the Creative Commons Attribution 4.0 License.
the Creative Commons Attribution 4.0 License.
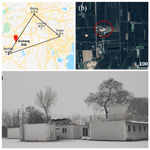
Measurement Report: Wintertime new particle formation in the rural area of the North China Plain – influencing factors and possible formation mechanism
Juan Hong
Min Tang
Qiaoqiao Wang
Nan Ma
Shaowen Zhu
Shaobin Zhang
Xihao Pan
Linhong Xie
Uwe Kuhn
Jiangchuan Tao
Yao He
Wanyun Xu
Runlong Cai
Yaqing Zhou
Zhibin Wang
Guangsheng Zhou
Yafang Cheng
The high concentration of fine particles and gaseous pollutants makes polluted areas, such as the urban setting of North China Plain (NCP) of China, a different environment for new particle formation (NPF) compared to many clean regions. Such conditions also hold for other polluted environments in this region (for instance, the rural area of NCP), yet the underlying mechanisms for NPF remain less understood, owing to the limited observations of particles in the sub−3 nm range. Comprehensive measurements, particularly covering the particle number size distribution down to 1.3 nm, were conducted at a rural background site of Gucheng (GC) in the North China Plain (NCP) from 12 November to 24 December 2018. In total, five NPF events during the 39 effective days of measurements for the campaign were identified, with the mean particle nucleation rate (J1.3) and growth rate (GR1.3–2.4) being 22.0 cm−3 s−1 and 3.9 nm h−1, respectively. During these 5 d, NPF concurrently occurred at an urban site in Beijing. Sharing similar sources and transport paths of air masses arriving at our site to that of urban Beijing, we hypothesize that NPF events during these days in this region might be a regional phenomenon. The simultaneous occurrence of NPF in both places implies that H2SO4-amine nucleation, concluded for urban Beijing there, could probably be the dominating mechanism for NPF at our rural site. The higher concentration of sulfuric acid during many non-event days compared to that of event days indicates that the content of sulfuric acid may not necessarily lead to NPF events under current atmosphere. Only when the condensation sink or coagulation sink was significantly lowered, atmospheric NPF occurred, implying that condensation sinks (CSs) and coagulation sinks (CoagSs) are the dominating factors controlling the occurrence of NPF for the present rural environment of the NCP, which is quite similar to the feature seen in urban Beijing.
- Article
(3282 KB) - Full-text XML
-
Supplement
(2870 KB) - BibTeX
- EndNote
Atmospheric new particle formation (NPF) is a major source of the global particles in terms of number concentration and size distribution (Kulmala et al., 2004) and is considered to contribute up to half of the global cloud condensation nuclei (CCN) budget in the lower troposphere (Spracklen et al., 2006; Dunne et al., 2016). In general, NPF consists of two consecutive processes, namely (a) the formation or nucleation of molecular clusters by low-volatile gaseous substances and (b) their subsequent growth to detectable sizes or even larger – at which sizes these particles may act as CCN or contribute to the particle mass concentration (Kulmala et al., 2000; Zhang et al., 2012).
Numerous laboratory measurements and field studies have shown that sulfuric acid molecules (H2SO4) are one of the key precursors to forming molecular clusters for nucleation (Nieminen et al., 2010; Sipilä et al., 2010; Kirkby et al., 2011; Riccobono et al., 2014; Stolzenburg et al., 2020). However, these H2SO4 clusters relevant to atmospheric nucleation are typically quite small (i.e., with diameters below 1.5 nm), making the detection efficiency of traditional instruments specific for NPF unsatisfactory (Kulmala, 2013). This led to large uncertainties in the measured formation rate of newly formed particles and thus precise measurements of these clusters or particles down to sub−3 nm were required. Recently, progress such as the use of a particle size magnifier (PSM; Vanhanen et al., 2011; Xiao et al., 2015), a neutral cluster and air ion spectrometer (NAIS; Mirme and Mirme, 2013), and a chemical ionization–atmospheric pressure interface time-of-flight mass spectrometer (CI-APi-TOF; Jokinen et al., 2012) have made it possible to directly measure the number concentration of clusters in the 1–3 nm size range. Benefitting from these novel techniques, observations have found that the growth of H2SO4 clusters would be significantly promoted after being stabilized by other precursors like amines, ammonia, or iodine species (Berndt et al., 2010; Kirkby et al., 2011; Almeida et al., 2013; Riccobono et al., 2014; Kürten et al., 2016; Sipilä et al., 2010). Furthermore, oxidation products from volatile organic compounds (for instance, highly oxidized organic compounds) were suggested to be important contributors to atmospheric nucleation (Ehn et al., 2014; Bianchi et al., 2016; Kirkby et al., 2016; Tröstl et al., 2016).
The North China Plain (NCP) of China has been suffering heavily from the highly complex air pollution for decades (Ma et al., 2016; Shen et al., 2018a, b; Zhang et al., 2020), owing to the high emissions or formation of different pollutants such as SO2, NH3, volatile organic compounds (VOCs), and fine particles from various sources (Guo et al., 2014; Zhang et al., 2015). Due to the high concentration of pre-existing particles, previous studies considered that, in the NCP, less NPF would occur, as the newly formed particles would be scavenged much faster before growing. By contrast, atmospheric NPF was still frequently observed in this region (Chu et al., 2019; Deng et al., 2020; Cai et al., 2021b), occurring more often than theoretically predicted (Kulmala et al., 2014) and indicating that the underlying mechanisms for NPF in this area might be different, meaning that those mechanisms previously found for other environments might not be completely applicable. The higher concentration of these gaseous precursors makes this region a unique condition for NPF compared to relatively clean environments (Kulmama et al., 2016; Yu et al., 2017; Wang et al., 2017), further supporting the hypothesis of different formation mechanisms and thereby distinct features of NPF events in this region. These doubts concerning NPF in the NCP, however, still remain to be elucidated due to limitations of comprehensive measurements, particularly for rural areas of the NCP, where observations regarding NPF were even more rare.
In addition, with respect to those existing studies concerning NPF in the NCP, they mainly focused on the measurements of particles beyond 3 nm. Without applicable instruments, observations of new particles down to sub−3 m were still quite limited (Fang et al., 2020; Zhou et al., 2020), causing large uncertainties in the measured characteristics of NPF for the current region. To fill the gap of the measurements of particles or clusters in the size range of 1–3 nm and further advance our understanding of NPF in this region, particularly in the rural area of NCP, we conducted a comprehensive measurement campaign at a rural background site in the NCP from 12 November to 24 December 2018. By obtaining the particle number size distribution over a wide diameter range (1.3 nm–10 µm), we aimed to investigate the characteristics of NPF events at the rural site in NCP during wintertime, find out which factors govern the occurring of NPF compared to other regions of NCP such as the urban areas, and explore the potential mechanisms for NPF in this area.
2.1 Field measurements site
The measurements were conducted at Gucheng (GC) site (39∘09′01.1′′ N, 115∘44′02.6′′ E), situated at an Ecological and Agricultural Meteorology Station (39∘09′ N, 115∘44′ E) of the Chinese Academy of Meteorological Sciences from 12 November to 24 December 2018. The station is located in Dingxing county, Baoding city, Hebei province, China (as seen in Fig. 1), and surrounded by agricultural fields and sporadically distributed villages. Being far from the urban and industrial emission areas, this site can be treated as a representative regional site in the northern part of NCP. More details about this site can be found in Lin et al. (2009) and Shen et al. (2018a).
2.2 Measurements
2.2.1 Particle number size distribution (PNSD) measurement
The aerosol sampling inlet was located on the rooftop of a measurement container, where room temperature was maintained at 22∘ C (Fig. 1c). The aerosol was sampled via a low-flow PM10 cyclone inlet, passed through a Nafion dryer, and directed to different instruments through stainless steel or conductive black tubing using an isokinetic flow splitter. The particle number size distribution (PNSD) of the aerosol particles with diameters from 10 to 10 000 nm was measured by using a scanning mobility particle sizer (SMPS; TSI 3938) and an aerodynamic particle sizer (APS; TSI 3321) at a time resolution of around 5 min. The SMPS consisted of an electrostatic classifier (TSI 3080) and a condensation particle counter (CPC; TSI 3772).
2.2.2 Sub−3 nm particle number concentration measurement
Sub−3 nm particles were measured with an Airmodus A11 nano condensation nucleus counter system (nCNC), consisting of a particle size magnifier (PSM; A10) and a butanol condensation particle counter (CPC; A20; Vanhanen et al., 2011). The Airmodus PSM uses diethylene glycol as the working fluid to activate and grow nano-sized particles. Specifically, the PSM was operated under the scanning mode so that the diethylene glycol flow varied between 0.1 and 1.3 L min−1. Thus, the number size distribution of five different size bins (i.e., 1.3–1.4, 1.4–1.6, 1.6–1.9, 1.9–2.4, and 2.4–3.7 nm) was obtained. Owing to the data quality, only the data of the former four size bins were used in this study. During this campaign, the duration of each scan was completed within around 240 s.
