the Creative Commons Attribution 4.0 License.
the Creative Commons Attribution 4.0 License.
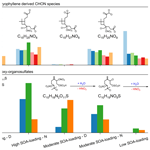
Impact of biogenic secondary organic aerosol (SOA) loading on the molecular composition of wintertime PM2.5 in urban Tianjin: an insight from Fourier transform ion cyclotron resonance mass spectrometry
Shujun Zhong
Shuang Chen
Junjun Deng
Yanbing Fan
Qiang Zhang
Qiaorong Xie
Yulin Qi
Libin Wu
Xiaodong Li
Chandra Mouli Pavuluri
Jialei Zhu
Xin Wang
Xiaole Pan
Zifa Wang
Yisheng Xu
Haijie Tong
Yafang Cheng
Kimitaka Kawamura
Biomass burning is one of the key sources of urban aerosols in the North China Plain, especially during winter, when the impact of secondary organic aerosols (SOAs) formed from biogenic volatile organic compounds (BVOCs) is generally considered to be minor. However, little is known about the influence of biogenic SOA loading on the molecular composition of wintertime organic aerosols. Here, we investigated the water-soluble organic compounds in fine particulate matter (PM2.5) from urban Tianjin by ultrahigh-resolution Fourier transform ion cyclotron resonanc mass spectrometry (FT-ICR MS). Our results show that most of the CHO and CHON compounds are derived from biomass burning which are poor in oxygen and contain aromatic rings that probably contribute to light-absorbing brown carbon (BrC) chromophores. Under moderate to high SOA-loading conditions, the nocturnal chemistry is more efficient than photooxidation to generate secondary CHO and CHON compounds with high oxygen content. Under low SOA loading, secondary CHO and CHON compounds with low oxygen content are mainly formed by photochemistry. Secondary CHO compounds are mainly derived from oxidation of monoterpenes. However, nocturnal chemistry may be more productive to sesquiterpene-derived CHON compounds. In contrast, the number- and intensity-weight of S-containing groups (CHOS and CHONS) increased significantly with the increase of biogenic SOA loading, which agrees with the fact that a majority of the S-containing groups are identified as organosulfates (OSs) and nitrooxy–organosulfates (nitrooxy–OSs) that are derived from the oxidation of BVOCs. Terpenes may be potential major contributors to organosulfates and nitrooxy–organosulfates. While the nocturnal chemistry is more beneficial to the formation of organosulfates and nitrooxy–organosulfates under low SOA loading. The SOA loading is an important factor that is associated with the oxidation degree, nitrate group content and chemodiversity of nitrooxy-organosulfates. Furthermore, our study suggests that the hydrolysis of nitrooxy-organosulfates is a possible pathway for the formation of organosulfates.
- Article
(7981 KB) -
Supplement
(14615 KB) - BibTeX
- EndNote
Organic aerosols account for about 20 %–90 % of fine particulate matter (PM2.5) in the ambient air (Fan et al., 2016; Kleindienst et al., 2007; Goldstein and Galbally, 2007). Various emission sources, including industrial production, coal combustion, traffic emissions, cooking, biomass burning, as well as primary bioaerosol, influence the formation of haze pollution in the North China Plain (Sun et al., 2013; Fan et al., 2016; Huang et al., 2021). Among these emission sources, biomass burning plays a prominent role in air quality and climate change. Biomass burning emissions include high concentrations of primary organic aerosol (POA), semivolatile organic compounds (SVOCs), and volatile organic compounds (VOCs; Koss et al., 2018). Humic-like substances (HULIS) account for 28 %–34 % of organic carbon (OC) in the PM2.5 emitted by incomplete combustion of solid biomass fuels in domestic stoves (Lin et al., 2010; Park and Yu, 2016). Domestic biomass combustion is also one of the key emission sources of HULIS, especially during winter and spring in the North China Plain (Li et al., 2019). Previous studies have revealed that biomass-burning particles contain plenty of important chromophores such as nitroaromatics and N-heterocyclic compounds, which can enhance the light absorption of aerosols (Wang et al., 2019). A recent study revealed that most of the light absorption of brown carbon (BrC) is related to biomass-burning particles, with a small amount from biogenic SOAs (Washenfelder et al., 2015). There are significant high columnar light-absorbing levels in areas with frequent biomass-burning activities (Arola et al., 2011). Therefore, biomass burning has been identified as an important contributor to atmospheric BrC (Yan et al., 2020; Saleh et al., 2014; Lin et al., 2016; Yue et al., 2022). Furthermore, large amounts of isoprene, toluene, propylene, and O3 precursors have been measured with high potential values of total ozone formation from biomass-burning activities such as wheat-straw burning in northern China (Zhu et al., 2016; Fu et al., 2012).
Secondary organic aerosols (SOAs) are the main products of the oxidation of volatile organic compounds (VOCs) in the presence of OH and NO3 radicals, O3, and other oxidants (Hallquist et al., 2009; Nie et al., 2022; Gentner et al., 2017). Organosulfates (OSs, ROS(O)2OH, esters of sulfuric acid) have been found to be the most abundant organosulfur compounds in atmospheric particulate matter, contribute significantly to the mass of SOAs, and play an important role in their formation pathways (Tolocka and Turpin, 2012; Brüggemann et al., 2020; Surratt et al., 2008; Fan et al., 2022). Moreover, nitrooxy–organosulfates (nitrooxy–OSs), which contain both nitrooxy (-ONO2) and the sulfate ester group (-OSO3H), also contribute greatly to the formation of SOAs (Brüggemann et al., 2020; Surratt et al., 2008; Xie et al., 2022). Previous studies have shown that both organosulfates and nitrooxy–organosulfates are mostly assumed to be formed by multiphase reactions between acidic sulfate particles and organic compounds from both biogenic and anthropogenic sources (Surratt et al., 2008; Iinuma et al., 2007; Zhang et al., 2012; Kristensen and Glasius, 2011; Riva et al., 2016; Fan et al., 2022). Long aliphatic chain organosulfates and nitrooxy–organosulfates (C17−28) are characterized by low degrees of oxidation and unsaturation; they may act as surfactants and affect the amphiphilicity of atmospheric particles (Su et al., 2022). Vehicle emissions might be their potential source. In contrast, (nitrooxy–)organosulfates derived from biogenic VOCs (BVOCs) have characteristics of a short carbon chain (C5−10), a high degree of oxidation, and double-bond equivalent (DBE) values close to those of their biogenic precursors (Tao et al., 2014; Passananti et al., 2016). In addition, the values of aromatic-like (nitrooxy–)organosulfates are relatively low, especially the polyaromatic (nitrooxy–)organosulfates, which are considered to be mainly originated from anthropogenic emissions precursors (e.g., fireworks) (Xie et al., 2020a, b; Kundu et al., 2013). Thus, both organosulfates and nitrooxy–organosulfates are prevalent in aerosol particles. They have been identified as potential SOA markers and have substantial implications for atmospheric physicochemical processes (Kristensen and Glasius, 2011; Zhang et al., 2012; Zhu et al., 2019; Brüggemann et al., 2017; Froyd et al., 2010; Tolocka and Turpin, 2012; Surratt et al., 2008).
Tianjin is a typical coastal city in the North China Plain where agriculture is developed; it is susceptible to open biomass burning during autumn and winter, especially the agricultural fires (Fan et al., 2020). Under the effect of land and sea breeze, organic aerosols in Tianjin are greatly influenced by sea source, land source, and diurnal chemistry (Fan et al., 2020). The low-molecular-weight organic compounds in aerosols, such as diacids, aliphatic lipids (n-alkanes, fatty acids, and fatty alcohols), and sugar compounds, have been investigated in Tianjin by gas chromatography–tandem mass spectrometry (Fan et al., 2020; Pavuluri et al., 2020; Fu et al., 2008). However, there are limited studies on the molecular composition of high-molecular-weight (HMW) organic compounds in ambient aerosols in Tianjin, especially the molecular markers with complex structure such as polycyclic aromatic hydrocarbons (PAHs) and polyacids emitted from biomass burning. In addition, little is known about organic sulfur-containing compounds and the contribution of secondary transformation processes from biogenic and anthropogenic sources. Fourier transform ion cyclotron resonance mass spectrometry (FT-ICR MS) is known for its ultrahigh resolution and has been applied to characterize natural organic mixtures in cloud water, rainwater, aerosols, and smoke particles emitted from biomass burning and coal combustion (Bianco et al., 2018; Mead et al., 2015; Song et al., 2018; Wu et al., 2019; Xie et al., 2020b; Qi et al., 2022; Han et al., 2022; Chen et al., 2022). Therefore, the purpose of this study is to learn the organic molecular composition with a wide range of molecular weight in urban aerosols using FT-ICR MS and to better understand the impacts of secondary aerosol processes on the molecular diversity in winter when biomass burning is generally active in the North Plain China.
Table 1The concentrations (ng m−3) of chemical compounds in PM2.5 samples.
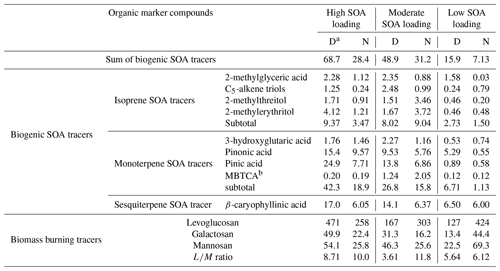
Note: a D: daytime, N: nighttime; b MBTCA: 3-methyl-1,2,3-butanetricarboxylic acid.
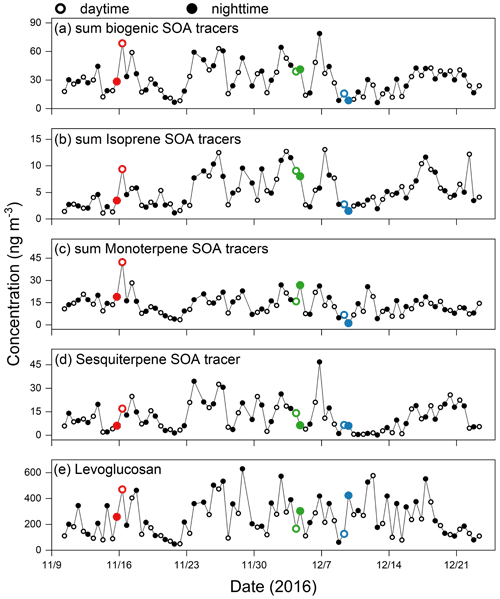
Figure 1Temporal variations in the concentrations of biogenic SOA tracers and biomass-burning tracer detected in Tianjin PM2.5 (Fan et al., 2020). (a) Sum of biogenic SOA tracers; (b) isoprene SOA tracers; (c) monoterpene SOA tracers; (d) β-caryophyllene SOA tracer. The red, green, and blue circles represent the PM2.5 samples with high, moderate, and low SOA loading, respectively.
