the Creative Commons Attribution 4.0 License.
the Creative Commons Attribution 4.0 License.
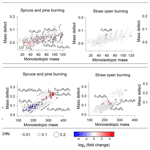
Bulk and molecular-level composition of primary organic aerosol from wood, straw, cow dung, and plastic burning
Jun Zhang
Tiantian Wang
Erlend Gammelsæter
Rico K. Y. Cheung
Mihnea Surdu
Sophie Bogler
Deepika Bhattu
Dongyu S. Wang
Tianqu Cui
Lu Qi
Houssni Lamkaddam
Imad El Haddad
Jay G. Slowik
Andre S. H. Prevot
During the past decades, the source apportionment of organic aerosol (OA) in ambient air has been improving substantially. The database of source retrieval model-resolved mass spectral profiles for different sources has been built with the aerosol mass spectrometer (AMS). However, distinguishing similar sources (such as wildfires and residential wood burning) remains challenging, as the hard ionization of the AMS mostly fragments compounds and therefore cannot capture detailed molecular information. Recent mass spectrometer technologies of soft ionization and high mass resolution have allowed for aerosol characterization at the molecular formula level. In this study, we systematically estimated the emission factors and characterized the primary OA (POA) chemical composition with the AMS and the extractive electrospray ionization time-of-flight mass spectrometer (EESI-TOF) for the first time from a variety of solid fuels, including beech logs, spruce and pine logs, spruce and pine branches and needles, straw, cow dung, and plastic bags. The emission factors of organic matter estimated by the AMS and hydrocarbon gases estimated by the total hydrocarbon analyzer are 16.2 ± 10.8 g kg−1 and 30.3 ± 8.5 g kg−1 for cow dung burning, which is generally higher than that of wood (beech, spruce, and pine), straw, and plastic bag burning (in the range from 1.1 to 6.2 g kg−1 and 14.1 to 19.3 g kg−1). The POA measured by the AMS shows that the f60 (mass fraction of 60) varies from 0.003 to 0.04 based on fuel types and combustion efficiency for wood (beech, spruce, and pine) and cow dung burning. On a molecular level, the dominant compound of POA from wood, straw, and cow dung is C6H10O5 (mainly levoglucosan), contributing ∼ 7 % to ∼ 30 % of the total intensity, followed by C8H12O6 with fractions of ∼ 2 % to ∼ 9 %. However, as they are prevalent in all burning of biomass material, they cannot act as tracers for the specific sources. By using the Mann–Whitney U test among the studied fuels, we find specific potential new markers for these fuels from the measurement of the AMS and EESI-TOF. Markers from spruce and pine burning are likely related to resin acids (e.g., compounds with 20–21 carbon atoms). The product from the pyrolysis of hardwood lignins is found especially in beech log burning. Nitrogen-containing species are selected markers primarily for cow dung open burning. These markers in the future will provide support for the source apportionment.
- Article
(5938 KB) - Full-text XML
-
Supplement
(1017 KB) - BibTeX
- EndNote
Emissions from combustion are a major source of primary organic aerosol (POA), black carbon (BC), inorganic aerosol, and inorganic/organic gases (Andreae, 2019; Bond et al., 2007). After being emitted to the atmosphere, volatile organic compounds (VOCs) can further react to form lower-volatility components and generate secondary organic aerosol (SOA). The primary emissions and their subsequent transformations have significant implications for air quality, climate, and human health (Chen et al., 2017). Accordingly, a large number of laboratory and field measurements have been carried out to disentangle the roles of burning-induced aerosols in polluted areas.
Solid fuel combustion is a major source of air pollution in many places over the world. In Southeast Asia, haze events are mainly attributed to the wildfires, agricultural waste burning, and peatland fires (Adam et al., 2021). In India, more than half of households use inefficient stoves for cooking, burning solid fuels such as firewood, charcoal, crop residues, and cow dung (Census of India, 2011). This contributes to poor household air quality, chronic and acute respiratory diseases, and even premature death (Smith et al., 2014). Plastic burning has been estimated to contribute 13.4 % of fine particulate matter (PM2.5) yearly in India, 6.8 % in wintertime in China (Haque et al., 2019), and 2 % to 7 % in wintertime in the US (Islam et al., 2022). The toxic pollutants released from plastic burning, including olefins, paraffin, and polycyclic aromatic hydrocarbons, can cause respiratory irritation and have carcinogenic and mutagenic effects (Pathak et al., 2023). The extent to which primary particulate matter adversely affects health is source-dependent. Recent studies have shown that biomass burning-related particles have been linked to reactive oxygen species and oxidative stress, increasing the risks of cardiovascular diseases (Daellenbach et al., 2020; de Oliveira Alves et al., 2017; Tuet et al., 2019). Therefore, identifying the sources of aerosols is essential for assessing health risks and developing mitigation strategies.
