent organic pollutants in the atmosphere of the Antarctic Plateau, Atmos. Environ., 149, 104–108, https://doi.org/10.1016/j.atmosenv.2016.11.015, 2017.
Casal, P., Casas, G., Vila-Costa, M., Cabrerizo, A., Pizarro, M., Jimenez, B., and Dachs, J.: Snow amplification of persistent organic pollutants at coastal Antarctica, Environ. Sci. Technol., 53, 8872–8882, 2019.
Casas, G., Martinez-Varela, A., Vila-Costa, M., Jiménez, B., and Dachs, J.: Rain amplification of persistent organic pollutants, Environ. Sci. Technol., 55, 12961–12972, https://doi.org/10.1021/acs.est.1c03295, 2021.
Channa, K. R., Röllin, H. B., Wilson, K. S., Nøst, T. H., Odland, J. Ø., Naik, I., and Sandanger, T. M.: Regional variation in pesticide concentrations in plasma of delivering women residing in rural Indian Ocean coastal regions of South Africa, J. Environ Monit., 14, 2952–2960, https://doi.org/10.1039/C2EM30264K, 2012.
Cincinelli, A., Martellini, T., Del Bubba, M., Lepri, L., Corsolini, S., Borghesi, N., King, M. D., and Dickhut, R. M.: Organochlorine pesticide air–water exchange and bioconcentration in krill in the Ross Sea, Environ. Pollut., 157, 2153–2158, https://doi.org/10.1016/j.envpol.2009.02.010, 2009.
Dachs, J., Lohmann, R., Ockenden, W. A., Méjanelle, L., Eisenreich, S. J., and Jones, K. C.: Oceanic biogeochemical controls on global dynamics of persistent organic pollutants, Environ. Sci. Technol., 36, 4229–4237, https://doi.org/10.1021/es025724k, 2002.
De March, B. G. E., De Wit, C. A., Muir, D. C. G., Braune, B. M., Gregor, D. J., Norstrom, R. J., and Stange, K.: Persistent organic pollutants, in: AMAP assessment report: Arctic pollution issues, 183–371, Oslo, Norway, ISBN 82-7655-061-4, 1998.
Dickhut, R. M., Cincinelli, A., Cochran, M., and Ducklow, H. W.: Atmospheric concentrations and air–water flux of organochlorine pesticides along the Western Antarctic Peninsula, Environ. Sci. Technol., 39, 465–470, https://doi.org/10.1021/es048648p, 2005.
Die, Q., Lu, A., Li, C., Li, H., Kong, H., and Li, B.: Occurrence of dioxin-like POPs in soils from urban green space in a metropolis, North China: implication to human exposure, Environ. Sci. Pollut. Res., 28, 5587–5597, https://doi.org/10.1007/s11356-020-10953-3, 2021.10.1007/s11356-020-10953-3, 2021.
FAO/UNEP: Polychlorinated biphenyls, in: Operation of the prior informed consent procedure for banned or severely restricted chemicals in international trade: decision guidance documents, FAO/United Nations, Rome-Geneva, 11–16, 1992.
Fisk, A. T., Hobson, K. A., and Norstrom, R. J.: Influence of chemical and biological factors on trophic transfer of persistent organic pollutants in the Northwater Polynya marine food web, Environ. Sci. Technol., 35, 732–738, https://doi.org/10.1021/es001459w, 2001a.
Fisk, A. T., Stern, G. A., Hobson, K. A., Strachan, W. J., Loewen, M. D., and Norstrom, R. J.: Persistent organic pollutants (POPs) in a small, herbivorous, Arctic marine zooplankton (Calanus hyperboreus): trends from April to July and the influence of lipids and trophic transfer, Mar. Pollut. Bull., 43, 93–101, https://doi.org/10.1016/S0025-326X(01)00038-8, 2001b.
Fuoco, R., Colombini, M. P., Abete, C., and Carignani, S.: Polychlorobiphenyls in sediment, soil and sea water samples from Antarctica, Int. J. Environ. Anal. Chem., 61, 309–318, https://doi.org/10.1080/03067319508027246, 1995.