2.1 Sample collection and organic matter isolation
In this study, we selected wintertime PM2.5 samples collected in urban Tianjin, East China, from November to December 2016, as part of the wintertime campaign of the Atmospheric Pollution and Human Health in a Chinese Megacity (APHH-Beijing) program (Shi et al., 2019). Levoglucosan is treated as the primary tracer of biomass burning (Simoneit, 2002). Isoprene and pinene are selected as the predominant BVOCs to indicate the photooxidation processes of plant and marine biogenic emission (Claeys et al., 2007; Helmig et al., 2006; Sharkey et al., 2008). Levoglucosan and biogenic SOA tracers from isoprene and terpene oxidation have been measured using a traditional gas chromatography–mass spectrometry (GC–MS). Details of these PM2.5 samples as well as molecular compositions of organic aerosols were provided in our previous study (Fan et al., 2020). Based on our previous study, we have determined six isoprene oxides, including 2-methylglyceric acid, C5-alkene triols and 2-methyltetrols, and four pinene oxides, such as 3-hydroxyglutaric acid (Fan et al., 2020). According to the sum of biogenic SOA tracers of daytime aerosols, we selected three groups that were strongly affected by biomass burning but with high, moderate, and low loadings of biogenic SOA, then selected the corresponding nocturnal ones (Table 1, Fig. 1) (i.e., high SOA loading – D: daytime sample with relatively high concentrations of SOA).
All the samples are analyzed by FT-ICR MS for the water-soluble organic compounds. The details of extraction and concentration of dissolved organic matter (DOM) from aerosol samples were taken from the previous study (Xie et al., 2020b). In short, the filter sample is sonicated in 10 mL ultrapure Milli-Q water for 10 min and repeated three times. The solution is then filtered with 0.45 µm hydrophilic PTFE filers. The extract is loaded onto a preconditioned solid-phase extraction (SPE) cartridge (Oasis HLB, Waters, US). The cartridge is dried under a pure nitrogen flow. Then, the retained organics are eluted with HPLC-grade methanol.
2.2 FT-ICR MS analysis
The isolated organic fractions are analyzed with a solariX 2XR FT-ICR instrument (Bruker Daltonik GmbH, Bremen, Germany) equipped with a 7 T superconducting magnet. Samples are ionized in negative ion mode using an electrospray ionization (ESI) ion source. For full scan mass spectra, mass spectra are acquired from 150 to 1000 (where most NOM measurements are conducted) with a transient size of 4 megawords using the quadrupolar detection mode. A total of 256 individual transients are collected and co-added to an enhanced signal-to-noise ratio, resulting in resolving power of ∼600 000 at 400. The full scan mass spectra are internally calibrated using a series of homologous compounds in DataAnalysis (Bruker Daltonics). A peak list with a signal-to-noise ratio () greater than 4 is generated. All possible formulae are calculated using Composer 15.6 (Sierra Analytics) software with a mass tolerance of ±0.5 ppm. A calculation criterion for the calculator is set as follows: C50H100O50N2S1. All the calculated formulae with DBE greater than 30 are excluded.
The relative abundance–weighted elemental ratios, AImod, MWw, and AImod,w, are calculated based on previous studies (Zhao et al., 2013; Sleighter and Hatcher, 2008; Koch and Dittmar, 2016). All assigned molecular formulae are categorized into the following five classifications according to their elemental composition: (1) combustion-derived polycyclic aromatic hydrocarbons (PAHs-like; AImod>0.66), (2) vascular plant-derived polyphenols and PAHs with aliphatic chains (Polyphenols-like; 0.50 < AImod≤0.66), (3) highly unsaturated and phenolic compounds (Phenols-like; AImod≤0.50 and ), (4) unsaturated aliphatic compounds (Aliphatics-like; ), (5) carbohydrate, saturated fatty and sulfonic acids (Carbohydrates-like; ) (Merder et al., 2020; Šantl-Temkiv et al., 2013). According to the ratio, the PAHs-like, Polyphenols-like, Phenols-like, and Aliphatics-like compounds are derived into O-poor and O-rich classes (Table S1) (Merder et al., 2020).
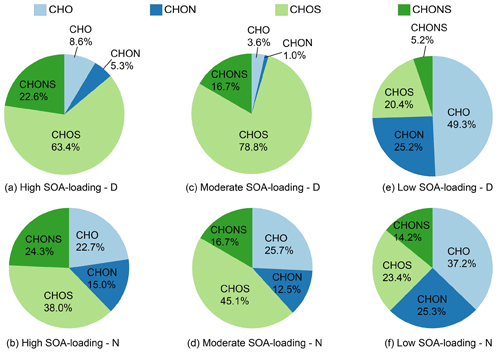
Figure 2Comparison of molecular elemental types of all PM2.5 samples. The pie chart shows the percentage of the different compound groups in various samples by intensity.
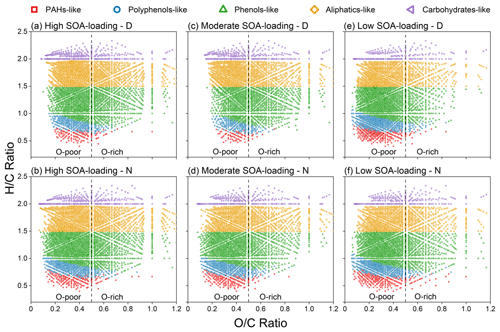
Figure 3The van Krevelen diagrams of five compounds classes in each sample: (1) PAHs-like – polycyclic aromatic hydrocarbons, (2) Polyphenols-like – polyphenols and PAHs with aliphatic chains, (3) Phenols-like – highly unsaturated and phenolic compounds, (4) Aliphatics-like – unsaturated aliphatic compounds, (5) Carbohydrates-like – carbohydrate, saturated fatty and sulfonic acids. Note: oxygen-poor compounds (), oxygen-rich compounds ().
3.1 General molecular characterization of organic aerosols
In this study, thousands of formulae (4995–6959) are obtained in each spectrum ranging from 150 to 1000 Da (Table S2). The identified molecular formulae are classified into CHO, CHON, CHOS, and CHONS components, based on their elemental compositions. For example, CHOS refers to formulae that contain C, H, O, and S elements. By comparing the number of four molecular components between all the samples, it was found that the S-containing species (CHOS and CHONS) are the most prominent components (61.8 %–96.5 %) in high and moderate SOA-loading samples, while only 25.2 %–37.6 % are present in the samples with low biogenic SOA loading (Fig. 2), suggesting that secondary transformation processes contributed significantly to S-containing compounds. Since there was little difference in the sum of biogenic SOA tracers between the nighttime samples in the moderate–high SOA-loading groups, the molecular compositions in these two groups are similar overall (Figs. 2 and 3).
The potential source of a compound class may be assessed by the ratio of the number and intensity weights of all compound classes, with a higher proportion indicating a greater contribution from the source. Aliphatics-like organics account for the highest proportion in high and moderate SOA-loading groups (43.8 %–50.5 %). In contrast, Phenols-like and Aliphatics-like contribute the most in the low biogenic SOA-loading groups (68.4 %–69.3 %). In addition, aromatic compounds (PAHs-like and Polyphenols-like) in the low biogenic SOA-loading groups (34.5 %–38.8 % and 30.5 %–33.7 %) are significantly higher than the other two groups (Figs. 3 and S1). These indicate that intense biomass burning may contribute greatly to PAHs-like, Polyphenols-like, and Phenols-like compounds. However, the increased loading of biogenic SOA contributes more saturated Aliphatics-like and Carbohydrates-like compounds.
Table S2 summarizes the number of components in each subgroup and the relative abundance–weighted elemental ratios, DBE, and modified aromaticity index (AImod) for each sample (Zhao et al., 2013; Sleighter and Hatcher, 2008). The AImod, based on heteroatoms such as oxygen, sulfur, and nitrogen, reflects C=C double-bond density to reveal the double-bond ratio to the total carbons in a molecule (Koch and Dittmar, 2006). As shown in Table S2, the AImod,w values observe similar change trends for different subgroups of each sample shown: AImod,w (CHON) > AImod,w (CHO) > AImod,w (CHOS) ≥ AImod,w (CHONS). The DBE values are also widely used to estimate the degree of unsaturation (Koch and Dittmar, 2006). In this study, the DBEw also have a similar pattern: DBEw (CHON) > DBEw (CHO) > DBEw (CHONS) > DBEw (CHOS). Trends in DBEw and AImod are similar to previous studies, such as DOM from urban aerosols and biomass-burning particulate matter (Song et al., 2018; Jiang et al., 2021). This might be because CHO and CHON compounds are mainly composed of combustion-derived highly unsaturated and phenolic compounds (Phenols-like), followed by highly aromatic PAHs-like and Polyphenols-like, while CHOS and CHONS were mostly Aliphatics-like, Phenols-like, and Carbohydrates-like with relatively high saturation (Table S3).
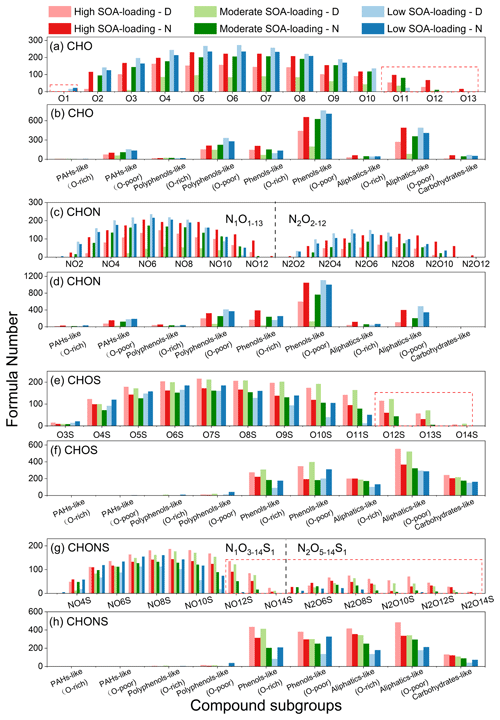
Figure 4(a, c, e, g) Classification of CHO, CHON, CHOS, and CHONS compounds into different subgroups based on the number of O and N atoms in molecules. (b, d, f, h) The number of five compound classifications of all molecules to each sample. The column is the sum of the formula number in each subgroup.