Organic aerosol (OA) source apportionment has been widely studied using receptor models, e.g., positive matrix factorization (PMF), with OA composition characterized by an aerosol mass spectrometer (AMS) or aerosol chemical speciation monitor (ACSM). Many studies have successfully resolved source-related factors, for example, hydrocarbon-like OA (HOA), oxygenated OA (OOA), biomass burning OA (BBOA), coal combustion OA (CCOA), and so on, via PMF (Chen et al., 2021; Huang et al., 2014; Ng et al., 2010; Tobler et al., 2020; Wang et al., 2019; Crippa et al., 2014). The identification and validation of resolved factors rely strongly upon the spectral characteristics of source emissions. For example, hydrocarbon ion series CnH and CnH, e.g., C4H at 57 and C3H at 41, are often referenced as tracers for HOA (Mohr et al., 2012), while C2H4O at 60 is the main marker for wood and other biomass burning, as C2H4O is a characteristic major fragment of anhydrosugars (e.g., levoglucosan) produced from cellulose pyrolysis (Alfarra et al., 2007). However, achieving finer separation and interpretation of sources within one of the OA categories mentioned above from highly mixed aerosols in the environment remains challenging because the laboratory mass spectral profile database of primary emissions is limited and the potential molecular specificity is impeded by intensive fragmentation in the AMS and ACSM.
To minimize the loss of the molecular information from fragmentation, soft ionization and novel sampling techniques have been deployed to measure the chemical composition of particles in greater detail. A thermal desorption aerosol gas chromatograph (TAG) coupled to an AMS has been used and provided the molecular characterization of OA and SOA (Bertrand et al., 2018). The filter inlet for gases and aerosols (FIGAERO) measures molecular composition of OA via thermal desorption coupled to a chemical-ionization mass spectrometer (Lopez-Hilfiker et al., 2014). Nonetheless, thermal decomposition can occur during the thermal desorption process (Stark et al., 2017), causing potential artifacts and hindering the identification of components. A liquid chromatography mass spectrometer can avoid thermal desorption and separate mixtures including isomers based their chemical affinity with the mobile and stationary phases (Zhang et al., 2021). However, it requires pre-treatment of samples, which could introduce artifacts and lowers the time resolution. An extractive electrospray ionization time-of-flight mass spectrometer (EESI-TOF) has been recently developed for online OA measurement with generally insignificant decomposition or fragmentation (Lopez-Hilfiker et al., 2019). As a result, it provides a molecular-level (i.e., molecular formula determination) mass spectrum with a time resolution of seconds. Consequently, improved real-time investigations of chemical composition in chamber experiments (Surdu et al., 2023; Bell et al., 2022) and SOA source apportionment in the field measurement (Tong et al., 2021; Kumar et al., 2022; Qi et al., 2022) became possible. Thus far, a detailed study of primary emissions from complex sources, e.g., combustion, has not yet been conducted with the EESI-TOF, which necessitates the measurement to fully utilize the chemical resolution capabilities of EESI-TOF for characterizing mass spectra and supporting the source apportionment in the field.
In this work, we systematically characterize the POA composition using both the AMS and EESI-TOF from a variety of burning fuels from both residential stoves (beech logs and a mixture of spruce and pine logs) and open combustion (spruce and pine branches and needles, straw, cow dung, and polyethylene plastic bags). The emission factors of trace gases are presented and possible molecular markers for the burning fuels in this study are discussed. This work allows for a better understanding of the POA chemical composition emitted from different burning sources, provides important reference spectra for source apportionment, and potential markers to use to assess the importance of different biomass burning sources.