2.2.3 Pollutant gases, PM2.5, and meteorological parameter measurement
The concentration of trace gases, including SO2, O3, CO, and NOx, was measured continuously during this campaign using different Thermo Fisher analyzers (43i-TLE, 49i, 48i, and 42i), respectively, at a time resolution of 1 min. The concentration of oxygenated volatile organic compounds (OVOCs) was measured with an iodide-adduct long time-of-flight chemical ionization mass spectrometer (I-CIMS; Aerodyne Research Inc., USA) at a time resolution of 10–30 s for the current study.
In addition, ambient meteorological conditions, such as wind speed, wind direction, temperature, relative humidity, and solar radiation, were also regularly measured in another building, which is located about 20 m to the southwest of the container, at the same observational site.
Furthermore, in order to investigate the influence of the origins and transport paths of air parcels to the local atmospheric compositions during NPF events, 72 h back-trajectories of air masses arriving at 100 m a.g.l. (above ground level) at our GC site were analyzed using the HYbrid Single-Particle Lagrangian Integrated Trajectory (HYSPLIT) model for the classified event days.
2.3 Data processing
2.3.1 Formation rate () and growth rate (GR)
defines the formation rate of atmospheric particles at a certain diameter (DP) and can be calculated, according to Kulmala et al. (2012), as follows:
where N is the particle number concentration between the diameter dp2 and dp1 (denoted as ΔDP), CoagS is the coagulation sink of particles, and GR is the particle growth rate out of the selected size bin.
In our study, we used two independent methods to calculate GR. One is the maximum concentration method (Kulmala et al., 2012), which is mainly for the PSM data. The other is based on the variation in the geometric mean diameters of the particle number size distribution, which is derived by fitting the PNSD into two or three log-normal modes using an automatic algorithm (DO-FIT model; Hussein et al., 2005), which is mainly for SMPS data.
where dp1 and dp2 were particle diameters at time t1 and t2, respectively.
2.3.2 Condensation sink (CS) and coagulation sink (CoagS)
The CS describes how fast the low-volatility molecules condense onto pre-existing aerosols and can be expressed as follows (Kulmala et al., 2012):
where D is the diffusion coefficient of the condensing vapor, which is usually referred to sulfuric acid, and βm,dp is the mass flux transition correction factor.
The CoagS represents how fast the freshly formed particles are lost to pre-existing particles through coagulation and can be calculated as follows:
where is the collision efficiency between particles at the diameter from dp to dp′.
2.3.3 Sulfuric acid proxy (SA proxy)
Sulfuric acid (SA) was considered to be one of the key precursors responsible for particle nucleation in the atmosphere. However, no direct measurement for the concentration of SA was available in current study. We therefore used a proxy variable to substitute the concentration of SA, as SA is mainly produced by the oxidation of SO2 by OH radicals, which can be approximated by the UV-B intensity (Petäjä et al., 2009). Thus, the proxy concentration of SA can be calculated, by Lu et al. (2019), as follows:
2.3.4 Classification of NPF event
The days of the NPF events were classified according to the method proposed by Dal Maso et al. (2005) and Kulmala et al. (2012), in which (i) a burst in the concentration of sub−3 nm particles or clusters was observed, and (ii) these particles had a continuous growth over a time span of hours (e.g., usually more than 10 h). If no clear growth of these newly formed particles (sub−3 nm particles) can be identified, then the day was classified as an undefined day. The days without both the burst of sub−3 nm particles and their subsequent growth were considered to be non-event days.
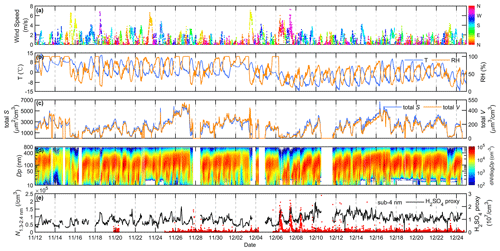
Figure 2Time series of (a) wind speed and wind direction, (b) temperature (T), and relative humidity (RH), (c) total particle surface and volume concentration calculated by using PNSD data, (d) measured PNSD in the size range of 10–800 nm, (e) particle number concentration in the range of 1.3 to 2.4 nm, and H2SO4 proxy concentration during the entire measurement period (12 November–24 December 2018). The white portion indicates that no data were available due to instrument maintenance or power failure. Note that the white portion in the PNSD in the size range of 10–15 nm, indicating no available data, is due to technical problems with our SMPS system; therefore, data for that time period from a parallel SMPS covering sizes of 15–800 nm were used instead.
2.3.5 Indicator for the occurrence of NPF
Previously, McMurry et al. (2005) proposed a dimensionless criterion, L, to predict the occurrence of NPF events in the atmosphere. After being validated in diverse atmospheric environments (Kuang et al., 2010; Cai et al., 2017), L has been used to investigate the governing factors for NPF events under typical atmospheric conditions. Recently, Cai et al. (2021a) proposed a new indicator, I, on the basis of L, which only considered H2SO4 to be driving the growth. The new indicator was calculated by further taking into account the condensation of other species (for instance, amines) and has been suggested to be a good quantitative representation for the occurrence of NPF after comparing with L for NPF events observed in urban Beijing (Deng et al., 2020). Detailed information for the calculation of I can be found in Cai et al. (2021a).
3.1 General characteristics of NPF at GC site
Figure 2 shows the time series of meteorological parameters (Fig. 2a shows the wind speed and direction; Fig. 2b shows the temperature and relative humidity) and aerosol properties (Fig. 2c shows the total surface and volume concentration; Fig. 2d and e show the PNSD in the size range of 10 to 800 nm and particle number concentration in the range of 1.3 to 2.4 nm) during this field campaign. During our study, wind speed was typically quite low, with an average of 1.18 m s−1, indicating stagnant meteorological conditions for the limited dilution of air pollutants at the current site. The temperature and relative humidity (RH) show opposite diurnal variations over the observational period, with the highest temperature and lowest RH during daytime and vice versa during nighttime. The observed time series of the concentration of different trace gases during current study is shown in Fig. S1 in the Supplement. To be specific, the campaign-averaged concentration of CO, O3, NOx, and SO2 was 1394, 7, 83, and 10 ppb, respectively.
According to the PNSD and PSM data, 5 d, with 4 d having a significant burst of sub−3 nm clusters (as shown in Fig. 2e), were classified as being NPF events occurring during the total experimental period. It has to be noted that, on the day of 18 November, though PSM data were not available due to technical issues, clear growth of nucleation mode particles with a typical banana-shaped PNSD was observed and lasted for more than 12 h. These particles under the growth of such a long time should not be from traffic emissions or transported particles. Therefore, it was also classified as an event day in our study. Considering all of these five NPF events, this corresponds to an NPF frequency of 12.8 %, which was lower than that at an urban site (i.e., Beijing) in the same region during the same season (Shen et al., 2018b found 25.8 %; Deng et al., 2020 found 51.4 %). Similar findings were also observed in Yue et al. (2009) and Wang et al. (2013) who found that NPF frequencies were higher at the Beijing urban site than at the corresponding regional background or rural site. Yue et al. (2009) and Wang et al. (2013) attributed this to the higher pollution level and correspondingly higher precursor content in the urban cities, leading to stronger NPF events there.
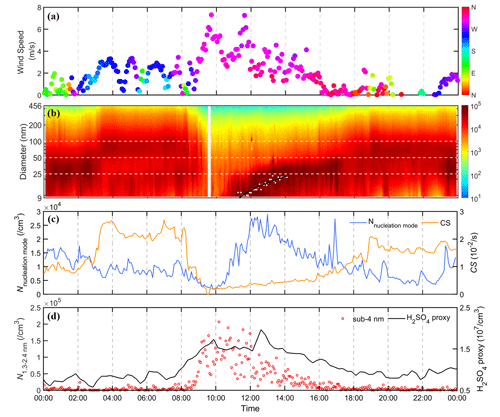
Figure 3A case of an NPF event on 7 December during this field campaign. Time series of (a) wind speed and wind directions and (b) the PNSD in the size range of 10–450 nm (the dotted white line represents the size with diameter at 25, 50, and 100 nm; black line represents the polynomial fit of the measured PNSD). (c) The particle number concentration of nucleation mode (9–25 nm) and CS. (d) The number concentration of sub−3 nm clusters and predicted concentration of sulfuric acid.
During our study, 6 d, with a slightly weak burst of sub−3 nm particles, were identified as being undefined days, as their formation and growth rate cannot be calculated accurately. For non-event days, we observed that, during many of them, some nucleation mode particles with sizes above 10 nm did appear. However, we did not observe the burst of sub−3 nm clusters from the PSM measurements, and moreover, no clear growth of these particles can be identified. This indicates that these small particles are probably not from the nucleation of H2SO4 with other species and their subsequent growth but more likely local emissions (traffic exhausts) or long-range transported particles.
Figure 3 shows a typical NPF event on 7 December as an example. Northwesterly wind prevailed, with elevated wind speed starting from around 08:00 LT, which was conducive to the diffusion of local pollutants, leading to a rapid decrease in CS concurrently. At the same time, an obvious rise in the H2SO4 concentration was observed, coinciding with a strong burst in the concentration of sub−3 nm clusters. Then, new particles with diameters larger than 10 nm, as shown in Fig. 3b, gradually formed by growth (exhibited as a visible banana shape in PNSD).