3.2 CHO compounds
The CHO compounds, which may contain carboxyl and/or hydroxyl functional groups, have been widely detected in the ESI negative mode and identified in water-soluble organic matter in aerosols and cloud water (Bianco et al., 2018; Tu et al., 2016; Xie et al., 2020a; Kourtchev et al., 2016). About 596 to 1967 ions could be assigned to CHO groups in the PM2.5 samples (Table S2). The intensity contribution of CHO compounds accounted for 3.6 %–49.3 % of the total compound in each sample, a difference of 1 order of magnitude (Fig. 2). Both the number and intensity contributions of CHO compounds decrease significantly with the increase of biogenic SOA loading, especially during the day, suggesting that biomass burning contributed greatly to the chemical diversity of CHO compounds.
As shown in Fig. 4a, the CHO compounds are classified into 13 subgroups based on their O numbers. Most of the O >10 subgroups are detected only in the samples with moderate to high biogenic SOA loading, and the number increased with SOA concentrations. These high-oxygen-containing compounds are mainly highly unsaturated Phenols-like compounds (Fig. 4b). Combined with DBE and carbon number plots (Fig. S3), these unique O-rich compounds might be lignin-like compounds containing a single benzene ring, which are particularly sensitive to the UV light (Qi et al., 2016). In contrast, CHO compounds containing one oxygen atom existed only in the low SOA-loading group. Moreover, the number of each CHO subgroup at night is much greater than that during the day in moderate–high SOA-loading groups, and the opposite is true in the low SOA-loading group. These suggest that nocturnal chemistry was more efficient than photochemistry in oxidizing and forming biogenic secondary organic aerosols with high oxygen content at moderate–high SOA loadings, while photochemistry dominates the formation of secondary CHO compounds with low oxygen content at low SOA loadings.
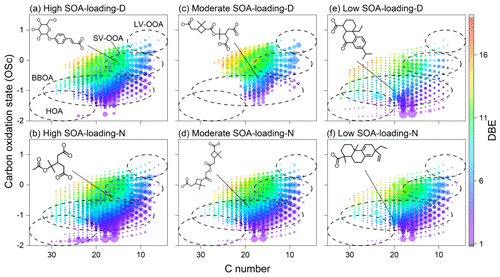
Figure 5The carbon oxidation state (OSC) versus C number of CHO compounds. The size and color bar denote the relative peak intensity and DBE value. The dashed circles are marked as SV-OOA (semi-volatile oxidized organic aerosol), LV-OOA (low-volatile oxidized organic aerosol), BBOOA (biomass-burning organic aerosol), and HOA (hydrocarbon-like organic aerosol). The formulae for biogenic SOA compounds with relatively high intensity were C15H18O8, C16H24O8, C19H28O7, C17H26O8, C20H26O3, C20H30O2, respectively. Note that the proposed structures were representative, not determined.
The carbon oxidation state (OSC) is a widely used parameter to describe the oxidation processes of complex organic mixtures (Kroll et al., 2011). The OSC values of semi-volatile and low-volatility oxidized organic aerosol (SV-OOA and LV-OOA) range from −1 to +1 and are less than 13 carbon atoms, which may be associated with multi-step oxidation reactions. The OSC values of biomass-burning organic aerosol (BBOA) is relatively low, ranging from −0.5 to −1.5, and greater than 7 carbon atoms. Molecules with OSC values less than −1 and a carbon number greater than 20 may be related to hydrocarbon-like organic aerosol (HOA). As shown in Fig. 5, the number of molecules in the SV-OOA and BBOA regions and their peak intensities increase significantly as the SOA loading increased, suggesting that the increase of SOA loading might promote the multi-step oxidation reactions. Some of the high-intensity CHO compounds, such as C19H28O7, C17H26O8, that may be typical dimers of α-pinene secondary organic aerosol as well as their homologues in the SV-OOA area, are detected in high and moderate SOA-loading samples (Fig. S6). However, the relatively high-intensity CHO compounds such as C20H26O3 and C20H30O2, which had DBE values of 8 and 6, and may be diterpenoid derivatives (dehydroabietic acid and pimaric acid), are detected in the samples with low SOA loading (Gómez-González et al., 2012; Kourtchev et al., 2014; Yasmeen et al., 2011, 2010; Kristensen et al., 2013, 2014; Müller et al., 2008). The most likely molecular structures of these α-pinene derivatives are illustrated in Fig. 5. Obviously, the oxygen content and DBE values of these biogenic secondary CHO compounds in low SOA-loading groups are significantly lower than that of the other two moderate–high SOA-loading groups. These results indicate that biogenic CHO compounds are mainly derivatives of monoterpenes, and the oxygen content of these biogenic SOAs increases significantly with the increase of SOA loading, especially monoterpene derivatives.
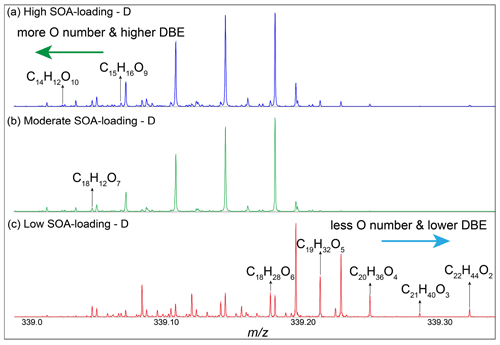
Figure 6Mass-scale-expanded segments (0.30 Da) of the broadband mass spectra for CHO compounds. The shift to higher mass defect in the low SOA-loading group – D sample reflects lower oxygen content and DBE value, and vice versa reflects higher oxygen content and DBE value in high and moderate SOA-loading groups – D sample.
In addition, the effects of natural oxidation processes can also be observed at the microscale of individual peaks in the expanded segments of the full-scan mass spectra. For example, Fig. 6 shows a ∼0.3 Da segment from three daytime samples. The unique CHO compounds are labeled between the three daytime samples, respectively. Two and one unique CHO compounds with higher oxygen content were detected in the first and second spectra and transferred to species with lower mass (Fig. 6a and b). On the contrary, there are five new CHO compounds with lower O content in the spectrum of samples in the low SOA-loading group – D, and the mass of these compounds increases with decreasing oxygen content (Fig. 6c). The DBE value increased with the increase of oxygen number and OSC, which is consistent with Fig. 5. These results indicate that secondary CHO organic aerosols have obvious bias in the formation processes. At low SOA loads, especially monoterpene derivatives, secondary CHO organic aerosols are dominated by conjugated polyene compounds with low oxygen content and high saturation, while with the increase of SOA load, multi-step oxidation formed the O-rich compounds containing monophenyl ring, which may be important light-absorbing chromophores in the atmosphere (Deng et al., 2022).
3.3 CHON compounds
Large amounts of organic nitrogen compounds are observed in all PM2.5 samples. The CHON group could be assigned to 272 to 2513 ions in all samples, whose abundance–weight contribution accounts for 1.0 %–25.3 %, being similar to CHO (Table S2, Fig. 2). Apparently, in the low SOA-loading group, the abundance–weight contribution of CHON compounds is significantly higher than that in the other two groups. Under the moderate–high SOA-loading groups, the concentration of levoglucosan, a marker of biomass burning, was about 2 times higher in the nighttime sample than that of the corresponding daytime samples (Fig. 1, Table 1), indicating that the intensity of biomass burning was relatively high at night, the same as was the abundance–weight contribution of CHON compounds. This is combined with the fact that most CHON compounds are classified as O-poor Phenols-like and Polyphenols-like compounds (Fig. 4d), which are similar to the characteristics of CHON compounds emitted from biomass materials (Song et al., 2018). Several of the highest intensity nitroaromatic CHON compounds with C numbers less than 10, such as C7H7N1O4, C8H9N1O3, C8H7N1O5, C9H9N1O5, C7H5N1O4, are detected in particulate matter emitted from combustion processes and potential contributors to light absorption as BrC chromophores (Song et al., 2018; Desyaterik et al., 2013; Yan et al., 2020; Iinuma et al., 2010) (Fig. S4). The CHON compounds are classified into 24 subgroups based on their N and O numbers, including N1On (N1O1 – N1O13) and N2On (N2O2 – N2O12) subgroups (Fig. 4c); 80 %–100 % of the CHON compounds have . Hence, it can be inferred that most of CHON compounds in this study contained oxidized nitrogen functional groups such as nitro- (-NO2) and/or organonitrates (-ONO2). These results suggest that CHON compounds might be mainly derived from biomass burning, such as nitrophenols, nitrocatechols, nitroguaiacols, and nitrosalicylic acids, which has also been observed in previous studies (Kourtchev et al., 2015; Zhang et al., 2013; Song et al., 2018).
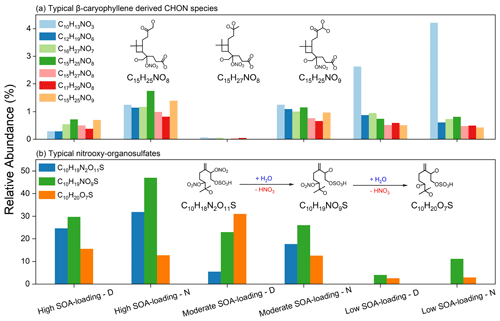
Figure 7(a) Relative abundance distributions of typical CHON compounds derived from β-caryophyllene. Some of the proposed chemical structures have been reported in a previous study (Chan et al., 2010). (b) Relative abundance distributions of typical nitrooxy–organosulfates. The hydrolysis reactions and the proposed chemical structures have been reported in a previous study (Lin et al., 2012).