2.1 Experimental setup and instrumentation
A total of 36 burning experiments were conducted using six different types of burning materials, including beech, spruce, pine, straw, cow dung, and plastic bags. Beech logs, spruce and pine logs, fresh spruce and pine branches and needles, and straw were sourced from a local forestry company in Würenlingen, Switzerland. Cow dung cakes (made of cow dung and straw) were collected from Goyla dairy, Delhi, India, and polyethylene plastic bags were bought in Delhi, India. To represent residential burning, logs of (1) beech and (2) spruce and pine were burned separately in a stove (Bruns et al., 2017). Agricultural waste combustion and forest fires were, respectively, represented by burning (1) straw and (2) a mixture of fresh branches and needles of spruce and pine in an open stainless steel cylinder (65 cm in diameter and 35 cm in height). Finally, the half-open stove (e.g., angithi) and waste burning in India and some other areas (Fleming et al., 2018), respectively, were represented by burning (1) cow dung cakes and (2) plastic bags on top of the stainless steel cylinder, with the fuel resting on a mesh steel plate. The experimental setup is shown in Fig. S1 in the Supplement. The fuels were ignited with fire starters and/or kindling and the emissions were pulled into either a chimney (for stove burning) or a hood (for open burning). After starters/kindling burned away (∼ 3 to 10 min after ignition), the emissions were introduced into a holding tank through stainless steel sampling lines heated to 180 ∘C and passing through an ejection dilutor (DI-1000, Dekati Ltd.) with a dilution ratio of ∼ 10. The holding tank is a stainless steel container (1 m3) used to store emissions. It is also designed for averaging the emissions at different combustion efficiency in order to fully characterize the emission in the real ambient. The emissions were injected into the holding tank for 10 to 30 min, depending on the emission source. Typically, the injection was stopped when the measured POA concentration was above ∼ 20 µg m−3 after ∼ 60-fold dilution in the sampling lines. In different burning experiments, POA concentrations in the holding tank varied between 1 to 5 mg m−3 prior to sampling line dilution. The holding tank was flushed overnight with clean air before each experiment, ensuring the background particle concentrations were less than 10 particles cm−3.
The emissions were delivered from the holding tank to sampling instruments via stainless steel lines (6 mm o.d.) for particles and via Teflon lines (6 mm o.d.) for gases. Gas analyzers were used for monitoring the concentration of CO (Horiba APMA-370), CO2 (LI-COR LI-7000), and total hydrocarbon (THC, including methane) (Horiba APHA-370). Particle concentrations were measured using a scanning mobility particle sizer (SMPS, model 3938, TSI) scanning a range of 16 to 638 nm. An Aethalometer (AE 33, Magee Scientific) was used to retrieve the concentration of equivalent BC (eBC). A long time-of-flight aerosol mass spectrometer (LTOF-AMS, Aerodyne Research, Inc.) with a mass resolution of ∼ 5000 over the range of 100 to 450 was deployed for online, non-refractory particle characterization and a subset of experiments were performed with a high-resolution time-of-flight AMS (HTOF-AMS, Aerodyne Research, Inc.) with a mass resolution of ∼ 2000 over the range of 100 to 450. The aerosols sampled by both the SMPS and AMS were dried with a Nafion dryer (Perma Pure). The aerosol was continuously sampled by the AMS through a 100 µm critical orifice and focused by PM1 aerodynamic lens. Therefore, the class of the PM in this study belongs to PM1. Mass spectra of positive ion fragments ( 10 to 450) were obtained with a TOF mass spectrometer and were analyzed with the software SQUIRREL (SeQUential Igor data RetRiEvaL) v.1.63 and PIKA (Peak Integration by Key Analysis) v.1.23 for the IGOR Pro software package (Wavemetrics, Inc.). A detailed description of the AMS can be found in Decarlo et al. (2006).
EESI-TOF was deployed for a real-time and molecular-level (i.e., molecular formula) measurement of OA with minimal analyte fragmentation or decomposition (Lopez-Hilfiker et al., 2019). Before entering the EESI-TOF, the aerosol passes through an activated charcoal denuder to remove gas-phase species. The aerosol intersects a spray of charged droplets generated by an electrospray probe. Particles coagulate with the electrospray (ES) droplets, and water-soluble compounds are extracted into the solvent and then ionized via the Coulomb explosion mechanism as the droplets evaporate. The electrospray solution was 100 ppm sodium iodide (NaI) in pure water (MilliQ), resulting in the formation of [M + Na]+ (M is the analyte) adduct in the positive ionization mode. The EESI-TOF mass analyzer achieved a mass resolution of ∼ 10000 at 173 and 11 000 at 323. The EESI-TOF operated with a time resolution of 1 s and alternated 1.5 min of background measurements (in which the sampled air passes through a high-efficiency particulate air (HEPA) filter to remove particles) with 3.5 min of direct sampling. These data were pre-averaged to 5 s for further analysis. Ions are only regarded as signals from emissions when their intensity difference between the particle measurement and the corresponding background measurement periods were 1.9 times bigger than the propagated standard errors over the measurement cycle. For those selected ions, their mass flux to the detector was calculated as Eq. (1):
where Massx and Ix are, respectively, the mass flux (attograms per second, ag s−1) and the ion flux (counts per second, cps) of a group of detected ions with the same molecular weight. MWx is the molecular weight of x (with the mass of the charge carrier, typically Na+, removed). Na is Avogadro's number. To assist with the peak identification, filters were collected from emissions and were analyzed with an ultrahigh-resolution mass spectrometer (Orbitrap). The Orbitrap (Orbitrap Exploris 120, Thermo Fisher) has a mass-resolving power of 140 000 at 200 and was operated in positive mode scanning from 50 to 450.