Galbán-Malagón, C., Berrojalbiz, N., Ojeda, M. J., and Dachs, J.: The oceanic biological pump modulates the atmospheric transport of persistent organic pollutants to the Arctic, Nat. Commun., 3, 862, https://doi.org/10.1038/ncomms1858, 2012.
Galbán-Malagón, C. J., Del Vento, S., Berrojalbiz, N., Ojeda, M. J., and Dachs, J.: Polychlorinated biphenyls, hexachlorocyclohexanes and hexachlorobenzene in seawater and phytoplankton from the Southern Ocean (Weddell, South Scotia, and Bellingshausen Seas), Environ. Sci. Technol., 47, 5578–5587, https://doi.org/10.1021/es400030q, 2013a.
Galbán-Malagón, C., Cabrerizo, A., Caballero, G., and Dachs, J.: Atmospheric occurrence and deposition of hexachlorobenzene and hexachlorocyclohexanes in the Southern Ocean and Antarctic Peninsula, Atmos. Environ., 80, 41–49, https://doi.org/10.1016/j.atmosenv.2013.07.061, 2013b.
Galbán-Malagón, C. J., Del Vento, S., Cabrerizo, A., and Dachs, J.: Factors affecting the atmospheric occurrence and deposition of polychlorinated biphenyls in the Southern Ocean, Atmos. Chem. Phys., 13, 12029–12041, https://doi.org/10.5194/acp-13-12029-2013, 2013c.
Galbán-Malagón, C. J., Berrojalbiz, N., Gioia, R., and Dachs, J.: The “Degradative” and “Biological” Pumps Controls on the Atmospheric Deposition and Sequestration of Hexachlorocyclohexanes and Hexachlorobenzene in the North Atlantic and Arctic Oceans, Environ. Sci. Technol., 47, 7195–7203, https://doi.org/10.1021/es4011256, 2013d.
Galbán-Malagón, C. J., Hernán, G., Abad, E., and Dachs, J.: Persistent organic pollutants in krill from the Bellingshausen, South Scotia, and Weddell Seas, Sci. Total. Environ., 610–611, 1487–1495, https://doi.org/10.1016/j.scitotenv.2017.08.108, 2018.
Gambaro, A., Manodori, L., Zangrando, R., Cincinelli, A., Capodaglio, G., and Cescon, P.: Atmospheric PCB concentrations at Terra Nova Bay, Antarctica, Environ. Sci. Technol., 39, 9406–9411, https://doi.org/10.1021/es0510921, 2005.
Garcia-Cegarra, A. M., Jung, J. L., Orrego, R., Padilha, J. D. A., Malm, O., Ferreira-Braz, B., Santelli, R. E., Pozo. K., Pribylova, P., Alvarado-Rybak, M., Azat, C., Kidd, K. A., Espejo, W., Chiang, G., and Bahamonde, P.: Persistence, bioaccumulation and vertical transfer of pollutants in long-finned pilot whales stranded in Chilean Patagonia, Sci. Total Environ., 770, 145259, https://doi.org/10.1016/j.scitotenv.2021.145259, 2021.
Gioia, R., Lohmann, R., Dachs, J., Temme, C., Lakaschus, S., Schulz-Bull, D., and Jones, K. C.: Polychlorinated biphenyls in air and water of the North Atlantic and Arctic Ocean, J. Geophys. Res.-Atmos., 113, D19302, https://doi.org/10.1029/2007JD009750, 2008.
Gupta, R. S., Sarkar, A., and Kureishey, T. W.: PCBs and organochlorine pesticides in krill, birds and water from Antartica, Deep-Sea Res. Pt. II, 43, 119–126, https://doi.org/10.1016/0967-0645(95)00086-0, 1996.
Hale, R. C., Kim, S. L., Harvey, E., La Guardia, M. J., Mainor, T. M., Bush, E. O., and Jacobs, E. M.: Antarctic research bases: local sources of polybrominated diphenyl ether (PBDE) flame retardants, Environ. Sci. Technol., 42, 1452–1457, https://doi.org/10.1021/es702547a, 2008.