The typical α-pinene and isoprene SOA components such as C10H14N1O5, C6H14N1O7, and C5H7N1O4 (Perraud et al., 2010; Ng et al., 2008) are not detected in all samples, suggesting that isoprene and monoterpene may not contribute significantly to secondary CHON aerosols but sesquiterpenes might. Figure 7a shows the peak intensity distributions of seven nitrogen-containing SOAs from β-caryophyllene (i.e., C10H13N1O3, C12H19N1O6, C16H27N1O7, C15H25N1O8, C15H27N1O8, C17H29N1O8, and C15H25N1O9) (Chan et al., 2010). The moderate SOA-loading – D sample with highest concentrations of sesquiterpene SOAs have the lowest relative abundance. In particular, the relative abundance of C10H13N1O3 in the low SOA-loading – N sample is about 3.5 times that of the high SOA-loading – N sample. The relative abundance of these compounds in the nighttime samples is about twice that of the daytime samples. All these nitrogen-containing SOA compounds are detected in the series of β-caryophyllene/NOx irradiation experiments (Chan et al., 2010), but our study demonstrates that nocturnal chemistry might be more conducive to sesquiterpene SOA compound formation, especially under low sesquiterpene-loading conditions.
3.4 CHOS compounds
In our PM2.5 samples, about 853 to 1663 ions are identified as CHOS, and the intensity contribution ranges from 20.4 % to 78.8 % (Fig. 2, Table S2). Their intensity contribution in the low SOA-loading samples (20.4 %–23.4 %) is lower than those of the moderate–high biogenic SOA-loading groups (38.0 %–78.8 %). The intensity contribution of daytime samples is 23.9 %–25.4 % higher than that of corresponding nighttime samples at moderate SOA loads (Fig. 2a–d), while it was opposite under the low SOA loads, with a 3 % higher intensity contribution at night (Fig. 2e and f). As shown in Table S2, the number of CHOS compounds is 853 in the low SOA-loading – D sample, which nearly doubles with increase of SOA loads. The average of AImod,w and DBEw values of CHOS compounds are significantly lower than that of CHO and CHON categories, and also much lower than that of CHOS generated by the combustion of coal (0.31) and biomass materials (0.13–0.18) (Song et al., 2018). As shown in Fig. 4e, the identified CHOS formulae are O3S-O14S class species, with O6−9S being the most abundant. Interestingly, almost all the CHOS formulae had ratios ≥4, and these CHOS compounds are tentatively regarded as organosulfates (OSs). The sulfate group (OSO3H) carries four O atoms and readily deprotonates by ESI(–); it has been identified as contributing significantly to the generation of SOA (Wang et al., 2016; Lin et al., 2012; Tolocka and Turpin, 2012). Furthermore, the most abundant CHOS compounds such as C15H24O7S, C15H28O7S, C15H26O7S, C10H18O6S, C9H16O6S, C10H18O7S, etc., and their corresponding homologues were detected (Figs. 8, S5 and S6), which are generated by the oxidation of isoprene, monoterpene, and sesquiterpene, respectively (Riva et al., 2016; Passananti et al., 2016; Surratt et al., 2008; Chan et al., 2010). These data indicate that the majority of CHOS compounds are derived primarily from the oxidation of BVOCs, and that the formation efficiency of nocturnal chemistry and photochemistry varies with biogenic SOA loads.
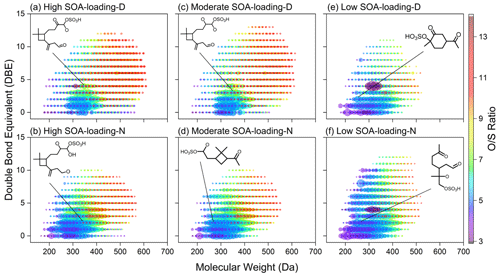
Figure 8DBE versus molecular weight of CHOS compounds. The color bar and marker size denote the ratios and the relative peak magnitudes of CHOS compounds. The formulae for biogenic SOA compounds with relatively high intensity were C15H24O7S, C15H28O7S, C15H26O7S, C10H18O6S, C9H16O6S, and C10H18O7S. Note that the proposed structures were representative, not determined.
About half of CHOS compounds are Aliphatics-like compounds, followed by Phenols-like and Carbohydrates-like compounds with low aromatic degree (Fig. 4f). Not only the total formulae number, but also the number of each CHOS subgroup in the moderate–high SOA-loading daytime samples are significantly higher than that in the corresponding nighttime samples. In contrast, in the case of low biogenic SOA loading, it is opposite. Interestingly, the O ≥12 organosulfates are identified only in the moderate–high biogenic SOA-loading groups, and the formula number is even slightly higher in the moderate SOA-loading group than that in high biogenic SOA-loading group (Fig. 4e). Additionally, 305 to 560 OS compounds with high ratios (≥10) are found to be densely distributed in the moderate–high biogenic SOA-loading groups compared to the samples with low SOA loads, particularly in the region of high molecular weight (HMW >500 Da) (Fig. 8). Similarly, the number of these HMW OSs is 1.6–2.3 times higher in the daytime samples with moderate–high biogenic SOA loads than in the nighttime samples, but only 33 % in the low SOA loads. Apparently, these high-oxygen-containing compounds are mainly composed of O-rich Phenols-like and Aliphatics-like compounds (Fig. 4f), unlike long-chain aliphatic organosulfates with a few or no additional functional groups which are emitted from traffic (Tao et al., 2014). Combined with a DBE value and C number (Figs. 8 and S5), it could be inferred that these O-rich species might have alicyclic alkane organosulfates containing conjugated polyene, similar to organosulfates derived from the oxidation of BVOCs (Chan et al., 2010; Surratt et al., 2008). The differences between daytime and nighttime aerosols indicate that photochemical oxidation should be more beneficial for multi-step oxidation of biogenic organosulfates when the biogenic SOA loading is relatively high (Fig. S6). On the other hand, organosulfates are more likely to be generated by nocturnal chemistry when the biogenic SOA loading is low.
In addition, it should be noted that C5 organosulfates (C5H10O5S, C5H10O6S and C5H12O7S, etc.) typically associated with isoprene (C5H8) in laboratory studies (Surratt et al., 2008; Chan et al., 2010) are not observed in all samples. Although the corresponding C6−9 isoprene-related organosulfate homologues are detected, their relative abundance is low (Figs. 8 and S5). In addition, the concentrations of isoprene and sesquiterpene SOA tracers were similar in the moderate–high SOA-loading groups, but monoterpene SOA differed greatly. These results suggest that isoprene may be a relatively minor contributor to the population of organosulfates in winter, and monoterpene might be the potential contributor to the high-oxygen-containing OSs.
3.5 CHONS compounds
The intensity of CHONS compounds accounts for 5.2 %–24.3 % of total compounds in all PM2.5 samples. Both the intensity weighted and number increased with the increase of biogenic SOA loads (Fig. 2, Table S2). The average ratios of CHONS are much higher than other subgroups (Table S2), which is consistent with S atoms in molecules, indicating that the oxidation time of CHONS compounds is longer or the oxidation efficiency is higher (Altieri et al., 2009). With the increase of biogenic SOA loading, the number of CHONS compounds increases dramatically by 1294, implying that biogenic SOA contributes significantly to the chemical diversity of CHONS compounds. Similar to CHOS compounds, the total number of CHONS compounds in the moderate–high SOA-loading daytime samples is 436 more than in the nighttime samples, whereas in the low SOA-loading group, the number of CHONS compounds in the daytime sample is 55 % than in the nighttime sample.
Based on the N and O atoms, CHONS compounds were classified into 22 subgroups, including N1OnS1 (N1O3S1-N1O14S1) and N2OnS1 (N2O5S1-N2O14S1) (Fig. 4g). It should be noted that more than 70 % of N1OnS1 formulae contain 7 or more O atoms, and about 50 % of N2OnS1 formulae have fewer than 10 O atoms, implying that these CHONS compounds are probably nitrooxy–organosulfates (nitrooxy–OSs) containing nitrate (-ONO2) groups (Fig. 4g). The CHONS compounds are mainly Phenols-like and Aliphatics-like, followed by Carbohydrates-like (Fig. 4h), suggesting that these compounds might contain long alkyl carbon chains character. Similar to CHOS compounds, CHONS compounds might be formed primarily by the secondary conversion processes of VOCs at high concentrations of nitrogen oxide (NOx) (Surratt et al., 2008; Kundu et al., 2013). The total formulae number and the number of each CHONS subgroup of daytime samples are both higher than that of corresponding nighttime samples at moderate and high SOA loads, while the number is opposite at low SOA loads (Fig. 4g and h), suggesting that nocturnal chemistry is more conducive to nitrooxy–OSs generation at low biogenic SOA loads, while photochemistry is more efficient for the formation of nitrooxy–OSs with the increase of biogenic SOA loads.
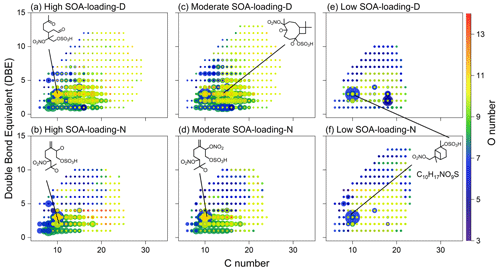
Figure 9Double-bond equivalent (DBE) versus C number for all the CHONS compounds. The color bar and marker size denote the number of O atoms and the relative peak intensities of molecular formulae on a logarithmic scale. Note that the proposed structure is representative, not determined.
Figure 9 shows the DBE, C, and O atomic distributions in the CHONS compounds. The most abundant nitrooxy–OSs – C10H17N1O7S1, C10H17N1O9S1, C10H19N1O9S1, C15H25N1O7S1, C10H18N2O11S1 – which are generated by the oxidation of α-terpinene, α, β-pinene, β-caryophyllene, and terpinolene in atmosphere and smog-chamber experiments (Altieri et al., 2009; Kundu et al., 2013; Lin et al., 2012; Surratt et al., 2008; Chan et al., 2010; Wang et al., 2021), as well as the corresponding homologues (Fig. S6), are detected in all samples, highlighting the importance of BVOCs to form CHONS compounds. It is worth noting that in the two low SOA-loading samples, the highest abundance of nitrooxy–OSs with 294.0653 and the molecular formula C10H17N1O7S1 might be formed by the oxidation of α-pinene in the presence of SO2 and NOx (Kundu et al., 2013; Altieri et al., 2009), suggesting that CHONS compounds might be mainly derived from the oxidation of monoterpene when SOA loading is low, especially pinonic acid and pinic acid.
Interestingly, most of the N1O12−14S1 and N2OnS1 species were detected only in the moderate and high SOA-loading samples, and the number of these compounds in daytime samples was more abundant than in the nighttime samples (Fig. 4g). According to Table 1, the sum concentrations of isoprene SOA tracers in the moderate and high SOA-loading groups are similar, but the concentrations of monoterpene SOA tracers were much different. Most of the relatively high-abundance CHONS compounds are monoterpene nitrooxy–OSs. Therefore, our results indicate that the load of biogenic SOAs is an important factor determining the oxidation degree, nitrate (-ONO2) content, and chemical diversity of CHONS compounds.