2.2 Data analysis
The emission factor (EF) of species i was calculated using the carbon mass balance method (Radke and Ward, 1993), expressed in the unit of grams per kilogram, as shown in Eq. (2).
where ΔCO, ΔCO2, ΔTHC, ΔOC, and ΔBC are the background-corrected carbon mass concentrations of CO, CO2, THC, OC (organic carbon), and BC. OC was calculated from the ratio of organic aerosol and the ratio of organic mass (OM) to OC (OM/OC) measured by the AMS (Canagaratna et al., 2015). mi is the mass concentration of species i. WC is the carbon mass fraction of the burning fuel. The WC was reported to be 0.46 for wood (Bertrand et al., 2017), 0.45 for straw (Li et al., 2007), 0.45 for cow dung (Font-Palma, 2019), and 0.84 for plastic bags (Li et al., 2001). In experiments, where BC is not available, the sum of OC and BC is considered equal to the particulate matter (PM) determined by SMPS. The effective density of particles applied in the SMPS is determined by comparing mass and volume distributions from the AMS and SMPS (Bahreini et al., 2005). The densities could be underestimated because of the non-spherical shape of particles, especially particles from plastic bag burning mainly due to the high contribution of BC. As the contribution of particles to the total carbon is much smaller than the gases, these two methods have little differences calculating the denominator in Eq. (2). Therefore, the EFs of CO, CO2, and THC are consistent using both methods. However, it could be important for calculating the EFs for particulate species because of the possible discrepancy between the mass measured by the SMPS and AMS arising from, for example, the particle size and effective density. Additionally, the acquired by the AMS would also add uncertainty when converting OM to OC because the high range of without peak fitting is not included in . More comparison is discussed in Sect. 3.1.
The combustion condition was characterized by the modified combustion efficiency (MCE, Eq. 3) (Ward and Hardy, 1991). When the MCE is higher than 0.9, the combustion is regarded as predominantly flaming. When the MCE is lower than 0.85, it is dominated by smoldering.
2.3 Identification of potential markers
The identification of potential markers for emissions was performed by the Mann–Whitney U test (Mann and Whitney, 1947; Wilcoxon, 1945), which has been applied in many fields and for the current study has the advantage that it does not require a large volume of normally distributed samples (Rugiel et al., 2021; Tai et al., 2022). It tests the null hypothesis that the two population medians are equal against the alternative hypothesis that the two populations are not equal. When the p value is smaller than the significance level of 0.1, the median of the tested sample is significantly high or low in the two-tailed test. Ions from a class of fuel that satisfy the pairwise comparison test between one fuel j and other types of fuels were considered to be significantly high-fraction or low-fraction ions in the fuel j and therefore have the potential as markers for the fuel j. The fold change (FC) of ion i in the fuel j was calculated as the Eq. (4), where the fi,j is the fraction of ion i in the mass spectra profiles of the fuel j and fi,other is the average fraction of ion i in the mass spectra from the other fuels.
3.1 Emission factors from combustion
The average EFs of CO, CO2, THC, PM, OM, and eBC, as well as the MCE values of the six types of burning are shown in Table 1, and the EFs and MCE values for each experiment are presented in Table S1.
Table 1Average emission factors of CO, CO2, THC, PM, OM, and BC as well as MCE for six types of burning.
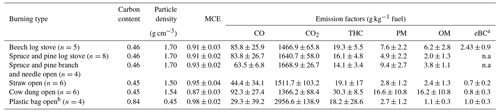
a The number of burns is indicated by n. b The emission factors of PM and OM are corrected as explained in the Supplement. c BC data are not available for some burns, denoted by “n.a.” (not available).