Table 1Summaries of the parameters (average values) relevant for NPF events during wintertime in China and other countries.
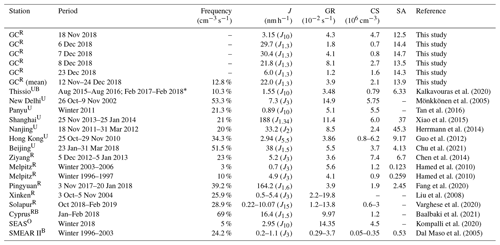
SEAS is the southeastern Arabian Sea, R is the rural site, UB is the urban background site, RB is the rural background site, U is the urban site, B is the background site, O is the ocean site, * is only in wintertime, and “–” is no number.
For all the identified NPF events, the formation rate of 1.3 nm (J1.3) particles ranged from 6.0 to about 30.4 cm−3 s−1, with an average value of 22.0 cm−3 s−1 at our GC site during the measurement period. Note that most atmospheric formation rates reported in China were based on the measured formation rates at relatively larger size, i.e., 3–10 nm, which are so-called “apparent” particle formation rates. In order to derive the formation rates of critical clusters from the apparent particle formation rates (Kulmala et al., 2017), the nuclei GR or GR at sub−3 nm is needed but usually remains unclear. Therefore, we focused more on the formation rate of particles at sizes below 3 nm in the following discussion. In principle, the particle formation rate is inversely proportional to the CS, as the nucleation precursors or clusters would be scavenged more rapidly under higher CS conditions, leading to a slower nanoparticle formation with a lower J. However, as shown in Table 1, in spite of the higher CS, the particle formation rates at our site appear to be higher than those in clean environments. This kind of intensive NPF becomes more noticeable for those Chinese megacities, such as Shanghai, Beijing, and Nanjing, which have an even higher J and CS compared to that at our GC site. The most plausible explanation could be the higher abundance of nucleating precursors for NPF in those polluted atmospheres, which is indicated by the SA concentration that is either measured in urban Shanghai and Nanjing or calculated in our study. To be specific, the mean SA proxy concentration during NPF at our GC site was around 1.4×107 cm−3, which is a factor of around 30 higher than that at Hyytiälä in Finland (Nieminen et al., 2014). The SA concentration during NPF at Shanghai (Xiao et al., 2015) and Nanjing (Herrmann et al., 2014) was even higher, at around 4×107 cm−3.
Although the formation rate of 1.3 nm particles is relatively high, the newly formed particles at our GC site usually cannot grow into very large particles within a short time, as indicated by their low GR. The average value of GR1.3–2.4 and GR9–15 at our site was 0.5 and 3.9 nm h−1, respectively, which is generally lower than many clean environments – GR1–3 of 0.9 nm h−1 for Hyytiälä (Kulmala, 2013) and of 5.1 nm h−1 for Jungfraujoch (Boulon et al., 2010) – but similar to those at urban Beijing (Chu et al., 2021) and rural Pingyuan (Fang et al., 2020). This could be attributed to the high CS or CoagS at those polluted environments, as the growth of small particles is limited, which are more vulnerable to the coagulation scavenging. However, despite the high CoagS, the observed GR at Shanghai and Nanjing was still exceptionally high. This discrepancy suggests that, besides the high concentration of precursors (mainly H2SO4) in polluted environments, including both rural and urban sites, other precursors with different efficiencies for nanoparticle growth and other involving mechanisms (for instance, multiphase reactions) may all contribute to the nanoparticle growth but have yet to be elucidated.
3.2 Potential mechanisms for NPF events in the rural NCP
To further understand the dominating nucleation mechanism in the rural atmosphere of NCP in China, we plotted the measured formation rate of 1.3 nm particles (J1.3) against the simulated H2SO4 concentration and compared the results to previous studies conducted in different environments, as shown in Fig. 4. As illustrated by the significant correlation between the concentration of sulfuric acid and the particle formation rates, sulfuric acid is considered to be the driving species in the initial steps of NPF, as confirmed conventionally. However, the obtained J1.3–H2SO4 relationship for current environment appeared to deviate largely from those obtained by other studies. If only referring to the slope of the J1.3–H2SO4 relationship, our results seem to approximate most to the ones measured by these CLOUD (the Cosmics Leaving OUtdoor Droplets chamber) experiments, based on the mechanism of H2SO4-DMA (dimethylamine) nucleation. However, without the direct measurements of other potential precursors, the molecules stabilizing H2SO4 clustering still remain unclear.
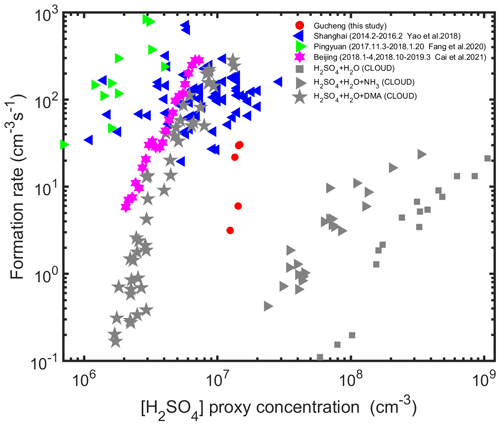
Figure 4The particle formation rate (J1.3) as a function of H2SO4 concentration for our study and for urban Shanghai (Yao et al., 2018), Beijing (Cai et al., 2021b), rural Pingyuan (Fang et al., 2020), and CLOUD measurements. The gray square, triangle, pentagram, and diamond represent CLOUD data for H2SO4 + H2O, H2SO4 + H2O + NH3, and H2SO4 + H2O + DMA (Kirkby et al., 2011; Riccobono et al., 2014), where DMA represents dimethylamine.
Comparing the particle formation rates reported in different environments in China, our results were of a similar magnitude to that in Beijing (Cai et al., 2021b), an urban site in the NCP. It has to be noted that the Cai et al. (2021b) study was conducted during a much longer time and completely covered the measurement period of our study. More importantly, during the 5 d of events in our study, NPF concurrently occurred at their measurement site (Liu et al., 2020). Additionally, for these 5 event days, air masses arriving at our site followed similar transport paths to that in urban Beijing (see Fig. S2 in the Supplement for an example), as they both originated from Siberian areas, where the concentration of gaseous pollutants and particulate matter was typically quite low, through the northwest of the observational sites. Taking both sources of evidence, we hypothesize that NPF events during these days in this area might be a regional phenomenon that is sharing the same or a similar nucleation mechanism. Cai et al. (2021b) and Yan et al. (2021) further concluded that H2SO4-DMA was the dominating nucleation mechanism for urban Beijing, with additional support from the measured C2-amine concentration. Considering the similarities between these two sites, we speculated that the clustering of H2SO4 with DMA may also dominate the nucleation process at our site during winter, though future work is needed to verify the current hypothesis.
On the other hand, we noticed that our results deviate significantly from the measured formation rate at Pingyuan (Fang et al., 2020), which is another rural site in the NCP. They concluded that neither H2SO4-NH3 nor H2SO4-DMA mechanisms could fully explain their observed particle formation rate but suggested that gaseous dicarboxylic acids were the dominating species for the initial step of H2SO4 clustering under a diacid-rich environment. Being similar to the rural environment of NCP, we cannot completely rule out the contribution of dicarboxylic acids to the H2SO4 stabilization. However, as illustrated in Fig. S4, the concentration of these four dicarboxylic acids during NPF events was in general lower than that during non-event days. Furthermore, during the daytime of events days when NPF was typically initiated, the signals of these diacids obtained from the I-CIMS did not show a clear increase, unlike sulfuric acid, but were rather elevated during the nighttime (see Fig. S5), which is obviously different to the case of Pingyuan. Hence, the involvement of diacids during the initial steps of nucleation under the current rural atmosphere might not hold. This statement does not necessarily mean that our previous inference was incorrect but, on the other hand, provides some hints that although NPF events in the NCP are regional, there might be no uniform theory but multiple mechanisms coexisting to explain its features, with the dominating one varying according to different emission patterns or meteorological conditions.
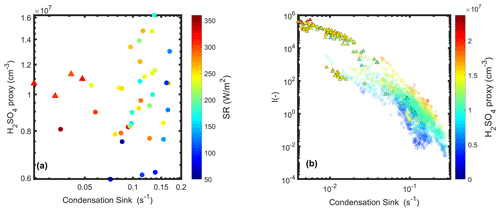
Figure 5(a) H2SO4 concentration as a function of the condensation sink during both event days and non-event days during our study. (b) The dimensionless indicator, I, as a function of the condensational sink. For both panels, the triangles indicate data for event days, while the circles indicate data for non-event days. The color bar indicates the solar radiation (a) and H2SO4 proxy concentration (b).
3.3 Governing factors for the occurrence of NPF in rural NCP
The high concentration of SO2, NH3, NOx, and VOCs (Chu et al., 2019), as well as fine particles, makes the NCP of China a unique context for NPF compared to many other environments. In principle, the competition between how fast the newly formed clusters grow and how fast they are scavenged determines whether or not NPF will occur in the atmosphere. However, in the NCP, the concentration of SA was typically quite high, probably reaching its maximum rate to form clusters. Thus, CS or CoagS becomes the dominant factor controlling the occurrence of NPF. This was partly confirmed by existing observations; for instance, Cai et al. (2021b) found that H2SO4 was high enough in urban Beijing but did not necessarily lead to the occurrence of NPF there. Their research pointed out that as long as CS or CoagS was below a certain threshold (Cai et al., 2017), NPF is very likely to occur.