In particular, previous studies have shown that nitrate (-ONO2) and/or sulfate (-OSO3H) might undergo hydrolysis in the presence of atmospheric water (Lin et al., 2012; Liu et al., 2012; Hu et al., 2011). Figure 7b shows one of the hydrolysis reactions of nitrooxy–OSs with relatively high abundance. Obviously, the terpene-related nitrooxy–OSs (C10H18N2O11S1) were only observed in the moderate and high SOA-loading groups. In the presence of water, the terpene-related nitrooxy–OSs substitutes the nitrate group with hydroxyl groups by hydrolysis. Therefore, it can be inferred that the corresponding CHOS and CHONS (N=1) organosulfates in the studied samples may be generated through the hydrolysis of nitrooxy–OSs.
Four categories of organic compounds, including CHO, CHON, CHOS, and CHONS species were determined by ultrahigh-resolution FT-ICR MS in the urban Tianjin during winter. Biomass burning was found to contribute significantly to CHO and CHON compounds; most of the them are O-poor and highly unsaturated PAHs and (Poly)phenols, which are important light-absorbing chromophores in the atmosphere. There is a significant change for both the number and abundance–weight contribution between daytime and nighttime samples at different biogenic SOA loadings. The nocturnal chemistry is more efficient than photochemistry in oxidizing and forming secondary CHO and CHON compounds with high oxygen content at moderate–high SOA loadings, while photochemistry dominates the formation of secondary CHO and CHON compounds with low oxygen content at low SOA loadings. The biogenic CHO compounds are mainly derivatives of monoterpenes. However, nocturnal chemistry might be more conducive to sesquiterpene SOA formation, especially under low sesquiterpene-loading conditions.
The S-containing compounds (CHOS and CHONS) are mainly derived from the oxidation of BVOCs. About 96 % of S-containing compounds are considered as organosulfates and nitrooxy–organosulfates. Compared with CHO and CHON compounds, high abundances of S-containing compounds with higher ratio and lower DBE and AImod values are mainly composed of by alicyclic alkane organosulfates. With the increasing SOA loading, the contribution of the number and abundance–weight contribution of S-containing compounds increased dramatically. The nocturnal chemistry is more conducive to nitrooxy–OSs generation at low biogenic SOA loadings, while photochemistry is more efficient for nitrooxy–OSs formation with the increase of biogenic SOA. Monoterpenes might be potential contributors to high-oxygen-content organosulfates. Our results show that the biogenic SOA is an important factor determining the oxidation degree, nitrate (-ONO2) content and chemical diversity of S-containing compounds in urban Tianjin. Moreover, some of the CHOS and CHON1S organosulfates can also be formed by the hydrolysis of the nitrate group of nitrooxy–organosulfates.
The data are available upon request from the corresponding author.
The supplement related to this article is available online at: https://doi.org/10.5194/acp-23-2061-2023-supplement.
PF designed the study. SZ, SC, JD, YF, and QX carried out the experiments and performed the data analysis. SZ prepared the first version of the manuscript with contributions from all co-authors. All authors verified the final version of the manuscript.
At least one of the (co-)authors is a member of the editorial board of Atmospheric Chemistry and Physics. The peer-review process was guided by an independent editor, and the authors also have no other competing interests to declare.
Publisher's note: Copernicus Publications remains neutral with regard to jurisdictional claims in published maps and institutional affiliations.
The authors greatly appreciate the assistance of the editor and three anonymous reviewers for the helpful comments that greatly improved the quality of this paper.
This research has been supported by the National Natural Science Foundation of China (grant nos. 42130513 and 41905110) and the Strategic Priority Research Program of the Chinese Academy of Sciences (grant no. XDA23020301).
This paper was edited by Aijun Ding and reviewed by three anonymous referees.
Altieri, K. E., Turpin, B. J., and Seitzinger, S. P.: Oligomers, organosulfates, and nitrooxy organosulfates in rainwater identified by ultra-high resolution electrospray ionization FT-ICR mass spectrometry, Atmos. Chem. Phys., 9, 2533–2542, https://doi.org/10.5194/acp-9-2533-2009, 2009.
Arola, A., Schuster, G., Myhre, G., Kazadzis, S., Dey, S., and Tripathi, S. N.: Inferring absorbing organic carbon content from AERONET data, Atmos. Chem. Phys., 11, 215–225, https://doi.org/10.5194/acp-11-215-2011, 2011.
Bianco, A., Deguillaume, L., Vaitilingom, M., Nicol, E., Baray, J. L., Chaumerliac, N., and Bridoux, M.: Molecular Characterization of Cloud Water Samples Collected at the Puy de Dome (France) by Fourier Transform Ion Cyclotron Resonance Mass Spectrometry, Environ. Sci. Technol., 52, 10275–10285, https://doi.org/10.1021/acs.est.8b01964, 2018.
Brüggemann, M., Poulain, L., Held, A., Stelzer, T., Zuth, C., Richters, S., Mutzel, A., van Pinxteren, D., Iinuma, Y., Katkevica, S., Rabe, R., Herrmann, H., and Hoffmann, T.: Real-time detection of highly oxidized organosulfates and BSOA marker compounds during the F-BEACh 2014 field study, Atmos. Chem. Phys., 17, 1453–1469, https://doi.org/10.5194/acp-17-1453-2017, 2017.
Brüggemann, M., Xu, R., Tilgner, A., Kwong, K. C., Mutzel, A., Poon, H. Y., Otto, T., Schaefer, T., Poulain, L., Chan, M. N., and Herrmann, H.: Organosulfates in Ambient Aerosol: State of Knowledge and Future Research Directions on Formation, Abundance, Fate, and Importance, Environ. Sci. Technol., 54, 3767–3782, https://doi.org/10.1021/acs.est.9b06751, 2020.
Chan, M. N., Surratt, J. D., Chan, A. W. H., Schilling, K., Offenberg, J. H., Lewandowski, M., Edney, E. O., Kleindienst, T. E., Jaoui, M., Edgerton, E. S., Tanner, R. L., Shaw, S. L., Zheng, M., Knipping, E. M., and Seinfeld, J. H.: Influence of aerosol acidity on the chemical composition of secondary organic aerosol from β-caryophyllene, Atmos. Chem. Phys., 11, 1735–1751, https://doi.org/10.5194/acp-11-1735-2011, 2011.
Chen, S., Xie, Q. R., Su, S. H., Wu, L. B., Zhong, S. J., Zhang, Z. M., Ma, C., Qi, Y. L., Hu, W., Deng, J. J., Ren, L. J., Zhu, D. Q., Guo, Q. J., Liu, C. Q., Jang, K. S., and Fu, P. Q.: Source and formation process impact the chemodiversity of rainwater dissolved organic matter along the Yangtze River Basin in summer, Water Res., 211, 118024, https://doi.org/10.1016/j.watres.2021.118024, 2022.
Claeys, M., Szmigielski, R., Kourtchev, I., van der Veken, P., Vermeylen, R., Maenhaut, W., Jaoui, M., Kleindienst, T. E., Lewandowski, M., Offenberg, J. H., and Edney, E. O.: Hydroxydicarboxylic acids: markers for secondary organic aerosol from the photooxidation of alpha-pinene, Environ. Sci. Technol., 41, 1628–1634, https://doi.org/10.1021/es0620181, 2007.
Deng, J., Ma, H., Wang, X., Zhong, S., Zhang, Z., Zhu, J., Fan, Y., Hu, W., Wu, L., Li, X., Ren, L., Pavuluri, C. M., Pan, X., Sun, Y., Wang, Z., Kawamura, K., and Fu, P.: Measurement report: Optical properties and sources of water-soluble brown carbon in Tianjin, North China – insights from organic molecular compositions, Atmos. Chem. Phys., 22, 6449–6470, https://doi.org/10.5194/acp-22-6449-2022, 2022.
Desyaterik, Y., Sun, Y., Shen, X., Lee, T., Wang, X., Wang, T., and Collett Jr., J. L.: Speciation of “brown” carbon in cloud water impacted by agricultural biomass burning in eastern China, J. Geophys. Res.-Atmos., 118, 7389–7399, https://doi.org/10.1002/jgrd.50561, 2013.
Fan, W. L., Chen, T., Zhu, Z. L., Zhang, H., Qiu, Y. L., and Yin, D. Q.: A review of secondary organic aerosols formation focusing on organosulfates and organic nitrates, J. Hazard. Mater., 430, 128406, https://doi.org/10.1016/j.jhazmat.2022.128406, 2022.
Fan, X., Wei, S., Zhu, M., Song, J., and Peng, P.: Comprehensive characterization of humic-like substances in smoke PM2.5 emitted from the combustion of biomass materials and fossil fuels, Atmos. Chem. Phys., 16, 13321–13340, https://doi.org/10.5194/acp-16-13321-2016, 2016.
Fan, Y., Liu, C.-Q., Li, L., Ren, L., Ren, H., Zhang, Z., Li, Q., Wang, S., Hu, W., Deng, J., Wu, L., Zhong, S., Zhao, Y., Pavuluri, C. M., Li, X., Pan, X., Sun, Y., Wang, Z., Kawamura, K., Shi, Z., and Fu, P.: Large contributions of biogenic and anthropogenic sources to fine organic aerosols in Tianjin, North China, Atmos. Chem. Phys., 20, 117–137, https://doi.org/10.5194/acp-20-117-2020, 2020.
Froyd, K. D., Murphy, S. M., Murphy, D. M., de Gouw, J. A., Eddingsaas, N. C., and Wennberg, P. O.: Contribution of isoprene-derived organosulfates to free tropospheric aerosol mass, P. Natl. Acad. Sci. USA, 107, 21360–21365, https://doi.org/10.1073/pnas.1012561107, 2010.
Fu, P. Q., Kawamura, K., Okuzawa, K., Aggarwal, S. G., Wang, G. H., Kanaya, Y., and Wang, Z. F.: Organic molecular compositions and temporal variations of summertime mountain aerosols over Mt. Tai, North China Plain, J. Geophys. Res.-Atmos., 113, D19107, https://doi.org/10.1029/2008JD009900, 2008.