The average MCE values depend on fuel types, with the lowest values of 0.87 ± 0.03 (average ± 1σ) observed from cow dung open burning and the highest values of 0.98 ± 0.02 from plastic bag open burning, consistent with smoldering combustion for cow dung and flaming/melting processes for plastic bags. Accordingly, cow dung had the highest average CO EF (92.3 ± 27.4 g kg−1) and the lowest CO2 EF (1366.2 ± 88.4 g kg−1) and vice versa for plastic bags. The strong relationship between the MCE and some EFs is also true for the THC. In general, lower the MCEs correspond to higher THC EFs within a given class of burning fuel. Taking straw burning as an example, as shown in Table S1, the EFs of THC vary from 0.7 to 39.3 g kg−1, with the MCE varying from nearly 1.00 to 0.89 correspondingly, resulting in the high standard deviation of the EFs. These EFs of gases are comparable with the reported EFs from the literature under similar conditions (Hennigan et al., 2011; Fang et al., 2017; Bertrand et al., 2017).
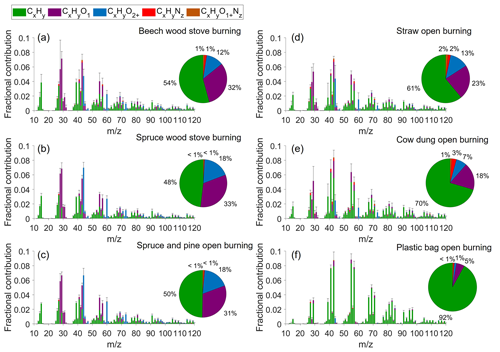
Figure 1Average AMS POA mass spectral profiles and elemental compositions of (a) beech log stove burning (n=6; n is the number of experiments), (b) spruce and pine log stove burning (n=9), (c) spruce and pine branch and needle open burning (n=4), (d) straw open burning (n=6), (e) cow dung open burning (n=5), and (f) plastic bag burning (n=4). The error bar denotes half the standard deviation in gray. The pie chart showing the contribution of elemental families is to the right of the mass spectrum.
The average EFs of PM are in the range of 3.1 to 16.6 g kg−1. In general, the PM emitted from cow dung is dominated by OM, and the eBC is minor. For beech logs and straw, the OM EF is around 3 times higher than the eBC EF. Noticeably, the emission of PM from plastic bags is not very high (2.7 g kg−1), but the EF of OM and eBC is similar (1.1 g kg−1 vs. 1.0 g kg−1). Note that when eBC data are not available, the sum of OC and BC in the denominator in Eq. (2) is assumed to be equal to the PM measured by the SMPS. Table S1 lists the comparison of EFs for particulate species where possible. For the experiments of cow dung open burning and plastic bag open burning, the EFs are consistent using both methods with the difference of PM EF < 6 %, and on average less than 15 % for OM EF. However, for some beech log stove burning experiments (BS3 and BS4), the effective density required in the calculation is not available, and the average density of other beech logs in this study is used. This results in some variance between these two methods. In general, the EFs of PM, OM, and BC agree well with some previous literature (Fang et al., 2017; Goetz et al., 2018; Tissari et al., 2008). Nevertheless, the reported EF values are highly dependent on the burning method (e.g., stove type) and combustion efficiency (Bertrand et al., 2017). Additionally, the reported EFs for plastics vary substantially with their composition, and the EFs of the pure polyethylene (PE) plastic bags are not often reported (Jayarathne et al., 2018; Wu et al., 2021; Hoffer et al., 2020).