Hao, Y., Li, Y., Han, X., Wang, T., Yang, R., Wang, P., Xiao, K., Li, W., Lu, H., H., Fu, J., Wang, Y., Shi, J., Zhang, Q., Jiang, G.: Air monitoring of polychlorinated biphenyls, polybrominated diphenyl ethers and organochlorine pesticides in West Antarctica during 2011–2017: Concentrations, temporal trends and potential sources, Environ. Pollut., 249, 381–389, https://doi.org/10.1016/j.envpol.2019.03.039, 2019.
Hop, H., Borgå, K., Gabrielsen, G. W., Kleivane, L., and Skaare, J. U.: Food web magnification of persistent organic pollutants in poikilotherms and homeotherms from the Barents Sea, Environ. Sci. Technol., 36, 2589–2597, https://doi.org/10.1021/es010231l, 2002.
Harner, T., Jantunen, L. M., Bidleman, T. F., Barrie, L. A., Kylin, H., Strachan, W. M., and Macdonald, R. W.: Microbial degradation is a key elimination pathway of hexachlorocyclohexanes from the Arctic Ocean, Geophys. Res. Lett., 27, 1155–1158, https://doi.org/10.1029/1999GL011326, 2000.
Helm, P. A., Diamond, M. L., Semkin, R., Strachan, W. M., Teixeira, C., and Gregor, D.: A mass balance model describing multiyear fate of organochlorine compounds in a high Arctic Lake, Environ. Sci. Technol., 36, 996–1003, https://doi.org/10.1021/es010952k, 2002.
Howard, P. H. (Ed.): Handbook of Environmental Fate and Exposure Data: For Organic Chemicals, Volume III Pesticides, 1st edn., Routledge, https://doi.org/10.1201/9780203719305, 1991.
Huang, Y., Xu, Y., Li, J., Xu, W., Zhang, G., Cheng, Z., Liu, J., Wang, Y., and Tian, C.: Organochlorine pesticides in the atmosphere and surface water from the equatorial Indian Ocean: enantiomeric signatures, sources, and fate, Environ. Sci. Technol., 47, 13395–13403, https://doi.org/10.1021/es403138p, 2013.
Hung, H., Kallenborn, R., Breivik, K., Su, Y., Brorström-Lundén, E., Olafsdottir, K., and Fellin, P.: Atmospheric monitoring of organic pollutants in the Arctic under the Arctic Monitoring and Assessment Programme (AMAP): 1993–2006, Sci. Total Environ., 408, 2854–2873, https://doi.org/10.1016/j.scitotenv.2009.10.044, 2010.
Hung, H., Katsoyiannis, A. A., Brorström-Lundén, E., Olafsdottir, K., Aas, W., Breivik, K., and Wilson, S.:Temporal trends of Persistent Organic Pollutants (POPs) in arctic air: 20 years of monitoring under the Arctic Monitoring and Assessment Programme (AMAP), Environ, Poll., 217, 52–61, https://doi.org/10.1016/j.envpol.2016.01.079, 2016.
Hung, H., Halsall, C., Ball, H., Bidleman, T. F., Dachs, J., De Silva, A., Hermanson, M., Kallenborn, R., Muir, D., Suhring, R., Wang, X., and Wilson, S.: Climate change influence on the levels and trends of persistent organic pollutants (POPs) and chemicals of emerging Arctic concern (CEACs) in the Arctic physical environment – a review, Environ. Sci. Process. Impacts, 24, 1577–1615, https://doi.org/10.1039/D1EM00485A, 2022.
Jantunen, L. M., Kylin, H., Bidleman, T. F.: Air–water gas exchange of α-hexachlorocyclohexane enantiomers in the South Atlantic Ocean and Antarctica, Deep-Sea Res. Pt., 51, 2661–2672, https://doi.org/10.1016/j.dsr2.2004.02.002, 2004.
Jayaraj, R., Megha, P., and Sreedev, P.: Organochlorine pesticides, their toxic effects on living organisms and their fate in the environment, Interdiscip. Toxicol., 9, 90–100, https://doi.org/10.1515/intox-2016-0012, 2016.
Jurado, E. and Dachs, J.: Seasonality in the “grasshopping” and atmospheric residence times of persistent organic pollutants over the oceans, Geophy. Res. Lett., 35, L17805, https://doi.org/10.1029/2008GL034698, 2008.