Was this also true for the rural atmosphere in the NCP? By comparing with non-event days at our site (see Fig. 5a), we noticed that the H2SO4 level was not significantly higher (but sometimes even lower) than that during non-event days. In other words, the abundance of H2SO4 did not always lead to NPF, and it was only when CS was significantly lowered that the event became more likely to occur. This strongly demonstrates the similarity between our site with that of urban Beijing in that CS would be the limiting factor for the occurrence of NPF. However, we noticed that there were a very few cases (two cases) in which the CS was somewhat low, with the levels being quite close to that under those event days, yet NPF still did not occur. The most plausible explanation for this could be, on the one hand, the lowered H2SO4 concentration on these days (as shown in Fig. 5a) and, on the other hand, that the other nucleating species rather than H2SO4 may not be always enough to initiate nucleation at this site.
As previously stated, the dimensionless criterion, I, is a good quantitative indicator to predict whether an NPF occurs or not during a certain day. Thus, we plotted I against the condensational sink for NPF days and other days under different H2SO4 levels. Cai et al. (2021a) found that the larger the I value, the higher frequency at which NPF events occurred for both urban Beijing and Shanghai, which was also clearly revealed by our results. On the one hand, as shown in Fig. 5b, the largest I values were mostly observed for NPF days, confirming its feasibility in predicting the occurrence of NPF events. On the other hand, the obtained I anti-correlated with CS quite well, while the influence from the available H2SO4 was not obvious. This strongly suggests that CS was the dominating factor governing the appearance of NPF events in the current environment, which is highly consistent with the feature observed in Beijing.
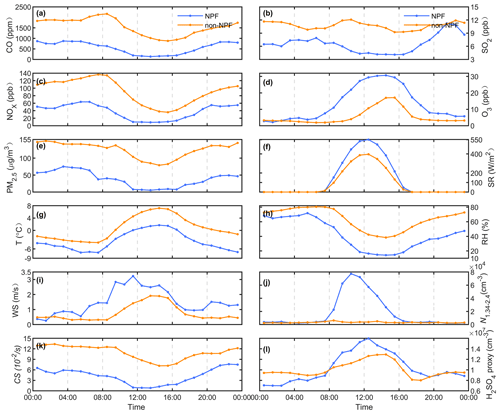
Figure 6Diurnal variation in (a) CO, (b) SO2, (c) NOx, (d) O3, (e) PM2.5, (f) solar radiation (SR), (g) T, (h) RH, (i) wind speed (WS), (j) number concentration of sub−3 nm cluster, (k) CS, and (l) H2SO4 proxy during the NPF and non-NPF days during this field campaign. These values were averaged over the 5 NPF days and 28 non-event days, respectively.
Moreover, we found that the RH level under event days was generally lower than that on non-event days (see Fig. 6). This is similar to the cases for which NPF was observed in Beijing by Yue et al. (2009), who suggested that photochemical reactions were faster on sunny days with low RH. In addition to this, the ambient temperature during NPF was relatively lower than that on non-event days (Kirkby et al., 2011; Riccobono et al., 2014). Yan et al. (2021) considered that temperature can affect the stability of H2SO4 clustering and thus influence NPF. Therefore, all of these factors could be the potential reasons increase or decrease the probability of NPF occurring in current rural areas. It has to be noted that all these features, including the reduced RH level in addition to ambient T during event days, could be coincidental, with reduced CS over clean days, for instance, being a consequence of air masses originating from the north and bringing drier, colder, and cleaner air to the site. Therefore, current discussion in this regard becomes ambiguous and may be inclusive but should still be considered separately when larger datasets are available. In addition, we observed that the O3 concentration was clearly higher during event days, implying that other condensable vapors, for instance, organics that involve O3, among others, in forming HOM, might also be important to NPF in this region. Although these organic compounds formed through O3 oxidation (Mohr et al., 2019) may not necessarily participate in H2SO4 clustering, they may contribute considerably to the growth of newly formed particles, which should not be ruled out in the study of NPF for this region and also need to be investigated in the future.
Most previous studies dealing with NPF in China were mainly based on the measurements of particles at larger sizes, typically above 3 nm, whereas the detection of particles at the sub−3 nm range was quite limited. In our study, by coupling a PSM with a traditional SMPS, we were able to measure the particle number size distribution down to 1.3 nm during NPF events in the wintertime at a rural site on the NCP. Correspondingly, the formation rate of particles at 1.3 nm was obtained, widening the data pool concerning the feature of NPF for this region. At the current rural environment, a high level of H2SO4 may not always initiate the occurrence of NPF. Only under the condition in which the CS was considerably low were NPF events more likely to take place. This feature is quite similar to that of the urban atmosphere of NCP, whereas NPF events were usually characterized by high formation rates, high CS, and high H2SO4 concentration. However, as our H2SO4 concentration was predicted from empirical parameters, we emphasize the need for caution regarding their particular associated uncertainties. Yang et al. (2021) demonstrated that the derived fitting parameters for the calculations of H2SO4 proxy may vary from site to site and between different seasons. For instance, they considered that the products from the ozonolysis of alkenes were able to oxidize SO2 to form gaseous H2SO4. Moreover, they pointed out that H2SO4 could be from primary emissions, such as vehicles or freshly emitted plumes. Sulfuric acid from these sources could account for 10 % of the total H2SO4 in the atmosphere. These aspects were not comprehensively considered in our calculations, which could bring huge uncertainties or errors to the estimation. Thereby, direct measurements of the H2SO4 concentration should be implemented in the future before deriving any further conclusions.
The details of the data used in this work can be found at https://doi.org/10.5281/zenodo.7326388 (Hong et al., 2022).
The supplement related to this article is available online at: https://doi.org/10.5194/acp-23-5699-2023-supplement.
JH collected the resources, wrote, and finalized the paper. MT analyzed the data, plotted the figures, and wrote the original draft. QW and NM planned the study, collected the resources, and reviewed the paper. SZhu, SZha, XP, LX, GL, and UK conducted the measurements. CY, JT, YK, YH, YZ, WX, GZ, BY, and ZW discussed the results. YC and HS contributed to fund acquisition.
At least one of the (co-)authors is a member of the editorial board of Atmospheric Chemistry and Physics. The peer-review process was guided by an independent editor, and the authors also have no other competing interests to declare.
Publisher's note: Copernicus Publications remains neutral with regard to jurisdictional claims in published maps and institutional affiliations.
This work has been supported by the National Natural Science Foundation of China (grant nos. 42175117, 41907182, 41877303, and 91644218) and the National key R&D Program of China (grant no. 2018YFC0213901), the Fundamental Research Funds for the Central Universities (grant no. 21621105), and Special Fund Project for Science and Technology Innovation Strategy of Guangdong Province (grant no. 2019B121205004).
This paper was edited by Birgit Wehner and reviewed by two anonymous referees.
Almeida, J., Schobesberger, S., Kürten, A., Ortega, I. K., Kupiainen-Määttä, O., Praplan, A. P., Adamov, A., Amorim, A., Bianchi, F., Breitenlechner, M., David, A., Dommen, J., Donahue, N. M., Downard, A., Dunne, E., Duplissy, J., Ehrhart, S., Flagan, R. C., Franchin, A., Guida, R., Hakala, J., Hansel, A., Heinritzi, M., Henschel, H., Jokinen, T., Junninen, H., Kajos, M., Kangasluoma, J., Keskinen, H., Kupc, A., Kurtén, T., Kvashin, A. N., Laaksonen, A., Lehtipalo, K., Leiminger, M., Leppä, J., Loukonen, V., Makhmutov, V., Mathot, S., McGrath, M. J., Nieminen, T., Olenius, T., Onnela, A., Petäjä, T., Riccobono, F., Riipinen, I., Rissanen, M., Rondo, L., Ruuskanen, T., Santos, F. D., Sarnela, N., Schallhart, S., Schnitzhofer, R., Seinfeld, J. H., Simon, M., Sipilä, M., Stozhkov, Y., Stratmann, F., Tomé, A., Tröstl, J., Tsagkogeorgas, G., Vaattovaara, P., Viisanen, Y., Virtanen, A., Vrtala, A., Wagner, P. E., Weingartner, E., Wex, H., Williamson, C., Wimmer, D., Ye, P., Yli-Juuti, T., Carslaw, K. S., Kulmala, M., Curtius, J., Baltensperger, U., Worsnop, D. R., Vehkamäki, H., and Kirkby, J.: Molecular understanding of sulphuric acid-amine particle nucleation in the atmosphere, Nature, 502, 359–363, https://doi.org/10.1038/nature12663, 2013.