Fu, P. Q., Kawamura, K., Chen, J., Li, J., Sun, Y. L., Liu, Y., Tachibana, E., Aggarwal, S. G., Okuzawa, K., Tanimoto, H., Kanaya, Y., and Wang, Z. F.: Diurnal variations of organic molecular tracers and stable carbon isotopic composition in atmospheric aerosols over Mt. Tai in the North China Plain: an influence of biomass burning, Atmos. Chem. Phys., 12, 8359–8375, https://doi.org/10.5194/acp-12-8359-2012, 2012.
Gentner, D. R., Jathar, S. H., Gordon, T. D., Bahreini, R., Day, D. A., El Haddad, I., Hayes, P. L., Pieber, S. M., Platt, S. M., de Gouw, J., Goldstein, A. H., Harley, R. A., Jimenez, J. L., Prevot, A. S. H., and Robinson, A. L.: Review of Urban Secondary Organic Aerosol Formation from Gasoline and Diesel Motor Vehicle Emissions, Environ. Sci. Technol., 51, 1074–1093, https://doi.org/10.1021/acs.est.6b04509, 2017.
Goldstein, A. H. and Galbally, I. E.: Known and unexplored organic constituents in the Earth's atmosphere, Environ. Sci. Technol., 41, 1514–1521, 2007.
Gómez-González, Y., Wang, W., Vermeylen, R., Chi, X., Neirynck, J., Janssens, I. A., Maenhaut, W., and Claeys, M.: Chemical characterisation of atmospheric aerosols during a 2007 summer field campaign at Brasschaat, Belgium: sources and source processes of biogenic secondary organic aerosol, Atmos. Chem. Phys., 12, 125–138, https://doi.org/10.5194/acp-12-125-2012, 2012.
Hallquist, M., Wenger, J. C., Baltensperger, U., Rudich, Y., Simpson, D., Claeys, M., Dommen, J., Donahue, N. M., George, C., Goldstein, A. H., Hamilton, J. F., Herrmann, H., Hoffmann, T., Iinuma, Y., Jang, M., Jenkin, M. E., Jimenez, J. L., Kiendler-Scharr, A., Maenhaut, W., McFiggans, G., Mentel, Th. F., Monod, A., Prévôt, A. S. H., Seinfeld, J. H., Surratt, J. D., Szmigielski, R., and Wildt, J.: The formation, properties and impact of secondary organic aerosol: current and emerging issues, Atmos. Chem. Phys., 9, 5155–5236, https://doi.org/10.5194/acp-9-5155-2009, 2009.
Han, H. X., Feng, Y. J., Chen, J., Xie, Q. R., Chen, S., Sheng, M., Zhong, S. J., Wei, W., Su, S. H., and Fu, P. Q.: Acidification impacts on the molecular composition of dissolved organic matter revealed by FT-ICR MS, Sci. Total Environ., 805, https://doi.org/10.1016/j.scitotenv.2021.150284, 2022.
Helmig, D., Ortega, J., Guenther, A., Herrick, J. D., and Geron, C.: Sesquiterpene emissions from loblolly pine and their potential contribution to biogenic aerosol formation in the Southeastern US, Atmos. Environ., 40, 4150–4157, https://doi.org/10.1016/j.atmosenv.2006.02.035, 2006.
Hu, K. S., Darer, A. I., and Elrod, M. J.: Thermodynamics and kinetics of the hydrolysis of atmospherically relevant organonitrates and organosulfates, Atmos. Chem. Phys., 11, 8307–8320, https://doi.org/10.5194/acp-11-8307-2011, 2011.
Huang, S., Hu, W., Chen, J., Wu, Z. J., Zhang, D. Z., and Fu, P. Q.: Overview of biological ice nucleating particles in the atmosphere, Environ. Int., 146, 106197, https://doi.org/10.1016/j.envint.2020.106197, 2021.
Iinuma, Y., Müller, C., Berndt, T., Böge, O., Claeys, M., and Herrmann, H.: Evidence for the Existence of Organosulfates from β-Pinene Ozonolysis in Ambient Secondary Organic Aerosol, Environ. Sci. Technol., 41, 6678–6683, https://doi.org/10.1021/es070938t, 2007.
Iinuma, Y., Boge, O., Grafe, R., and Herrmann, H.: Methyl-nitrocatechols: atmospheric tracer compounds for biomass burning secondary organic aerosols, Environ. Sci. Technol., 44, 8453–8459, https://doi.org/10.1021/es102938a, 2010.
Jiang, H., Li, J., Sun, R., Tian, C., Tang, J., Jiang, B., Liao, Y., Chen, C. E., and Zhang, G.: Molecular Dynamics and Light Absorption Properties of Atmospheric Dissolved Organic Matter, Environ. Sci. Technol., 55, 10268–10279, https://doi.org/10.1021/acs.est.1c01770, 2021.
Kleindienst, T. E., Jaoui, M., Lewandowski, M., Offenberg, J. H., Lewis, C. W., Bhave, P. V., and Edney, E. O.: Estimates of the contributions of biogenic and anthropogenic hydrocarbons to secondary organic aerosol at a southeastern US location, Atmos. Environ., 41, 8288–8300, https://doi.org/10.1016/j.atmosenv.2007.06.045, 2007.
Koch, B. P. and Dittmar, T.: From mass to structure: an aromaticity index for high-resolution mass data of natural organic matter, Rapid Commun. Mass Spectrom., 20, 926–932, https://doi.org/10.1002/rcm.2386, 2006.
Koch, B. P. and Dittmar, T.: From mass to structure: an aromaticity index for high-resolution mass data of natural organic matter, Rapid Commun. Mass Spectrom., 30, 250–250, https://doi.org/10.1002/rcm.7433, 2016.
Koss, A. R., Sekimoto, K., Gilman, J. B., Selimovic, V., Coggon, M. M., Zarzana, K. J., Yuan, B., Lerner, B. M., Brown, S. S., Jimenez, J. L., Krechmer, J., Roberts, J. M., Warneke, C., Yokelson, R. J., and de Gouw, J.: Non-methane organic gas emissions from biomass burning: identification, quantification, and emission factors from PTR-ToF during the FIREX 2016 laboratory experiment, Atmos. Chem. Phys., 18, 3299–3319, https://doi.org/10.5194/acp-18-3299-2018, 2018.
Kourtchev, I., Fuller, S. J., Giorio, C., Healy, R. M., Wilson, E., O'Connor, I., Wenger, J. C., McLeod, M., Aalto, J., Ruuskanen, T. M., Maenhaut, W., Jones, R., Venables, D. S., Sodeau, J. R., Kulmala, M., and Kalberer, M.: Molecular composition of biogenic secondary organic aerosols using ultrahigh-resolution mass spectrometry: comparing laboratory and field studies, Atmos. Chem. Phys., 14, 2155–2167, https://doi.org/10.5194/acp-14-2155-2014, 2014.
Kourtchev, I., Doussin, J.-F., Giorio, C., Mahon, B., Wilson, E. M., Maurin, N., Pangui, E., Venables, D. S., Wenger, J. C., and Kalberer, M.: Molecular composition of fresh and aged secondary organic aerosol from a mixture of biogenic volatile compounds: a high-resolution mass spectrometry study, Atmos. Chem. Phys., 15, 5683–5695, https://doi.org/10.5194/acp-15-5683-2015, 2015.
Kourtchev, I., Godoi, R. H. M., Connors, S., Levine, J. G., Archibald, A. T., Godoi, A. F. L., Paralovo, S. L., Barbosa, C. G. G., Souza, R. A. F., Manzi, A. O., Seco, R., Sjostedt, S., Park, J.-H., Guenther, A., Kim, S., Smith, J., Martin, S. T., and Kalberer, M.: Molecular composition of organic aerosols in central Amazonia: an ultra-high-resolution mass spectrometry study, Atmos. Chem. Phys., 16, 11899–11913, https://doi.org/10.5194/acp-16-11899-2016, 2016.
Kristensen, K. and Glasius, M.: Organosulfates and oxidation products from biogenic hydrocarbons in fine aerosols from a forest in North West Europe during spring, Atmos. Environ., 45, 4546–4556, https://doi.org/10.1016/j.atmosenv.2011.05.063, 2011.
Kristensen, K., Enggrob, K. L., King, S. M., Worton, D. R., Platt, S. M., Mortensen, R., Rosenoern, T., Surratt, J. D., Bilde, M., Goldstein, A. H., and Glasius, M.: Formation and occurrence of dimer esters of pinene oxidation products in atmospheric aerosols, Atmos. Chem. Phys., 13, 3763–3776, https://doi.org/10.5194/acp-13-3763-2013, 2013.
Kristensen, K., Cui, T., Zhang, H., Gold, A., Glasius, M., and Surratt, J. D.: Dimers in α-pinene secondary organic aerosol: effect of hydroxyl radical, ozone, relative humidity and aerosol acidity, Atmos. Chem. Phys., 14, 4201–4218, https://doi.org/10.5194/acp-14-4201-2014, 2014.
Kroll, J. H., Donahue, N. M., Jimenez, J. L., Kessler, S. H., Canagaratna, M. R., Wilson, K. R., Altieri, K. E., Mazzoleni, L. R., Wozniak, A. S., Bluhm, H., Mysak, E. R., Smith, J. D., Kolb, C. E., and Worsnop, D. R.: Carbon oxidation state as a metric for describing the chemistry of atmospheric organic aerosol, Nature Chem., 3, 133–139, https://doi.org/10.1038/nchem.948, 2011.
Kundu, S., Quraishi, T. A., Yu, G., Suarez, C., Keutsch, F. N., and Stone, E. A.: Evidence and quantitation of aromatic organosulfates in ambient aerosols in Lahore, Pakistan, Atmos. Chem. Phys., 13, 4865–4875, https://doi.org/10.5194/acp-13-4865-2013, 2013.
Li, X., Han, J., Hopke, P. K., Hu, J., Shu, Q., Chang, Q., and Ying, Q.: Quantifying primary and secondary humic-like substances in urban aerosol based on emission source characterization and a source-oriented air quality model, Atmos. Chem. Phys., 19, 2327–2341, https://doi.org/10.5194/acp-19-2327-2019, 2019.
Lin, P., Engling, G., and Yu, J. Z.: Humic-like substances in fresh emissions of rice straw burning and in ambient aerosols in the Pearl River Delta Region, China, Atmos. Chem. Phys., 10, 6487–6500, https://doi.org/10.5194/acp-10-6487-2010, 2010.