Kallenborn, R., Breivik, K., Eckhardt, S., Lunder, C. R., Manø, S., Schlabach, M., and Stohl, A.: Long-term monitoring of persistent organic pollutants (POPs) at the Norwegian Troll station in Dronning Maud Land, Antarctica, Atmos. Chem. Phys., 13, 6983–6992, https://doi.org/10.5194/acp-13-6983-2013, 2013.
Kallenborn, R., Oehme, M., Wynn-Williams, D. D., Schlabach, M., and Harris, J.: Ambient air levels and atmospheric long-range transport of persistent organochlorines to Signy Island, Antarctica, Sci. Total Environ., 220, 167–180, https://doi.org/10.1016/S0048-9697(98)00257-5, 1998.
Kelly, T. J., Mukund, R., Spicer, C. W., and Pollack, A. J.: Concentrations and transformations of hazardous air pollutants, Environ. Sci. Technol., 28, 378A–387A, https://doi.org/10.1021/es00057a003, 1994.
Kennish, M. J.: Practical Handbook of Estuarine and Marine Pollution, 1st edn., CRC Press, https://doi.org/10.1201/9780203742488, 2017.
Khairy, M. A., Luek, J. L., Dickhut, R., and Lohmann, R.: Levels, sources and chemical fate of persistent organic pollutants in the atmosphere and snow along the western Antarctic Peninsula, Environ. Pollut., 216, 304–313, https://doi.org/10.1016/j.envpol.2016.05.092, 2016.
Kim, J. T., Son, M. H., Kang, J. H., Kim, J. H., Jung, J. W., and Chang, Y. S.: Occurrence of legacy and new persistent organic pollutants in avian tissues from King George Island, Antarctica, Environ. Sci. Technol., 49, 13628–13638, https://doi.org/10.1021/acs.est.5b03181, 2015.
Klánová, J., Matykiewiczová, N., Máčka, Z., Prošek, P., Láska, K., and Klán, P.: Persistent organic pollutants in soils and sediments from James Ross Island, Antarctica, Environ. Pollut., 152, 416–423, https://doi.org/10.1016/j.envpol.2007.06.026, 2008.
Kong, D., MacLeod, M., Hung, H., and Cousins, I. T.: Statistical analysis of long-term monitoring data for persistent organic pollutants in the atmosphere at 20 monitoring stations broadly indicates declining concentrations, Environ. Sci. Technol., 48, 12492–12499, https://doi.org/10.1021/es502909n, 2014.
Krasnobaev, A., Ten Dam, G., Boerrigter-Eenling, R., Peng, F., van Leeuwen, S. P., Morley, S. A., Peck, L. S., and Van Den Brink, N. W.: Legacy and emerging persistent organic pollutants in Antarctic benthic invertebrates near Rothera Point, Western Antarctic Peninsula, Environ. Sci. Technol., 54, 2763–2771, https://doi.org/10.1021/acs.est.9b06622, 2020.
Lakaschus, S., Weber, K., Wania, F., Bruhn, R., and Schrems, O.: The air–sea equilibrium and time trend of hexachlorocyclohexanes in the Atlantic Ocean between the Arctic and Antarctica, Environ. Sci. Technol., 36, 138–145, https://doi.org/10.1021/es010211j, 2002.
Larsson, P., Järnmark, C., and Södergren, A.: PCBs and chlorinated pesticides in the atmosphere and aquatic organisms of Ross Island, Antarctica, Mar. Pollut. Bull., 25, 281–287, https://doi.org/10.1016/0025-326X(92)90683-W, 1992.
Larsson, P., Andersson, A., Broman, D., Nordbäck, J., and Lundberg, E.: Persistent organic pollutants (POPs) in pelagic systems, AMBIO, 29, 202–209, https://doi.org/10.1579/0044-7447-29.4.202, 2000.
Li, Y., Geng, D., Hu, Y., Wang, P., Zhang, Q., and Jiang, G.: Levels and distribution of polychlorinated biphenyls in the atmosphere close to Chinese Great Wall Station, Antarctica: Results from XAD-resin passive air sampling, Chinese Sci. Bull., 57, 1499–1503, https://doi.org/10.1007/s11434-012-5048-8, 2012a.