Baalbaki, R., Pikridas, M., Jokinen, T., Laurila, T., Dada, L., Bezantakos, S., Ahonen, L., Neitola, K., Maisser, A., Bimenyimana, E., Christodoulou, A., Unga, F., Savvides, C., Lehtipalo, K., Kangasluoma, J., Biskos, G., Petäjä, T., Kerminen, V.-M., Sciare, J., and Kulmala, M.: Towards understanding the characteristics of new particle formation in the Eastern Mediterranean, Atmos. Chem. Phys., 21, 9223–9251, https://doi.org/10.5194/acp-21-9223-2021, 2021.
Berndt, T., Stratmann, F., Sipilä, M., Vanhanen, J., Petäjä, T., Mikkilä, J., Grüner, A., Spindler, G., Lee Mauldin, R., Curtius, J., Kulmala, M., and Heintzenberg, J.: Laboratory study on new particle formation from the reaction OH + SO2: Influence of experimental conditions, H2O vapour, NH3 and the amine tert-butylamine on the overall process, Atmos. Chem. Phys., 10, 7101–7116, https://doi.org/10.5194/acp-10-7101-2010, 2010.
Bianchi, F., Tröstl, J., Junninen, H., Frege, C., Henne, S., Hoyle, C. R., Molteni, U., Herrmann, E., Adamov, A., Bukowiecki, N., Chen, X., Duplissy, J., Gysel, M., Hutterli, M., Kangasluoma, J., Kontkanen, J., Kürten, A., Manninen, H. E., Münch, S., Peräkylä, O., Petäjä, T., Rondo, L., Williamson, C., Weingartner, E., Curtius, J., Worsnop, D. R., Kulmala, M., Dommen, J., and Baltensperger, U.: New particle formation in the free troposphere: A question of chemistry and timing, Science, 352, 1109–1112, https://doi.org/10.1126/science.aad5456, 2016.
Boulon, J., Sellegri, K., Venzac, H., Picard, D., Weingartner, E., Wehrle, G., Collaud Coen, M., Bütikofer, R., Flückiger, E., Baltensperger, U., and Laj, P.: New particle formation and ultrafine charged aerosol climatology at a high altitude site in the Alps (Jungfraujoch, 3580 m a.s.l., Switzerland), Atmos. Chem. Phys., 10, 9333–9349, https://doi.org/10.5194/acp-10-9333-2010, 2010.
Cai, R., Yang, D., Fu, Y., Wang, X., Li, X., Ma, Y., Hao, J., Zheng, J., and Jiang, J.: Aerosol surface area concentration: A governing factor in new particle formation in Beijing, Atmos. Chem. Phys., 17, 12327–12340, https://doi.org/10.5194/acp-17-12327-2017, 2017.
Cai, R., Yan, C., Worsnop, D. R., Bianchi, F., Kerminen, V.-M., Liu, Y., Wang, L., Zheng, J., Kulmala, M., and Jiang, J.: An indicator for sulfuric acid–amine nucleation in atmospheric environments, Aerosol Sci. Tech., 55, 1059–1069, https://doi.org/10.1080/02786826.2021.1922598, 2021a.
Cai, R., Yan, C., Yang, D., Yin, R., Lu, Y., Deng, C., Fu, Y., Ruan, J., Li, X., Kontkanen, J., Zhang, Q., Kangasluoma, J., Ma, Y., Hao, J., Worsnop, D. R., Bianchi, F., Paasonen, P., Kerminen, V. M., Liu, Y., Wang, L., Zheng, J., Kulmala, M., and Jiang, J.: Sulfuric acid-amine nucleation in urban Beijing, Atmos. Chem. Phys., 21, 2457–2468, https://doi.org/10.5194/acp-21-2457-2021, 2021b.
Chen, C., Hu, M., Wu, Z., Wu, Y., Guo, S., Chen, W., Luo, B., Shao, M., Zhang, Y., and Xie, S. Characterization of new particle formation event in the rural site of Sichuan Basin and its contribution to cloud condensation nuclei, China Environ. Sci., 34, 2764–2772, 2014.
Chu, B., Matti Kerminen, V., Bianchi, F., Yan, C., Petäjä, T., and Kulmala, M.: Atmospheric new particle formation in China, Atmos. Chem. Phys., 19, 115–138, https://doi.org/10.5194/acp-19-115-2019, 2019.
Chu, B., Dada, L., Liu, Y., Yao, L., Wang, Y., Du, W., Cai, J., Dällenbach, K. R., Chen, X., Simonen, P., Zhou, Y., Deng, C., Fu, Y., Yin, R., Li, H., He, X. C., Feng, Z., Yan, C., Kangasluoma, J., Bianchi, F., Jiang, J., Kujansuu, J., Kerminen, V. M., Petäjä, T., He, H., and Kulmala, M.: Particle growth with photochemical age from new particle formation to haze in the winter of Beijing, China, Sci. Total Environ., 753, 142207, https://doi.org/10.1016/j.scitotenv.2020.142207, 2021.
Dal Maso, M., Kulmala, M., Riipinen, I., Wagner, R., Hussein, T., Aalto, P. P., and Lehtinen, K. E. J.: Formation and growth of fresh atmospheric aerosols: Eight years of aerosol size distribution data from SMEAR II, Hyytiälä, Finland, Boreal Environ. Res., 10, 323–336, 2005.
Deng, C., Fu, Y., Dada, L., Yan, C., Cai, R., Yang, D., Zhou, Y., Yin, R., Lu, Y., Li, X., Qiao, X., Fan, X., Nie, W., Kontkanen, J., Kangasluoma, J., Chu, B., Ding, A., Kerminen, V.-M., Paasonen, P., Worsnop, D. R., Bianchi, F., Liu, Y., Zheng, J., Wang, L., Kulmala, M., and Jiang, J.: Seasonal Characteristics of New Particle Formation and Growth in Urban Beijing, Environ. Sci. Technol., 54, 8547–8557, https://doi.org/10.1021/acs.est.0c00808, 2020.
Dunne, E. M., Gordon, H., Kürten, A., Almeida, J., Duplissy, J., Williamson, C., Ortega, I. K., Pringle, K. J., Adamov, A., Baltensperger, U., Barmet, P., Benduhn, F., Bianchi, F., Breitenlechner, M., Clarke, A., Curtius, J., Dommen, J., Donahue, N. M., Ehrhart, S., Flagan, R. C., Franchin, A., Guida, R., Hakala, J., Hansel, A., Heinritzi, M., Jokinen, T., Kangasluoma, J., Kirkby, J., Kulmala, M., Kupc, A., Lawler, M. J., Lehtipalo, K., Makhmutov, V., Mann, G., Mathot, S., Merikanto, J., Miettinen, P., Nenes, A., Onnela, A., Rap, A., Reddington, C. L. S., Riccobono, F., Richards, N. A. D., Rissanen, M. P., Rondo, L., Sarnela, N., Schobesberger, S., Sengupta, K., Simon, M., Sipilä, M., Smith, J. N., Stozkhov, Y., Tomé, A., Tröstl, J., Wagner, P. E., Wimmer, D., Winkler, P. M., Worsnop, D. R., and Carslaw, K. S.: Global atmospheric particle formation from CERN CLOUD measurements, Science, 354, 1119–1124, https://doi.org/10.1126/science.aaf2649, 2016.
Ehn, M., Thornton, J. A., Kleist, E., Sipilä, M., Junninen, H., Pullinen, I., Springer, M., Rubach, F., Tillmann, R., Lee, B., Lopez-Hilfiker, F., Andres, S., Acir, I.-H., Rissanen, M., Jokinen, T., Schobesberger, S., Kangasluoma, J., Kontkanen, J., Nieminen, T., Kurtén, T., Nielsen, L. B., Jørgensen, S., Kjaergaard, H. G., Canagaratna, M., Maso, M. D., Berndt, T., Petäjä, T., Wahner, A., Kerminen, V.-M., Kulmala, M., Worsnop, D. R., Wildt, J., and Mentel, T. F.: A large source of low-volatility secondary organic aerosol, Nature, 506, 476–479, https://doi.org/10.1038/nature13032, 2014.
Fang, X., Hu, M., Shang, D., Tang, R., Shi, L., Olenius, T., Wang, Y., Wang, H., Zhang, Z., Chen, S., Yu, X., Zhu, W., Lou, S., Ma, Y., Li, X., Zeng, L., Wu, Z., Zheng, J., and Guo, S.: Observational Evidence for the Involvement of Dicarboxylic Acids in Particle Nucleation, Environ. Sci. Technol. Lett., 7, 388–394, https://doi.org/10.1021/acs.estlett.0c00270, 2020.
Guo, H., Wang, D. W., Cheung, K., Ling, Z. H., Chan, C. K., and Yao, X. H.: Observation of aerosol size distribution and new particle formation at a mountain site in subtropical Hong Kong, Atmos. Chem. Phys., 12, 9923–9939, https://doi.org/10.5194/acp-12-9923-2012, 2012.
Guo, S., Hu, M., Zamora, M. L., Peng, J., Shang, D., Zheng, J., Du, Z., Wu, Z., Shao, M., Zeng, L., Molina, M. J., and Zhang, R.: Elucidating severe urban haze formation in China, P. Natl. Acad. Sci. USA, 111, 17373–17378, https://doi.org/10.1073/pnas.1419604111, 2014.