3.2 Chemical composition of POAs from combustion
3.2.1 Chemical composition of POAs measured with the AMS
The chemical composition of POAs of burning emissions is characterized with the AMS and EESI-TOF simultaneously. As shown in Fig. 1, the average mass spectra from 10 to 120 measured with the AMS are grouped into the CxHy, CxHyO1, CxHyO2+, CxHyNz, and CxHyO1+Nz families based on their elemental composition. In all the POAs, the CxHy family is the most abundant group, mainly from ions at 29, 39, 41, 43, 55, 57, 67, and 69 originating primarily from hydrocarbon compounds, with the biggest contribution from plastic bags (92 %), followed by cow dung (70 %) and straw (61 %), and generally higher than that of wood (beech, spruce, and pine, 48 % to 54 %) burning. The CxHyO2+ and CxHyO1 families are the second largest compositions, with major ions at 28, 29, 43, 44, and 60, which are higher in wood and straw emissions compared to cow dung. Among these ions, the mass fractions of 44 (f44, mostly CO, the mass fraction of 43 (f43, mostly C2H3O+ and C3H, and mass fraction of 60 (f60, mostly C2H4O have the largest impact on the oxidation state of the aerosol. The fragment C2H4O at 60 is widely used as a levoglucosan-related marker for biomass burning and is most prominent in the wood burning emissions compared to the other burning fuels. Figure 2a shows that the POAs in this study are at the bottom left of the ambient OOA range (Ng et al., 2010) in the f44 vs. f43 plot, indicating the POA is less oxygenated, which is consistent with previous studies (Hennigan et al., 2011; Fang et al., 2017; Xu et al., 2020). As shown in Fig. 2b, the f60 for the biomass source studied is greater than the background level (Cubison et al., 2011), suggesting the f60 filter (f60=0.003) in the ambient is unlikely to miss biomass combustion. The contribution of f60 relates to the burning fuel types and the combustion efficiency. For example, the f60 from wood burning ranges from 0.02 to 0.04, generally higher than that of cow dung. The f60 of straw open burning is distributed from below 0.01 to 0.025, resulting from the low to high MCE values correspondingly. The pie charts in Fig. 1 indicate that the N-containing fragments from the AMS are mainly from the CxHyNz and CxHyO1+Nz family, and they are the largest in the emission of cow dung open burning (4.3 %), followed by straw (3.4 %), while the wood is relatively minimal (≤1 %) (Stockwell et al., 2016). The nitrogen in organonitrates would appear mainly at fragments of NO+ and NO. However, the NO+ and NO originating from organonitrates are estimated to be almost 20 times smaller than the CxHyNz family in cow dung burning using the NO+ and NO ratio between inorganic nitrates and organonitrates (Farmer et al., 2010), suggesting their contributions to organic nitrogen are minor.
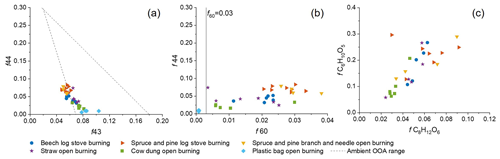
Figure 2Scatterplots of (a) f44 vs. f43 from the AMS, (b) f44 vs. f60 from the AMS, and (c) f C6H10O5 vs. fC8H12O6 from EESI-TOF. The dashed line denotes the estimated OOA range and the solid line denotes f60 background level in the ambient from Ng et al. (2010) and Cubison et al. (2011).
In the region from 120 to 450 as shown in Fig. S3, polycyclic aromatic hydrocarbons (PAHs) are observed. Based on the spectra of laboratory standards (Dzepina et al., 2007; Aiken et al., 2007), parent ions at 226, 252, 276, 300, and 326 correspond, respectively, to C18H10 (benzo[ghi]fluoranthene and cyclopenta[cd]pyrene) C20H12 (benzofluoranthene and benzopyrene), C22H12 (indenopyrene and benzoperylene), C24H12 (coronene), and C26H14 (dibenzoperylene). The fragment of 239 could be methylbenzo[ghi]fluoranthene (C19H12) (Dzepina et al., 2007; Ji et al., 2010) or a fragment of dehydroabietic acid, which has been found in fresh pine resin (Colombini et al., 2005). The 219 and 285 also could arise from the fragmentation of retene and dehydroabietic acid, respectively, which also can be derived from conifer resin (Dzepina et al., 2007; Jen et al., 2019; Zetra et al., 2016). These ions contribute 0.69 % ± 0.14 % and 0.66 % ± 0.11 %, respectively, to the total POA of the spruce and pine branch and needle open burning, on the one hand, and spruce and pine log stove burning, on the other hand, which is distinct from straw (0.36 % ± 0.13 %), beech logs (0.34 % ± 0.14 %), and cow dung (0.25 % ± 0.13 %). Not many PAHs are observed with the AMS for the plastic bags. The difference for the observed PAH contribution is mainly caused by the burning material, i.e., the precursor of PAHs, such as lignin, single-ring compounds, and aliphatic hydrocarbons. The burning of PE has lower yield of PAHs than lignin (Zhou et al., 2015), resulting in the lower PAH contribution in the burning of polyethylene plastic bags.