Li, Y., Geng, D., Liu, F., Wang, T., Wang, P., Zhang, Q., and Jiang, G.: Study of PCBs and PBDEs in King George Island, Antarctica, using PUF passive air sampling, Atmos. Environ., 51, 140–145, https://doi.org/10.1016/j.atmosenv.2012.01.034, 2012b.
Li, Y., Lohmann, R., Zou, X., Wang, C., and Zhang, L.: Air-water exchange and distribution pattern of organochlorine pesticides in the atmosphere and surface water of the open Pacific Ocean, Environ. Pollut., 265, 114956, https://doi.org/10.1016/j.envpol.2020.114956, 2020.
Li, Y. F.: Global gridded technical hexachlorocyclohexane usage inventories using a global cropland as a surrogate, J. Geophys. Res., 104, 23785–23797, https://doi.org/10.1029/1999JD900448, 1999.
Lohmann, R., Breivik, K., Dachs, J., and Muir, D.: Global fate of POPs: current and future research directions, Environ. Pollut., 150, 150–165, https://doi.org/10.1016/j.envpol.2007.06.051, 2007.
Lohmann, R., Gioia, R., Jones, K. C., Nizzetto, L., Temme, C., Xie, Z., Schulz-Bull, D., Hand, I., Morgan, E., and Jantunen, L.: Organochlorine pesticides and PAHs in the surface water and atmosphere of the North Atlantic and Arctic Ocean, Environ. Sci. Technol., 43, 5633–5639, https://doi.org/10.1021/es901229k, 2009.
Lozoya, J. P., Rodríguez, M., Azcune, G., Lacerot, G., Pérez-Parada, A., Lenzi, J., Rossi, F., and de Mello, F. T.: Stranded pellets in Fildes Peninsula (King George Island, Antarctica): New evidence of Southern Ocean connectivity, Sci. Total Environ., 838, 155830, https://doi.org/10.1016/j.scitotenv.2022.155830, 2022.
Ma, J., Hung, H., Tian, C., and Kallenborn, R.: Revolatilization of persistent organic pollutants in the Arctic induced by climate change, Nat. Clim. Change, 1, 255–260, https://doi.org/10.1038/nclimate1167, 2011.
Mackay, D. and Wania, F.: Transport of contaminants to the Arctic: partitioning, processes and models, Sci. Total Environ., 160, 25–38, https://doi.org/10.1016/0048-9697(95)04342-X, 1995.
Montone, R. C., Taniguchi, S., and Weber, R. R.: PCBs in the atmosphere of King George Island, Antarctica, Sci. Total Environ., 308, 167–173, https://doi.org/10.1016/S0048-9697(02)00649-6, 2003.
Montone, R. C., Taniguchi, S., Boian, C., and Weber, R. R.: PCBs and chlorinated pesticides (DDTs, HCHs and HCB) in the atmosphere of the southwest Atlantic and Antarctic oceans, Mar. Pollut. Bull., 50, 778–782, https://doi.org/10.1016/j.marpolbul.2005.03.002, 2005.
Montone, R. C., Alvarez, C. E., Bícego, M. C., Braga, E. S., Brito, T. A., Campos, L. S. ..., and Weber, R. R.: Environmental Assessment of Admiralty Bay, King George Island, Antarctica, in: Adaptation and Evolution in Marine Environments, Volume 2, 157–175, https://doi.org/10.1007/978-3-642-27349-0_9, 2013.
Mortimer, M. R. and Connell, D. W.: A model of the environmental fate of chlorohydrocarbon contaminants associated with Sydney sewage discharges, Chemosphere, 30, 2021–2038, https://doi.org/10.1016/0045-6535(95)00081-I, 1995.
Nizzetto, L., Lohmann, R., Gioia, R., Dachs, J., and Jones, K. C.: Atlantic Ocean surface waters buffer declining atmospheric concentrations of persistent organic pollutants, Environ. Sci. Technol., 44, 6978–6984, https://doi.org/10.1021/es101293v, 2010.
Pennington, D. W.: An evaluation of chemical persistence screening approaches, Chemosphere, 44, 1589–1601, https://doi.org/10.1016/S0045-6535(00)00530-0, 2001.