Hamed, A., Birmili, W., Joutsensaari, J., Mikkonen, S., Asmi, A., Wehner, B., Spindler, G., Jaatinen, A., Wiedensohler, A., Korhonen, H., J. Lehtinen, K. E., and Laaksonen, A.: Changes in the production rate of secondary aerosol particles in Central Europe in view of decreasing SO2 emissions between 1996 and 2006, Atmos. Chem. Phys., 10, 1071–1091, https://doi.org/10.5194/acp-10-1071-2010, 2010.
Herrmann, E., Ding, A. J., Kerminen, V. M., Petäjä, T., Yang, X. Q., Sun, J. N., Qi, X. M., Manninen, H., Hakala, J., Nieminen, T., Aalto, P. P., Kulmala, M., and Fu, C. B.: Aerosols and nucleation in eastern China: First insights from the new SORPES-NJU station, Atmos. Chem. Phys., 14, 2169–2183, https://doi.org/10.5194/acp-14-2169-2014, 2014.
Hong, J., Tang, M., Wang, Q., Ma, N., Zhu, S., Zhang, S., Pan, X., Xie, L., Li, G., Kuhn, U., Yan, C., Tao, J., Kuang, Y., He, Y., Xu, W., Cai, R., Zhou, Y., Wang, Z., Zhou, G., Yuan, B., Cheng, Y., and Su, H.: Data sets: Wintertime new particle formation in the rural area of North China Plain: influencing factors and possible formation mechanism, Zenodo [data set], https://doi.org/10.5281/zenodo.7326388, 2022.
Hussein, T., Dal Maso, M., Petäjä, T., Koponen, I. K., Paatero, P., Aalto, P. P., Hämeri, K., and Kulmala, M.: Evaluation of an automatic algorithm for fitting the particle number size distributions, Boreal Environ. Res., 10, 337–355, 2005.
Jokinen, T., Sipilä, M., Junninen, H., Ehn, M., Lönn, G., Hakala, J., Petäjä, T., Mauldin, R. L., Kulmala, M., and Worsnop, D. R.: Atmospheric sulphuric acid and neutral cluster measurements using CI-APi-TOF, Atmos. Chem. Phys., 12, 4117–4125, https://doi.org/10.5194/acp-12-4117-2012, 2012.
Kalkavouras, P., Bougiatioti, A., Grivas, G., Stavroulas, I., Kalivitis, N., Liakakou, E., Gerasopoulos, E., Pilinis, C., and Mihalopoulos, N.: On the regional aspects of new particle formation in the Eastern Mediterranean: A comparative study between a background and an urban site based on long term observations, Atmos. Res., 239, 104911, https://doi.org/10.1016/j.atmosres.2020.104911, 2020.
Kirkby, J., Curtius, J., Almeida, J., Dunne, E., Duplissy, J., Ehrhart, S., Franchin, A., Gagné, S., Ickes, L., Kürten, A., Kupc, A., Metzger, A., Riccobono, F., Rondo, L., Schobesberger, S., Tsagkogeorgas, G., Wimmer, D., Amorim, A., Bianchi, F., Breitenlechner, M., David, A., Dommen, J., Downard, A., Ehn, M., Flagan, R. C., Haider, S., Hansel, A., Hauser, D., Jud, W., Junninen, H., Kreissl, F., Kvashin, A., Laaksonen, A., Lehtipalo, K., Lima, J., Lovejoy, E. R., Makhmutov, V., Mathot, S., Mikkilä, J., Minginette, P., Mogo, S., Nieminen, T., Onnela, A., Pereira, P., Petäjä, T., Schnitzhofer, R., Seinfeld, J. H., Sipilä, M., Stozhkov, Y., Stratmann, F., Tomé, A., Vanhanen, J., Viisanen, Y., Vrtala, A., Wagner, P. E., Walther, H., Weingartner, E., Wex, H., Winkler, P. M., Carslaw, K. S., Worsnop, D. R., Baltensperger, U., and Kulmala, M.: Role of sulphuric acid, ammonia and galactic cosmic rays in atmospheric aerosol nucleation, Nature, 476, 429–435, https://doi.org/10.1038/nature10343, 2011.
Kirkby, J., Duplissy, J., Sengupta, K., Frege, C., Gordon, H., Williamson, C., Heinritzi, M., Simon, M., Yan, C., Almeida, J., Trostl, J., Nieminen, T., Ortega, I. K., Wagner, R., Adamov, A., Amorim, A., Bernhammer, A. K., Bianchi, F., Breitenlechner, M., Brilke, S., Chen, X., Craven, J., Dias, A., Ehrhart, S., Flagan, R. C., Franchin, A., Fuchs, C., Guida, R., Hakala, J., Hoyle, C. R., Jokinen, T., Junninen, H., Kangasluoma, J., Kim, J., Krapf, M., Kurten, A., Laaksonen, A., Lehtipalo, K., Makhmutov, V., Mathot, S., Molteni, U., Onnela, A., Perakyla, O., Piel, F., Petaja, T., Praplan, A. P., Pringle, K., Rap, A., Richards, N. A. D., Riipinen, I., Rissanen, M. P., Rondo, L., Sarnela, N., Schobesberger, S., Scott, C. E., Seinfeld, J. H., Sipila, M., Steiner, G., Stozhkov, Y., Stratmann, F., Tomé, A., Virtanen, A., Vogel, A. L., Wagner, A. C., Wagner, P. E., Weingartner, E., Wimmer, D., Winkler, P. M., Ye, P., Zhang, X., Hansel, A., Dommen, J., Donahue, N. M., Worsnop, D. R., Baltensperger, U., Kulmala, M., Carslaw, K. S., and Curtius, J.: Ion-induced nucleation of pure biogenic particles, Nature, 533, 521–526, https://doi.org/10.1038/nature17953, 2016.
Kompalli, S. K., Nair, V. S., Jayachandran, V., Gogoi, M. M., and Babu, S. S.: Particle number size distributions and new particle formation events over the northern Indian Ocean during continental outflow, Atmos. Environ., 238, 117719, https://doi.org/10.1016/j.atmosenv.2020.117719, 2020.
Kuang, C., Riipinen, I., Sihto, S.-L., Kulmala, M., McCormick, A. V., and McMurry, P. H.: An improved criterion for new particle formation in diverse atmospheric environments, Atmos. Chem. Phys., 10, 8469–8480, https://doi.org/10.5194/acp-10-8469-2010, 2010.
Kulmala, M.: Direct Observations of Atmospheric Aerosol Nucleation, Science, 339, 943–946, https://doi.org/10.1126/science.1227385, 2013.
Kulmala, M., Pirjola, L., and Mäkelä, J. M.: Stable sulphate clusters as a source of new atmospheric particles, Nature, 404, 66–69, https://doi.org/10.1038/35003550, 2000.
Kulmala, M., Vehkamäki, H., Petäjä, T., Dal Maso, M., Lauri, A., Kerminen, V. M., Birmili, W., and McMurry, P. H.: Formation and growth rates of ultrafine atmospheric particles: A review of observations, J. Aerosol Sci., 35, 143–176, https://doi.org/10.1016/j.jaerosci.2003.10.003, 2004.
Kulmala, M., Petäjä, T., Nieminen, T., Sipilä, M., Manninen, H. E., Lehtipalo, K., Dal Maso, M., Aalto, P. P., Junninen, H., Paasonen, P., Riipinen, I., Lehtinen, K. E. J., Laaksonen, A., and Kerminen, V.-M.: Measurement of the nucleation of atmospheric aerosol particles, Nat. Protoc., 7, 1651–1667, https://doi.org/10.1038/nprot.2012.091, 2012.
Kulmala, M., Petäjä, T., Ehn, M., Thornton, J., Sipilä, M., Worsnop, D. R., and Kerminen, V. M.: Chemistry of atmospheric nucleation: On the recent advances on precursor characterization and atmospheric cluster composition in connection with atmospheric new particle formation, Annu. Rev. Phys. Chem., 65, 21–37, https://doi.org/10.1146/annurev-physchem-040412-110014, 2014.
Kulmama, M., Petäjä, T., Kerminen, V. M., Kujansuu, J., Ruuskanen, T., Ding, A., Nie, W., Hu, M., Wang, Z., Wu, Z., Wang, L., and Worsnop, D. R.: On secondary new particle formation in China, Front. Environ. Sci. Eng., 10, 1–10, https://doi.org/10.1007/s11783-016-0850-1, 2016.
Kulmala, M., Kerminen, V.-M., Petäjä, T., Ding, A. J., and Wang, L.: Atmospheric gas-to-particle conversion: why NPF events are observed in megacities?, Faraday Discuss., 200, 271–288, https://doi.org/10.1039/C6FD00257A, 2017.
Kürten, A., Bergen, A., Heinritzi, M., Leiminger, M., Lorenz, V., Piel, F., Simon, M., Sitals, R., Wagner, A. C., and Curtius, J.: Observation of new particle formation and measurement of sulfuric acid, ammonia, amines and highly oxidized organic molecules at a rural site in central Germany, Atmos. Chem. Phys., 16, 12793–12813, https://doi.org/10.5194/acp-16-12793-2016, 2016.