3.2.2 Chemical composition of POAs measured with the EESI-TOF
The EESI-TOF provides an important complement to the highly fragmented mass spectra generated by the AMS, where intact compounds measured by the EESI-TOF from 100 to 400 without assuming specific response factors toward each molecular formula are shown in Fig. 3. The mass spectrum of plastic bags is not shown because the EESI-TOF is insensitive to hydrocarbons because of their low solubility in the electrospray and low affinity for Na+. The bin of compounds containing greater than 0.7 has the largest and similar contribution in wood burning (29.9 % to 31.5 %), and it is slightly smaller in straw (25 %) and cow dung (20.1 %). smaller than 0.15 contributes 15.3 % to 18.8 % in spruce and pine, which is similar to the fraction in cow dung (13.5 %) but much higher compared to beech logs (4.7 %) and straw (8.8 %), mainly due to the greater contribution of compounds with carbon numbers in the range of 18 to 21. Cow dung has a slightly lower fraction of low and a slightly higher fraction of high compared to other fuels studied.
As shown in the pie charts in Fig. 3, the CxHyOz family is the main group measured by the EESI-TOF with a contribution from 80 % to 97 %. The N-containing species have the highest contribution (19.6 %) in the POA from cow dung open burning, which is much higher than other fuels in this study (2.5 % to 8.9 %). Of the N-containing species in cow dung POA, 95 % contain one nitrogen atom and are in a wide range of carbon numbers between 5 and 22. They are mainly in the range of < 0.15 to 0.5 and the from 1.2 to > 1.7 (Fig. S4). The degree of unsaturation, calculated from the ratio of the double-bond equivalent to the number of carbons (DBE / C). The difference in all the studied POAs is not major (Fig. S5).
On a molecular level, C6H10O5 ( 162.0523) is most abundant in wood combustion (20.3 % ± 6.1 %) and is less pronounced in straw (15.0 % ± 7.9 %) and even less so in cow dung emissions (9.9 % ± 5.4 %) (Fig. 2c). It could be mainly assigned to levoglucosan (or similar dehydrated sugars), which is formed from the pyrolysis of cellulose and hemicellulose (Simoneit, 2002). The second-most abundant species presented in the POAs is C8H12O6 ( 204.0628) in this study, contributing between 2 % and 9 %. It has been observed from the primary biomass burning emissions in the laboratory and ambient studies (Kumar et al., 2022; Kong et al., 2021). In addition, compounds with 18 and 20 carbon atoms are rich in many fuel types, particularly in spruce and pine burning emissions, and are notably minimal in beech logs.
The (calculated as the ratio of total oxygen to total carbon) of the POAs from five types of burning measured by the EESI-TOF is 0.32 ± 0.07 to 0.41 ± 0.02, which is higher than that of the AMS (0.16 ± 0.07 to 0.37 ± 0.08). The difference likely occurs because the EESI-TOF is insensitive to species with low water solubility and/or low affinity for Na+ (e.g., hydrocarbons including polyaromatic hydrocarbons). This will contribute to an underestimation of . The total nitrogen-to-total carbon ratio () of cow dung measured by EESI-TOF is 0.019, which is slightly higher than that in the AMS measurements (0.015). This could be partially because of the difference in EESI sensitivity to the N-containing molecules. Another reason is that the total carbon from POA measured by the EESI-TOF is smaller, again consistent with the absence of non-water-soluble substances or molecules that do not bind to Na+.
3.3 Literature markers for solid-fuel combustion
Levoglucosan and dehydrated sugars having the molecular formula C6H10O5 are commonly used as tracers for biomass burning. A range of values for the fraction of C6H10O5 is observed both for the same fuel type under different burning conditions and for different fuel types, as seen in Fig. 2c. Thus, C6H10O5 is a good untargeted marker for biomass burning but cannot be used to determine the specific source (or type of combustible) responsible for biomass burning emissions. Likewise, the C8H12O6 is not a suitable marker for specific emission sources, as it is prevalent in all burning of biomass material. Additionally, C8H12O6 has been regarded as a tracer for terpene or syringol-derived SOA (Szmigielski et al., 2007; Yee et al., 2013); however, our results suggest this molecular formula is not a good marker for SOAs due to the strong contribution from biomass burning-derived POA.
At a higher mass range, C16H32O2 and C18HO2 are likely to be the common saturated and unsaturated fatty acids corresponding to palmitic, linolenic, linoleic, and oleic acid, which are important structural components of cells and have been found in the emission of cooking, biomass burning, and cow dung (Simoneit, 2002; Neves et al., 2009a, b; Brown et al., 2021). The corresponding compounds for the C20H30O2 and C20H28O2 are most likely resin acids (e.g., abietic acid and pimaric acid) and dehydroabietic acid, which have been specifically found in coniferous resin (Holmbom, 1977; Simoneit, 2002) and served as biomass burning tracers (Simoneit et al., 1993; Liang et al., 2021). The C20HO2,3 have been found as diterpenoids from the wood of Cunninghamia konishii (Li and Kuo, 2002). This plant species belongs to the class of Pinopsida, which also includes spruce and pine.