Potapowicz, J., Szumińska, D., Szopińska, M., and Polkowska, Ż.: The influence of global climate change on the environmental fate of anthropogenic pollution released from the permafrost: Part I. Case study of Antarctica, Sci. Total Environ., 651, 1534–1548, https://doi.org/10.1016/j.scitotenv.2018.09.168, 2019.
Pozo, K., Martellini, T., Corsolini, S., Harner, T., Estellano, V., Kukučka, P., and Cincinelli, A.: Persistent organic pollutants (POPs) in the atmosphere of coastal areas of the Ross Sea, Antarctica: Indications for long-term downward trends, Chemosphere, 178, 458–465, https://doi.org/10.1016/j.chemosphere.2017.02.118, 2017.
Prats, R. M., Van Drooge, B. L., Fernández, P., and Grimalt, J. O.: Field comparison of passive polyurethane foam and active air sampling techniques for analysis of gas-phase semi-volatile organic compounds at a remote high-mountain site, Sci. Total Environ., 803, 149738, https://doi.org/10.1016/j.scitotenv.2021.149738, 2022.
Qiu, X., Zhu, T., Li, J., Pan, H., Li, Q., Miao, G., and Gong, J.: Organochlorine pesticides in the air around the Taihu Lake, China, Environ. Sci. Technol., 38, 1368–1374, https://doi.org/10.1021/es035052d, 2004.
R Core Team: R: A Language and Environment for Statistical Computing, R Foundation for Statistical Computing, Vienna, Austria, https://www.R-project.org/, last access: 31 December 2022.
Risebrough, R. W., Rieche, P., Peakall, D. B., Herman, S. T., and Kirven, M. N.: Polychlorinated biphenyls in the global ecosystem, Nature, 220, 1098–1102, https://doi.org/10.1038/2201098a0, 1968.
Risebrough, R. W., Walker, W., Schmidt, T. T., De Lappe, B. W., and Connors, C. W.: Transfer of chlorinated biphenyls to Antarctica, Nature, 264, 738–739, https://doi.org/10.1038/264738a0, 1976.
Risebrough, R. W., De Lappe, B. W., and Younghans-Haug, C.: PCB and PCT contamination in Winter Quarters Bay, Antarctica, Mar. Pollut. Bull., 21, 523–529, https://doi.org/10.1016/0025-326X(90)90300-W, 1990.
Safe, S. H.: Polychlorinated biphenyls (PCBs): environmental impact, biochemical and toxic responses, and implications for risk assessment, Crit. Rev. Toxicol., 24, 87–149, https://doi.org/10.3109/10408449409049308, 1994.
Sinkkonen, S. and Paasivirta, J.: Degradation half-life times of PCDDs, PCDFs and PCBs for environmental fate modeling, Chemosphere, 40, 943–949, https://doi.org/10.1016/S0045-6535(99)00337-9, 2000.
Sladen, W. J., Menzie, C. M., and Reichel, W. L.: DDT residues in Adelie penguins and a crabeater seal from Antarctica, Nature, 210, 670–673, https://doi.org/10.1038/210670a0, 1966.
Tanabe, S., Kawano, M., and Tatsukawa, R.: Chlorinated hydrocarbons in the Antarctic, western Pacific and eastern Indian Oceans, Trans. Tokyo Univ. Fish, 5, 97–109, 1982.
Tanabe, S., Hidaka, H., and Tatsukawa, R.: PCBs and chlorinated hydrocarbon pesticides in Antarctic atmosphere and hydrosphere, Chemosphere, 12, 277–288, https://doi.org/10.1016/0045-6535(83)90171-6, 1983.
Tatton, J. G. and Ruzicka, J. H. A.: Organochlorine pesticides in Antarctica, Nature, 215, 346–348, https://doi.org/10.1038/215346a0, 1967.
United Nations Environment Program (UNEP): Final Act of the Conference of Plenipotentiaries on the Stockholm Convention on Persistent Organic Pollutants, Geneva, Switzerland, 2001.
United Nations Economic Commission for Europe (UNECE): Aarhus Convention on Access to Information, Public Participation in Decision-making and Access to Justice in Environmental Matters, 2256 UNTS 119, 40 ILM 532, 1999.