Lin, W., Xu, X., Ge, B., and Zhang, X.: Characteristics of gaseous pollutants at Gucheng, a rural site southwest of Beijing, J. Geophys. Res.-Atmos., 114, D00G14, https://doi.org/10.1029/2008JD010339, 2009.
Liu, S., Hu, M., Wu, Z., Wehner, B., Wiedensohler, A., and Cheng, Y.: Aerosol number size distribution and new particle formation at a rural/coastal site in Pearl River Delta (PRD) of China, Atmos. Environ., 42, 6275–6283, https://doi.org/10.1016/j.atmosenv.2008.01.063, 2008.
Liu, Y., Yan, C., Feng, Z., Zheng, F., Fan, X., Zhang, Y., Li, C., Zhou, Y., Lin, Z., Guo, Y., Zhang, Y., Ma, L., Zhou, W., Liu, Z., Dada, L., Dällenbach, K., Kontkanen, J., Cai, R., Chan, T., Chu, B., Du, W., Yao, L., Wang, Y., Cai, J., Kangasluoma, J., Kokkonen, T., Kujansuu, J., Rusanen, A., Deng, C., Fu, Y., Yin, R., Li, X., Lu, Y., Liu, Y., Lian, C., Yang, D., Wang, W., Ge, M., Wang, Y., Worsnop, D. R., Junninen, H., He, H., Kerminen, V. M., Zheng, J., Wang, L., Jiang, J., Petäjä, T., Bianchi, F., and Kulmala, M.: Continuous and comprehensive atmospheric observations in Beijing: a station to understand the complex urban atmospheric environment, Big Earth Data, 4, 295–321, https://doi.org/10.1080/20964471.2020.1798707, 2020.
Lu, Y., Yan, C., Fu, Y., Chen, Y., Liu, Y., Yang, G., Wang, Y., Bianchi, F., Chu, B., Zhou, Y., Yin, R., Baalbaki, R., Garmash, O., Deng, C., Wang, W., Liu, Y., Petäjä, T., Kerminen, V. M., Jiang, J., Kulmala, M., and Wang, L.: A proxy for atmospheric daytime gaseous sulfuric acid concentration in urban Beijing, Atmos. Chem. Phys., 19, 1971–1983, https://doi.org/10.5194/acp-19-1971-2019, 2019.
Ma, N., Zhao, C., Tao, J., Wu, Z., Kecorius, S., Wang, Z., Groß, J., Liu, H., Bian, Y., Kuang, Y., Teich, M., Spindler, G., Muller, K., Van Pinxteren, D., Herrmann, H., Hu, M., and Wiedensohler, A.: Variation of CCN activity during new particle formation events in the North China Plain, Atmos. Chem. Phys., 16, 8593–8607, https://doi.org/10.5194/acp-16-8593-2016, 2016.
McMurry, P. H., Fink, M., Sakurai, H., Stolzenburg, M. R., Mauldin, I. L., Smith, J., Eisele, F., Moore, K., Sjostedt, S., Tanner, D., Huey, L. G., Nowak, J. B., Edgerton, E., and Voisin, D.: A criterion for new particle formation in the sulfur-rich Atlanta atmosphere, J. Geophys. Res.-Atmos., 110, 1–10, https://doi.org/10.1029/2005JD005901, 2005.
Mirme, S. and Mirme, A.: The mathematical principles and design of the NAIS – A spectrometer for the measurement of cluster ion and nanometer aerosol size distributions, Atmos. Meas. Tech., 6, 1061–1071, https://doi.org/10.5194/amt-6-1061-2013, 2013.
Mohr, C., Thornton, J. A., Heitto, A., Lopez-Hilfiker, F. D., Lutz, A., Riipinen, I., Hong, J., Donahue, N. M., Hallquist, M., Petäjä, T., Kulmala, M., and Yli-Juuti, T.: Molecular identification of organic vapors driving atmospheric nanoparticle growth, Nat. Commun., 10, 4442, https://doi.org/10.1038/s41467-019-12473-2, 2019.
Mönkkönen, P., Koponen, I. K., Lehtinen, K. E. J., Hämeri, K., Uma, R., and Kulmala, M.: Measurements in a highly polluted Asian mega city: observations of aerosol number size distribution, modal parameters and nucleation events, Atmos. Chem. Phys., 5, 57–66, https://doi.org/10.5194/acp-5-57-2005, 2005.
Nieminen, T., Lehtinen, K. E. J., and Kulmala, M.: Sub-10 nm particle growth by vapor condensation-effects of vapor molecule size and particle thermal speed, Atmos. Chem. Phys., 10, 9773–9779, https://doi.org/10.5194/acp-10-9773-2010, 2010.
Nieminen, T., Asmi, A., Aalto, P. P., Keronen, P., Petäjä, T., Kulmala, M., Kerminen, V. M., Nieminen, T., and Dal Maso, M.: Trends in atmospheric new-particle formation: 16 years of observations in a boreal-forest environment, Boreal Environ. Res., 19, 191–214, 2014.
Petäjä, T., Mauldin, R. L., Kosciuch, E., McGrath, J., Nieminen, T., Paasonen, P., Boy, M., Adamov, A., Kotiaho, T., and Kulmala, M.: Sulfuric acid and OH concentrations in a boreal forest site, Atmos. Chem. Phys., 9, 7435–7448, https://doi.org/10.5194/acp-9-7435-2009, 2009.
Riccobono, F., Schobesberger, S., Scott, C. E., Dommen, J., Ortega, I. K., Rondo, L., Almeida, J., Amorim, A., Bianchi, F., Breitenlechner, M., David, A., Downard, A., Dunne, E. M., Duplissy, J., Ehrhart, S., Flagan, R. C., Franchin, A., Hansel, A., Junninen, H., Kajos, M., Keskinen, H., Kupc, A., Kürten, A., Kvashin, A. N., Laaksonen, A., Lehtipalo, K., Makhmutov, V., Mathot, S., Nieminen, T., Onnela, A., Petäjä, T., Praplan, A. P., Santos, F. D., Schallhart, S., Seinfeld, J. H., Sipilä, M., Spracklen, D. V., Stozhkov, Y., Stratmann, F., Tomé, A., Tsagkogeorgas, G., Vaattovaara, P., Viisanen, Y., Vrtala, A., Wagner, P. E., Weingartner, E., Wex, H., Wimmer, D., Carslaw, K. S., Curtius, J., Donahue, N. M., Kirkby, J., Kulmala, M., Worsnop, D. R., and Baltensperger, U.: Oxidation products of biogenic emissions contribute to nucleation of atmospheric particles, Science, 344, 717–721, https://doi.org/10.1126/science.1243527, 2014.
Shen, X., Sun, J., Zhang, X., Zhang, Y., Wang, Y., Tan, K., Wang, P., Zhang, L., Qi, X., Che, H., Zhang, Z., Zhong, J., Zhao, H., and Ren, S.: Comparison of Submicron Particles at a Rural and an Urban Site in the North China Plain during the December 2016 Heavy Pollution Episodes, J. Meteorol. Res., 32, 26–37, https://doi.org/10.1007/s13351-018-7060-7, 2018a.
Shen, X., Sun, J., Kivekäs, N., Kristensson, A., Zhang, X., Zhang, Y., Zhang, L., Fan, R., Qi, X., Ma, Q., and Zhou, H.: Spatial distribution and occurrence probability of regional new particle formation events in eastern China, Atmos. Chem. Phys., 18, 587–599, https://doi.org/10.5194/acp-18-587-2018, 2018b.
Sipilä, M., Berndt, T., Petäjä, T., Brus, D., Vanhanen, J., Stratmann, F., Patokoski, J., Mauldin III, R. L., Hyvärinen, A. P., Lihavainen, H., and Kulmala, M.: The Role of Sulfuric Acid in Atmospheric Nucleation, Science, 327, 1243–1246, 2010.
Spracklen, D. V., Carslaw, K. S., Kulmala, M., Kerminen, V. M., Mann, G. W., and Sihto, S. L.: The contribution of boundary layer nucleation events to total particle concentrations on regional and global scales, Atmos. Chem. Phys., 6, 5631–5648, https://doi.org/10.5194/acp-6-5631-2006, 2006.
Stolzenburg, D., Simon, M., Ranjithkumar, A., Kürten, A., Lehtipalo, K., Gordon, H., Ehrhart, S., Finkenzeller, H., Pichelstorfer, L., Nieminen, T., He, X.-C., Brilke, S., Xiao, M., Amorim, A., Baalbaki, R., Baccarini, A., Beck, L., Bräkling, S., Caudillo Murillo, L., Chen, D., Chu, B., Dada, L., Dias, A., Dommen, J., Duplissy, J., El Haddad, I., Fischer, L., Gonzalez Carracedo, L., Heinritzi, M., Kim, C., Koenig, T. K., Kong, W., Lamkaddam, H., Lee, C. P., Leiminger, M., Li, Z., Makhmutov, V., Manninen, H. E., Marie, G., Marten, R., Müller, T., Nie, W., Partoll, E., Petäjää, T., Pfeifer, J., Philippov, M., Rissanen, M. P., Rörup, B., Schobesberger, S., Schuchmann, S., Shen, J., Sipilä, M., Steiner, G., Stozhkov, Y., Tauber, C., Tham, Y. J., Tomé, A., Vazquez-Pufleau, M., Wagner, A. C., Wang, M., Wang, Y., Weber, S. K., Wimmer, D., Wlasits, P. J., Wu, Y., Ye, Q., Zauner-Wieczorek, M., Baltensperger, U., Carslaw, K. S., Curtius, J., Donahue, N. M., Flagan, R. C., Hansel, A., Kulmala, M., Lelieveld, J., Volkamer, R., Kirkby, J., and Winkler, P. M.: Enhanced growth rate of atmospheric particles from sulfuric acid, Atmos. Chem. Phys., 20, 7359–7372, https://doi.org/10.5194/acp-20-7359-2020, 2020.