Lin, P., Yu, J. Z., Engling, G., and Kalberer, M.: Organosulfates in humic-like substance fraction isolated from aerosols at seven locations in East Asia: a study by ultra-high-resolution mass spectrometry, Environ. Sci. Technol., 46, 13118–13127, https://doi.org/10.1021/es303570v, 2012.
Lin, P., Aiona, P. K., Li, Y., Shiraiwa, M., Laskin, J., Nizkorodov, S. A., and Laskin, A.: Molecular Characterization of Brown Carbon in Biomass Burning Aerosol Particles, Environ. Sci. Technol., 50, 11815–11824, https://doi.org/10.1021/acs.est.6b03024, 2016.
Liu, S., Shilling, J. E., Song, C., Hiranuma, N., Zaveri, R. A., and Russell, L. M.: Hydrolysis of Organonitrate Functional Groups in Aerosol Particles, Aerosol Sci. Technol., 46, 1359–1369, https://doi.org/10.1080/02786826.2012.716175, 2012.
Mead, R. N., Felix, J. D., Avery, G. B., Kieber, R. J., Willey, J. D., and Podgorski, D. C.: Characterization of CHOS compounds in rainwater from continental and coastal storms by ultrahigh resolution mass spectrometry, Atmos. Environ., 105, 162–168, https://doi.org/10.1016/j.atmosenv.2015.01.057, 2015.
Merder, J., Freund, J. A., Feudel, U., Hansen, C. T., Hawkes, J. A., Jacob, B., Klaproth, K., Niggemann, J., Noriega-Ortega, B. E., Osterholz, H., Rossel, P. E., Seidel, M., Singer, G., Stubbins, A., Waska, H., and Dittmar, T.: ICBM-OCEAN: Processing Ultrahigh-Resolution Mass Spectrometry Data of Complex Molecular Mixtures, Anal. Chem., 92, 6832–6838, https://doi.org/10.1021/acs.analchem.9b05659, 2020.
Müller, L., Reinnig, M.-C., Warnke, J., and Hoffmann, Th.: Unambiguous identification of esters as oligomers in secondary organic aerosol formed from cyclohexene and cyclohexene/α-pinene ozonolysis, Atmos. Chem. Phys., 8, 1423–1433, https://doi.org/10.5194/acp-8-1423-2008, 2008.
Ng, N. L., Kwan, A. J., Surratt, J. D., Chan, A. W. H., Chhabra, P. S., Sorooshian, A., Pye, H. O. T., Crounse, J. D., Wennberg, P. O., Flagan, R. C., and Seinfeld, J. H.: Secondary organic aerosol (SOA) formation from reaction of isoprene with nitrate radicals (NO3), Atmos. Chem. Phys., 8, 4117–4140, https://doi.org/10.5194/acp-8-4117-2008, 2008.
Nie, W., Yan, C., Huang, D. D., Wang, Z., Liu, Y. L., Qiao, X. H., Guo, Y. S., Tian, L. H., Zheng, P. G., Xu, Z. N., Li, Y. Y., Xu, Z., Qi, X. M., Sun, P., Wang, J. P., Zheng, F. X., Li, X. X., Yin, R. J., Dallenbach, K. R., Bianchi, F., Petaja, T., Zhang, Y. J., Wang, M. Y., Schervish, M., Wang, S. N., Qiao, L. P., Wang, Q., Zhou, M., Wang, H. L., Yu, C. A., Yao, D. W., Guo, H., Ye, P. L., Lee, S. C., Li, Y. J., Liu, Y. C., Chi, X. G., Kerminen, V. M., Ehn, M., Donahue, N. M., Wang, T., Huang, C., Kulmala, M., Worsnop, D., Jiang, J. K., and Ding, A. J.: Secondary organic aerosol formed by condensing anthropogenic vapours over China's megacities, Nat. Geosci., 15, 255–261, https://doi.org/10.1038/s41561-022-00922-5, 2022.
Park, S. S. and Yu, J.: Chemical and light absorption properties of humic-like substances from biomass burning emissions under controlled combustion experiments, Atmos. Environ., 136, 114–122, https://doi.org/10.1016/j.atmosenv.2016.04.022, 2016.
Passananti, M., Kong, L., Shang, J., Dupart, Y., Perrier, S., Chen, J., Donaldson, D. J., and George, C.: Organosulfate Formation through the Heterogeneous Reaction of Sulfur Dioxide with Unsaturated Fatty Acids and Long-Chain Alkenes, Angewandte Chemie International Edition, 55, 10336–10339, https://doi.org/10.1002/anie.201605266, 2016.
Pavuluri, C. M., Wang, S., Fu, P. Q., Zhao, W., Xu, Z., and Liu, C. Q.: Molecular distributions of diacids, oxoacids and α-dicarbonyls in summer- and winter-time fine aerosols from Tianjin, North China: Emissions from combustion sources and aqueous phase secondary formation, J. Geophys. Res.-Atmos., 126, e2020JD032961, https://doi.org/10.1029/2020jd032961, 2020.
Perraud, V., Bruns, E. A., Ezell, M. J., Johnson, S. N., Greaves, J., and Finlayson-Pitts, B. J.: Identification of organic nitrates in the NO3 radical initiated oxidation of alpha-pinene by atmospheric pressure chemical ionization mass spectrometry, Environ. Sci. Technol., 44, 5887–5893, https://doi.org/10.1021/es1005658, 2010.
Qi, Y., Hempelmann, R., and Volmer, D. A.: Shedding light on the structures of lignin compounds: photo-oxidation under artificial UV light and characterization by high resolution mass spectrometry, Anal. Bioanal. Chem., 408, 8203–8210, https://doi.org/10.1007/s00216-016-9928-7, 2016.
Qi, Y., Xie, Q., Wang, J.-J., He, D., Bao, H., Fu, Q.-L., Su, S., Sheng, M., Li, S.-L., Volmer, D. A., Wu, F., Jiang, G., Liu, C.-Q., and Fu, P.: Deciphering dissolved organic matter by Fourier transform ion cyclotron resonance mass spectrometry (FT-ICR MS): from bulk to fractions and individuals, Carbon Res., 1, 3, https://doi.org/10.1007/s44246-022-00002-8, 2022.
Riva, M., Da Silva Barbosa, T., Lin, Y.-H., Stone, E. A., Gold, A., and Surratt, J. D.: Chemical characterization of organosulfates in secondary organic aerosol derived from the photooxidation of alkanes, Atmos. Chem. Phys., 16, 11001–11018, https://doi.org/10.5194/acp-16-11001-2016, 2016.
Saleh, R., Robinson, E. S., Tkacik, D. S., Ahern, A. T., Liu, S., Aiken, A. C., Sullivan, R. C., Presto, A. A., Dubey, M. K., Yokelson, R. J., Donahue, N. M., and Robinson, A. L.: Brownness of organics in aerosols from biomass burning linked to their black carbon content, Nat. Geosci., 7, 647–650, https://doi.org/10.1038/ngeo2220, 2014.
Šantl-Temkiv, T., Finster, K., Dittmar, T., Hansen, B. M., Thyrhaug, R., Nielsen, N. W., and Karlson, U. G.: Hailstones: a window into the microbial and chemical inventory of a storm cloud, PloS one, 8, e53550, https://doi.org/10.1371/journal.pone.0053550, 2013.
Sharkey, T. D., Wiberley, A. E., and Donohue, A. R.: Isoprene emission from plants: why and how, Ann. Bot., 101, 5–18, https://doi.org/10.1093/aob/mcm240, 2008.
Shi, Z., Vu, T., Kotthaus, S., Harrison, R. M., Grimmond, S., Yue, S., Zhu, T., Lee, J., Han, Y., Demuzere, M., Dunmore, R. E., Ren, L., Liu, D., Wang, Y., Wild, O., Allan, J., Acton, W. J., Barlow, J., Barratt, B., Beddows, D., Bloss, W. J., Calzolai, G., Carruthers, D., Carslaw, D. C., Chan, Q., Chatzidiakou, L., Chen, Y., Crilley, L., Coe, H., Dai, T., Doherty, R., Duan, F., Fu, P., Ge, B., Ge, M., Guan, D., Hamilton, J. F., He, K., Heal, M., Heard, D., Hewitt, C. N., Hollaway, M., Hu, M., Ji, D., Jiang, X., Jones, R., Kalberer, M., Kelly, F. J., Kramer, L., Langford, B., Lin, C., Lewis, A. C., Li, J., Li, W., Liu, H., Liu, J., Loh, M., Lu, K., Lucarelli, F., Mann, G., McFiggans, G., Miller, M. R., Mills, G., Monk, P., Nemitz, E., O'Connor, F., Ouyang, B., Palmer, P. I., Percival, C., Popoola, O., Reeves, C., Rickard, A. R., Shao, L., Shi, G., Spracklen, D., Stevenson, D., Sun, Y., Sun, Z., Tao, S., Tong, S., Wang, Q., Wang, W., Wang, X., Wang, X., Wang, Z., Wei, L., Whalley, L., Wu, X., Wu, Z., Xie, P., Yang, F., Zhang, Q., Zhang, Y., Zhang, Y., and Zheng, M.: Introduction to the special issue “In-depth study of air pollution sources and processes within Beijing and its surrounding region (APHH-Beijing)”, Atmos. Chem. Phys., 19, 7519–7546, https://doi.org/10.5194/acp-19-7519-2019, 2019.
Simoneit, B. R. T.: Biomass burning – a review of organic tracers for smoke from incomplete combustion, Appl. Geochem., 17, 129–162, https://doi.org/10.1016/S0883-2927(01)00061-0, 2002.
Sleighter, R. L. and Hatcher, P. G.: Molecular characterization of dissolved organic matter (DOM) along a river to ocean transect of the lower Chesapeake Bay by ultrahigh resolution electrospray ionization Fourier transform ion cyclotron resonance mass spectrometry, Marine Chem., 110, 140–152, https://doi.org/10.1016/j.marchem.2008.04.008, 2008.
Song, J., Li, M., Jiang, B., Wei, S., Fan, X., and Peng, P.: Molecular Characterization of Water-Soluble Humic like Substances in Smoke Particles Emitted from Combustion of Biomass Materials and Coal Using Ultrahigh-Resolution Electrospray Ionization Fourier Transform Ion Cyclotron Resonance Mass Spectrometry, Environ. Sci. Technol., 52, 2575–2585, https://doi.org/10.1021/acs.est.7b06126, 2018.