Venier, M. and Hites, R. A.: Regression model of partial pressures of PCBs, PAHs, and organochlorine pesticides in the Great Lakes’ atmosphere, Environ. Sci. Technol., 44, 618–623, https://doi.org/10.1021/es902804s, 2010.
Vergara, E., Hernández, V., Barra, R., Munkittrick, K. R., Galbán-Malagón, C., and Chiang, G.: Presence of Organochlorine pollutants in fat and scats of pinnipeds from the Antarctic Peninsula and South Shetland Islands, and their relationship to trophic position, Sci. Total Environ., 685, 1276–1283, https://doi.org/10.1016/j.scitotenv.2019.06.122, 2019.
Vasseghian, Y., Hosseinzadeh, S., Khataee, A., and Dragoi, E. N.: The concentration of persistent organic pollutants in water resources: A global systematic review, meta-analysis and probabilistic risk assessment, Sci. Total Environ., 796, 149000, https://doi.org/10.1016/j.scitotenv.2021.149000, 2021.
Vaughan, D. G., Marshall, G. J., Connolley, W. M., Parkinson, C., Mulvaney, R., Hodgson, D. A., King, J. C., Pudsey, C. J., and Turner, J.: Recent rapid regional climate warming on the Antarctic Peninsula, Climatic Change, 60, 243–274, https://doi.org/10.1023/A:1026021217991, 2003.
Vijgen, J.: The legacy of Lindane HCH Isomer production in A global overview of Residue management Formulation and Disposal, Internation HCH and pesticides Association, ISBN 87-991210-1-8, http://www.ihpa.info/docs/library/reports/Lindane Main Report DEF20JAN06.pdf (last access: 10 March 2023), 2006.
Voldner, E. C. and Li, Y. F.: Global usage of selected persistent organochlorines, Sci. Total Environ., 160, 201–210, https://doi.org/10.1016/0048-9697(95)04357-7, 1995.
Von Waldow, H., MacLeod, M., Scheringer, M., and Hungerbühler, K.: Quantifying remoteness from emission sources of persistent organic pollutants on a global scale, Environ. Sci. Technol., 44, 2791–2796, https://doi.org/10.1021/es9030694, 2010.
Vorkamp, K., Carlsson, P., Corsolini, S., de Wit, C. A., Dietz, R., Gribble, M. O., Houde, M., Kalia, V., Letcher, R. J., Morris, A., Rigét, F. F., Routti, H., and Muir, D. C.: Influences of climate change on long-term time series of persistent organic pollutants (POPs) in Arctic and Antarctic biota, Environ. Sci.-Proc. Imp., 24, 1643–1660, https://doi.org/10.1039/D2EM00134A, 2022.
Wang, P., Zhang, Q., Li, Y., Zhu, Ch., Chen, Z., Zheng, S., Sun, H., Liang, L., and Jiang, G.: Occurrence of chiral organochlorine compounds in the environmental matrices from King George Island and Ardley Island, west Antarctica, Scientific Reports, 5, 1–9, https://doi.org/10.1038/srep13913, 2015.
Wang, P., Li, Y., Zhang, Q., Yang, Q., Zhang, L., Liu, F., Fu, J., Meng, W., Wang, d., Sun, H., Zheng, S., Hao, Y., Liang, Y., and Jiang, G.: Three-year monitoring of atmospheric PCBs and PBDEs at the Chinese Great Wall Station, West Antarctica: levels, chiral signature, environmental behaviors and source implication, Atmos. Environ., 150, 407–416, https://doi.org/10.1016/j.atmosenv.2016.11.036, 2017.
Wang, P., Meng, W., Li, Y., Zhang, Q., Zhang, L., Fu, J., Yang, R., and Jiang, G.: Temporal variation (2011–2014) of atmospheric OCPs at King George Island, west Antarctica, Atmos. Environ., 191, 432–439, https://doi.org/10.1016/j.atmosenv.2018.08.036, 2018.
Wania, F. and Mackay, D.: Peer reviewed: tracking the distribution of persistent organic pollutants, Environ. Sci. Technol., 30, 390A–396A, https://doi.org/10.1021/es962399q, 1996.