Tan, H. B., Yin, Y., Li, F., Liu, X. T., Chan, P. W., Deng, T., Deng, X. J., Wan, Q. L., and Wu, D.: Measurements of particle number size distributions and new particle formation events during winter in the Pearl River Delta region, China, J. Trop. Meteorol., 22, 191–199, https://doi.org/10.16555/j.1006-8775.2016.02.009, 2016.
Tröstl, J., Chuang, W. K., Gordon, H., Heinritzi, M., Yan, C., Molteni, U., Ahlm, L., Frege, C., Bianchi, F., Wagner, R., Simon, M., Lehtipalo, K., Williamson, C., Craven, J. S., Duplissy, J., Adamov, A., Almeida, J., Bernhammer, A. K., Breitenlechner, M., Brilke, S., Dias, A., Ehrhart, S., Flagan, R. C., Franchin, A., Fuchs, C., Guida, R., Gysel, M., Hansel, A., Hoyle, C. R., Jokinen, T., Junninen, H., Kangasluoma, J., Keskinen, H., Kim, J., Krapf, M., Kürten, A., Laaksonen, A., Lawler, M., Leiminger, M., Mathot, S., Möhler, O., Nieminen, T., Onnela, A., Petäjä, T., Piel, F. M., Miettinen, P., Rissanen, M. P., Rondo, L., Sarnela, N., Schobesberger, S., Sengupta, K., Sipilä, M., Smith, J. N., Steiner, G., Tomè, A., Virtanen, A., Wagner, A. C., Weingartner, E., Wimmer, D., Winkler, P. M., Ye, P., Carslaw, K. S., Curtius, J., Dommen, J., Kirkby, J., Kulmala, M., Riipinen, I., Worsnop, D. R., Donahue, N. M., and Baltensperger, U.: The role of low-volatility organic compounds in initial particle growth in the atmosphere, Nature, 533, 527–531, https://doi.org/10.1038/nature18271, 2016.
Vanhanen, J., Mikkil, J., Lehtipalo, K., Sipil, M., Manninen, H. E., Siivola, E., and Pet, T.: Particle Size Magnifier for Nano-CN Detection, Aerosol Sci. Tech., 45, 533–542, https://doi.org/10.1080/02786826.2010.547889, 2011.
Varghese, M., Leena, P. P., Murugavel, P., Bankar, S., Todekar, K., Chowdhuri, S., Safai, P. D., Malap, N., Konwar, M., Dixit, S., Rao, Y. J., and Prabha, T. V.: New Particle Formation Observed from a Rain Shadow Region of the Western Ghats India, Toxicol. Environ. Chem., 102, 305–333, https://doi.org/10.1080/02772248.2020.1789134, 2020.
Wang, Z., Wu, Z., Yue, D., Shang, D., Guo, S., Sun, J., Ding, A., Wang, L., Jiang, J., Guo, H., Gao, J., Cheung, H. C., Morawska, L., Keywood, M., and Hu, M.: New particle formation in China: Current knowledge and further directions, Sci. Total Environ., 577, 258–266, https://doi.org/10.1016/j.scitotenv.2016.10.177, 2017.
Wang, Z. B., Hu, M., Sun, J. Y., Wu, Z. J., Yue, D. L., Shen, X. J., Zhang, Y. M., Pei, X. Y., Cheng, Y. F., and Wiedensohler, A.: Characteristics of regional new particle formation in urban and regional background environments in the North China Plain, Atmos. Chem. Phys., 13, 12495–12506, https://doi.org/10.5194/acp-13-12495-2013, 2013.
Xiao, S., Wang, M. Y., Yao, L., Kulmala, M., Zhou, B., Yang, X., Chen, J. M., Wang, D. F., Fu, Q. Y., Worsnop, D. R., and Wang, L.: Strong atmospheric new particle formation in winter in urban Shanghai, China, Atmos. Chem. Phys., 15, 1769–1781, https://doi.org/10.5194/acp-15-1769-2015, 2015.
Yan, C., Yin, R., Lu, Y., Dada, L., Yang, D., Fu, Y., Kontkanen, J., Deng, C., Garmash, O., Ruan, J., Baalbaki, R., Schervish, M., Cai, R., Bloss, M., Chan, T., Chen, T., Chen, Q., Chen, X., Chen, Y., Chu, B., Dällenbach, K., Foreback, B., He, X., Heikkinen, L., Jokinen, T., Junninen, H., Kangasluoma, J., Kokkonen, T., Kurppa, M., Lehtipalo, K., Li, H., Li, H., Li, X., Liu, Y., Ma, Q., Paasonen, P., Rantala, P., Pileci, R. E., Rusanen, A., Sarnela, N., Simonen, P., Wang, S., Wang, W., Wang, Y., Xue, M., Yang, G., Yao, L., Zhou, Y., Kujansuu, J., Petäjä, T., Nie, W., Ma, Y., Ge, M., He, H., Donahue, N. M., Worsnop, D. R., Veli-Matti Kerminen, Wang, L., Liu, Y., Zheng, J., Kulmala, M., Jiang, J., and Bianchi, F.: The Synergistic Role of Sulfuric Acid, Bases, and Oxidized Organics Governing New-Particle Formation in Beijing, Geophys. Res. Lett., 48, 1–12, https://doi.org/10.1029/2020GL091944, 2021.
Yang, L., Nie, W., Liu, Y., Xu, Z., Xiao, M., Qi, X., Li, Y., Wang, R., Zou, J., Paasonen, P., Yan, C., Xu, Z., Wang, J., Zhou, C., Yuan, J., Sun, J., Chi, X., Kerminen, V. M., Kulmala, M., and Ding, A.: Toward Building a Physical Proxy for Gas-Phase Sulfuric Acid Concentration Based on Its Budget Analysis in Polluted Yangtze River Delta, East China, Environ. Sci. Technol., 55, 6665–6676, https://doi.org/10.1021/acs.est.1c00738, 2021.
Yao, L., Garmash, O., Bianchi, F., Zheng, J., Yan, C., Paasonen, P., Sipilä, M., Wang, M., Wang, X., and Xiao, S.: Atmospheric new particle formation from sulfuric acid and amines in a Chinese megacity, Science, 281, 278–281, 2018.
Yu, H., Ren, L., and Kanawade, V. P.: New Particle Formation and Growth Mechanisms in Highly Polluted Environments, Curr. Pollut. Rep., 3, 245–253, https://doi.org/10.1007/s40726-017-0067-3, 2017.
Yue, D., Hu, M., Wu, Z., Wang, Z., Guo, S., Wehner, B., Nowak, A., Achtert, P., Wiedensohler, A., Jung, J., Kim, Y. J., and Liu, S.: Characteristics of aerosol size distributions and new particle formation in the summer in Beijing, J. Geophys. Res.-Atmos., 114, 1–13, https://doi.org/10.1029/2008JD010894, 2009.
Zhang, R., Khalizov, A., Wang, L., Hu, M., and Xu, W.: Nucleation and Growth of Nanoparticles in the Atmosphere, Chem. Rev., 112, 1957–2011, https://doi.org/10.1021/cr2001756, 2012.
Zhang, R., Wang, G., Guo, S., Zamora, M. L., Ying, Q., Lin, Y., Wang, W., Hu, M., and Wang, Y.: Formation of Urban Fine Particulate Matter, Chem. Rev., 115, 3803–3855, https://doi.org/10.1021/acs.chemrev.5b00067, 2015.
Zhang, Y., Tao, J., Ma, N., Kuang, Y., Wang, Z., Cheng, P., Xu, W., Yang, W., Zhang, S., Xiong, C., Dong, W., Xie, L., Sun, Y., Fu, P., Zhou, G., Cheng, Y., and Su, H.: Predicting cloud condensation nuclei number concentration based on conventional measurements of aerosol properties in the North China Plain, Sci. Total Environ., 719, 137473, https://doi.org/10.1016/j.scitotenv.2020.137473, 2020.
Zhou, Y., Dada, L., Liu, Y., Fu, Y., Kangasluoma, J., Chan, T., Yan, C., Chu, B., Daellenbach, K. R., Bianchi, F., Kokkonen, T. V., Liu, Y., Kujansuu, J., Kerminen, V. M., Petäjä, T., Wang, L., Jiang, J., and Kulmala, M.: Variation of size-segregated particle number concentrations in wintertime Beijing, Atmos. Chem. Phys., 20, 1201–1216, https://doi.org/10.5194/acp-20-1201-2020, 2020.