Su, S. H., Xie, Q. R., Smith, A. J., Lang, Y. C., Hu, W., Cao, D., Yue, S. Y., Chen, S., Zhu, J. L., Xu, Y. S., Bell, N. G. A., Uhrin, D., and Fu, P. Q.: A New Structural Classification Scheme for Dissolved Organic Sulfur in Urban Snow from North China, Environ. Sci. Technol. Lett., 9, 366–374, https://doi.org/10.1021/acs.estlett.2c00153, 2022.
Sun, Y. L., Wang, Z. F., Fu, P. Q., Yang, T., Jiang, Q., Dong, H. B., Li, J., and Jia, J. J.: Aerosol composition, sources and processes during wintertime in Beijing, China, Atmos. Chem. Phys., 13, 4577–4592, https://doi.org/10.5194/acp-13-4577-2013, 2013.
Surratt, J. D., Gómez-González, Y., Chan, A. W. H., Vermeylen, R., Shahgholi, M., Kleindienst, T. E., Edney, E. O., Offenberg, J. H., Lewandowski, M., Jaoui, M., Maenhaut, W., Claeys, M., Flagan, R. C., and Seinfeld, J. H.: Organosulfate Formation in Biogenic Secondary Organic Aerosol, J. Phys. Chem. A, 112, 8345–8378, https://doi.org/10.1021/jp802310p, 2008.
Tao, S., Lu, X., Levac, N., Bateman, A. P., Nguyen, T. B., Bones, D. L., Nizkorodov, S. A., Laskin, J., Laskin, A., and Yang, X.: Molecular characterization of organosulfates in organic aerosols from Shanghai and Los Angeles urban areas by nanospray-desorption electrospray ionization high-resolution mass spectrometry, Environ. Sci. Technol., 48, 10993–11001, https://doi.org/10.1021/es5024674, 2014.
Tolocka, M. P. and Turpin, B.: Contribution of Organosulfur Compounds to Organic Aerosol Mass, Environ. Sci. Technol., 46, 7978–7983, https://doi.org/10.1021/es300651v, 2012.
Tu, P., Hall, W. A., and Johnston, M. V.: Characterization of Highly Oxidized Molecules in Fresh and Aged Biogenic Secondary Organic Aerosol, Anal. Chem., 88, 4495–4501, https://doi.org/10.1021/acs.analchem.6b00378, 2016.
Wang, X. K., Rossignol, S., Ma, Y., Yao, L., Wang, M. Y., Chen, J. M., George, C., and Wang, L.: Molecular characterization of atmospheric particulate organosulfates in three megacities at the middle and lower reaches of the Yangtze River, Atmos. Chem. Phys., 16, 2285–2298, https://doi.org/10.5194/acp-16-2285-2016, 2016.
Wang, Y., Hu, M., Lin, P., Tan, T., Li, M., Xu, N., Zheng, J., Du, Z., Qin, Y., Wu, Y., Lu, S., Song, Y., Wu, Z., Guo, S., Zeng, L., Huang, X., and He, L.: Enhancement in Particulate Organic Nitrogen and Light Absorption of Humic-Like Substances over Tibetan Plateau Due to Long-Range Transported Biomass Burning Emissions, Environ. Sci. Technol., 53, 14222–14232, https://doi.org/10.1021/acs.est.9b06152, 2019.
Wang, Y., Tong, R., and Yu, J. Z.: Chemical Synthesis of Multifunctional Air Pollutants: Terpene-Derived Nitrooxy Organosulfates, Environ. Sci. Technol., 55, 8573–8582, https://doi.org/10.1021/acs.est.1c00348, 2021.
Washenfelder, R. A., Attwood, A. R., Brock, C. A., Guo, H., Xu, L., Weber, R. J., Ng, N. L., Allen, H. M., Ayres, B. R., Baumann, K., Cohen, R. C., Draper, D. C., Duffey, K. C., Edgerton, E., Fry, J. L., Hu, W. W., Jimenez, J. L., Palm, B. B., Romer, P., Stone, E. A., Wooldridge, P. J., and Brown, S. S.: Biomass burning dominates brown carbon absorption in the rural southeastern United States, Geophys. Res. Lett., 42, 653–664, https://doi.org/10.1002/2014gl062444, 2015.
Wu, C., Yang, J., Fu, Q., Zhu, B., Ruan, T., and Jiang, G.: Molecular characterization of water-soluble organic compounds in PM2.5 using ultrahigh resolution mass spectrometry, Sci. Total Environ., 668, 917–924, https://doi.org/10.1016/j.scitotenv.2019.03.031, 2019.
Xie, Q., Li, Y., Yue, S., Su, S., Cao, D., Xu, Y., Chen, J., Tong, H., Su, H., Cheng, Y., Zhao, W., Hu, W., Wang, Z., Yang, T., Pan, X., Sun, Y., Wang, Z., Liu, C.-Q., Kawamura, K., and Fu, P.: Increase of High Molecular Weight Organosulfate with Intensifying Urban Air Pollution in the Megacity Beijing, J. Geophys. Res.-Atmos., 125, e2019JD032200, https://doi.org/10.1029/2019JD032200, 2020a.
Xie, Q., Su, S., Chen, S., Xu, Y., Cao, D., Chen, J., Ren, L., Yue, S., Zhao, W., Sun, Y., Wang, Z., Tong, H., Su, H., Cheng, Y., Kawamura, K., Jiang, G., Liu, C.-Q., and Fu, P.: Molecular characterization of firework-related urban aerosols using Fourier transform ion cyclotron resonance mass spectrometry, Atmos. Chem. Phys., 20, 6803–6820, https://doi.org/10.5194/acp-20-6803-2020, 2020b.
Xie, Q., Su, S., Dai, Y., Hu, W., Yue, S., Cao, D., Jiang, G., and Fu, P.: Deciphering 13C and 34S Isotopes of Organosulfates in Urban Aerosols by FT-ICR Mass Spectrometry, Environ. Sci. Technol. Lett., 9, 526–532, https://doi.org/10.1021/acs.estlett.2c00255, 2022.
Yan, C., Zheng, M., Desyaterik, Y., Sullivan, A. P., Wu, Y., and Collett Jr., J. L.: Molecular Characterization of Water-Soluble Brown Carbon Chromophores in Beijing, China, J. Geophys. Res.-Atmos., 125, e2019JD032018, https://doi.org/10.1029/2019jd032018, 2020.
Yasmeen, F., Vermeylen, R., Szmigielski, R., Iinuma, Y., Böge, O., Herrmann, H., Maenhaut, W., and Claeys, M.: Terpenylic acid and related compounds: precursors for dimers in secondary organic aerosol from the ozonolysis of α- and β-pinene, Atmos. Chem. Phys., 10, 9383–9392, https://doi.org/10.5194/acp-10-9383-2010, 2010.
Yasmeen, F., Szmigielski, R., Vermeylen, R., Gómez-González, Y., Surratt, J. D., Chan, A. W. H., Seinfeld, J. H., Maenhaut, W., and Claeys, M.: Mass spectrometric characterization of isomeric terpenoic acids from the oxidation of α-pinene, β-pinene, d-limonene, and Δ3-carene in fine forest aerosol, J. Mass Spectrom., 46, 425–442, https://doi.org/10.1002/jms.1911, 2011.
Yue, S. Y., Zhu, J. L., Chen, S., Xie, Q. R., Li, W., Li, L. J., Ren, H., Su, S. H., Li, P., Ma, H., Fan, Y. B., Cheng, B. R., Wu, L. B., Deng, J. J., Hu, W., Ren, L. J., Wei, L. F., Zhao, W. Y., Tian, Y., Pan, X. L., Sun, Y. L., Wang, Z. F., Wu, F. C., Liu, C.-Q., Su, H., Penner, J. E., Pöschl, U., Andreae, M. O., Cheng, Y. F., and Fu, P. Q.: Brown carbon from biomass burning imposes strong circum-Arctic warming, One Earth, 5, 293–304, https://doi.org/10.1016/j.oneear.2022.02.006, 2022.
Zhang, H., Worton, D. R., Lewandowski, M., Ortega, J., Rubitschun, C. L., Park, J.-H., Kristensen, K., Campuzano-Jost, P., Day, D. A., Jimenez, J. L., Jaoui, M., Offenberg, J. H., Kleindienst, T. E., Gilman, J., Kuster, W. C., de Gouw, J., Park, C., Schade, G. W., Frossard, A. A., Russell, L., Kaser, L., Jud, W., Hansel, A., Cappellin, L., Karl, T., Glasius, M., Guenther, A., Goldstein, A. H., Seinfeld, J. H., Gold, A., Kamens, R. M., and Surratt, J. D.: Organosulfates as Tracers for Secondary Organic Aerosol (SOA) Formation from 2-Methyl-3-Buten-2-ol (MBO) in the Atmosphere, Environ. Sci. Technol., 46, 9437–9446, https://doi.org/10.1021/es301648z, 2012.
Zhang, X., Lin, Y.-H., Surratt, J. D., and Weber, R. J.: Sources, Composition and Absorption Ångström Exponent of Light-absorbing Organic Components in Aerosol Extracts from the Los Angeles Basin, Environ. Sci. Technol., 47, 3685–3693, https://doi.org/10.1021/es305047b, 2013.
Zhao, Y., Hallar, A. G., and Mazzoleni, L. R.: Atmospheric organic matter in clouds: exact masses and molecular formula identification using ultrahigh-resolution FT-ICR mass spectrometry, Atmos. Chem. Phys., 13, 12343–12362, https://doi.org/10.5194/acp-13-12343-2013, 2013.
Zhu, M., Jiang, B., Li, S., Yu, Q., Yu, X., Zhang, Y., Bi, X., Yu, J., George, C., Yu, Z., and Wang, X.: Organosulfur Compounds Formed from Heterogeneous Reaction between SO2 and Particulate-Bound Unsaturated Fatty Acids in Ambient Air, Environ. Sci. Technol. Lett., 6, 318–322, https://doi.org/10.1021/acs.estlett.9b00218, 2019.
Zhu, Y., Yang, L., Chen, J., Wang, X., Xue, L., Sui, X., Wen, L., Xu, C., Yao, L., Zhang, J., Shao, M., Lu, S., and Wang, W.: Characteristics of ambient volatile organic compounds and the influence of biomass burning at a rural site in Northern China during summer 2013, Atmos. Environ., 124, 156–165, https://doi.org/10.1016/j.atmosenv.2015.08.097, 2016.