Wasley, J., Robinson, S. A., Lovelock, C. E., and Popp, M.: Climate change manipulations show Antarctic flora is more strongly affected by elevated nutrients than water, Glob. Change Biol., 12, 1800–1812, https://doi.org/10.1111/j.1365-2486.2006.01209.x, 2006.
Weber, K. and Goerke, H.: Persistent organic pollutants (POPs) in Antarctic fish: levels, patterns, changes, Chemosphere, 53, 667–678, https://doi.org/10.1016/S0045-6535(03)00551-4, 2003.
Wild, S., Eulaers, I., Covaci, A., Bossi, R., Hawker, D., Cropp, R., Southwell, C., Emmerson, L., Lepoint, G., Eisenmann, P., and Bengtson-Nash, S.: South polar skua (Catharacta maccormicki) as biovectors for long-range transport of persistent organic pollutants to Antarctica, Environ. Pollut., 292, 118358, https://doi.org/10.1016/j.envpol.2021.118358, 2022.
Wong, F., Hung, H., Dryfhout-Clark, H., Aas, W., Bohlin-Nizzetto, P., Breivik, K., Nerentorp Mastromonaco, M., Brorström Lundén, E., Ólafsdóttir, K., Sigurðsson, Á., Vorkamp, K., Bossi, R., Skov, H., Hakola, H., Barresi, E., Sverko, E., Fellin, P., Li, H., Vlasenko, A., Zapevalov, M., Samsonov, D., and Wilson, S.: Time trends of persistent organic pollutants (POPs) and Chemicals of Emerging Arctic Concern (CEAC) in Arctic air from 25 years of monitoring, Sci. Total Environ., 775, 145109, https://doi.org/10.1016/j.scitotenv.2021.145109, 2021.
Wu, X., Lam, J. C., Xia, C., Kang, H., Sun, L., Xie, Z., and Lam, P. K.: Atmospheric HCH concentrations over the marine boundary layer from Shanghai, China to the Arctic Ocean: Role of human activity and climate change, Environ. Sci. Technol., 44, 8422–8428, https://doi.org/10.1021/es102127h, 2010.
Wu, X., Lam, J. C., Xia, C., Kang, H., Xie, Z., and Lam, P. K.: Atmospheric concentrations of DDTs and chlordanes measured from Shanghai, China to the Arctic Ocean during the Third China Arctic Research Expedition in 2008, Atmos. Environ., 45, 3750–3757, https://doi.org/10.1016/j.atmosenv.2011.04.012, 2011.
Wu, X., Chen, A., Yuan, Z., Kang, H., and Xie, Z.: Atmospheric organochlorine pesticides (OCPs) and polychlorinated biphenyls (PCBs) in the Antarctic marginal seas: Distribution, sources and transportation, Chemosphere, 258, 127359, https://doi.org/10.1016/j.chemosphere.2020.127359, 2020.
Xie, J., Tao, L., Wu, Q., Bian, Z., Wang, M., Li, Y., Zhu. G., and Lin, T.: Bioaccumulation of organochlorine pesticides in Antarctic krill (Euphausia superba): Profile, influencing factors, and mechanisms, J. Hazard. Mater., 426, 128115, https://doi.org/10.1016/j.jhazmat.2021.128115, 2022.
Xie, Z., Koch, B. P., Möller, A., Sturm, R., and Ebinghaus, R.: Transport and fate of hexachlorocyclohexanes in the oceanic air and surface seawater, Biogeosciences, 8, 2621–2633, https://doi.org/10.5194/bg-8-2621-2011, 2011.
Zhang, L., Bidleman, T., Perry, M. J., and Lohmann, R.: Fate of chiral and achiral organochlorine pesticides in the North Atlantic bloom experiment, Environ. Sci. Technol., 46, 8106–8114, https://doi.org/10.1021/es3009248.
Vecchiato, M., Argiriadis, E., Zambon, S., Barbante, C., Toscano, G., Gambaro, A., and Piazza, R.: Persistent Organic Pollutants (POPs) in Antarctica: Occurrence in continental and coastal surface snow, Microchem. J., 119, 75–82, https://doi.org/10.1016/j.microc.2014.10.010, 2015.