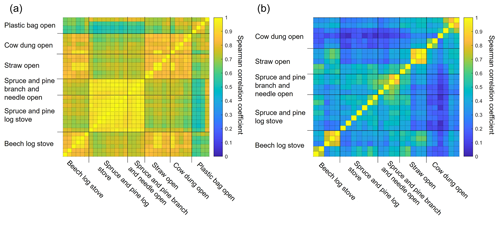
Figure 4The correlation matrix of POAs measured with (a) the HR data from the AMS and (b) EESI-TOF using Spearman's correlation function. Note that some experiments had neither AMS nor EESI-TOF data.
These typical markers stated above are well-known, but due to their presence in more than one fuel, the determination of different biomass burning (BB) sources (or even biomass burning-derived POA) is challenging. For example, scaling levoglucosan to total BB OM requires a priori knowledge of the BB source and burning condition (Favez et al., 2010). Therefore, it is complicated to apply these markers in the source apportionment without comparison to statistically rule out other possibilities.
3.4 Identification of potential markers for specific solid fuels
To investigate the feasibility of distinguishing differences between the combustion fuel sources based on the measured species, the similarity of mass spectra acquired from each experiment by the AMS and EESI-TOF is assessed with Spearman's rank correlation coefficient (r), as shown in Fig. 4. The calculation of Spearman's coefficient is equivalent to calculating the Pearson correlation coefficient on the rank-ordered data, so it assesses monotonic relationships for ions from two mass spectra. In the correlation matrix with the fragment ions from the AMS (Fig. 4a), it is clear that the POAs from the same burning fuel strongly correlate. For instance, the average correlation coefficients of the AMS POA MS for all experiments using the same fuel range from 0.84 to 0.95. When comparing different fuels, a strong correlation is also found between spruce and pine log stove burning and spruce and pine branch and needle open burning (0.95 ± 0.02). This is mainly because these two types of burning are closely related (i.e., derived from the same plants) and therefore have similar chemical composition. The correlation weakened when comparing POAs from different materials (e.g., spruce – beech 0.77 ± 0.03; spruce – straw 0.76 ± 0.03; spruce – cow dung 0.75 ± 0.03).
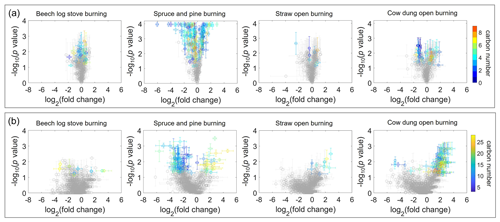
Figure 5The statistic p value vs. fold change with the dataset from the AMS in (a) and EESI-TOF in (b). The color bars are the number of carbon atoms. The horizontal error bars are the 1 standard deviation given by the p-value variations in the pairwise tests, and the vertical error bars are the 1 standard deviation of the log2 (fold change).
By contrast, the correlation coefficients based on the species from EESI-TOF are much lower among different burning fuels and even amongst the same fuel type (0.44 to 0.68). Noticeably, only a weak intra-fuel correlation is found for spruce/pine log stove burning (0.44 ± 0.07), indicating that there are significant differences between experiments which are likely driven by burn-to-burn variability caused by differences in the combustion condition or variance of the fuel materials (e.g., with or without bark, amount of sap in the wood). Nevertheless, the variability between different fuels is clearly larger than the intra-fuel variability for the POAs. For example, the correlation between the cow dung and all the other fuels (average 0.27 ± 0.11) is significantly lower than that among cow dung emissions (0.49 ± 0.16). This suggests that the EESI-TOF may be capable of distinguishing between different types of BB fuels.
To perform a more detailed analysis and identify markers between the emissions, the Mann–Whitney U test (see Sect. 2.2) of the POAs from different fuels measured by the AMS and EESI-TOF is conducted. Considering that both spruce and pine logs as well as spruce and pine branches and needles are similar fuel types and have a comparable POA composition in Figs. 1 to 3, they were classified as the same fuel for this test. Results of the Mann–Whitney U test are presented in Fig. 5, where we show the average −log10 of the p value as a function of the log2 of the fold change (FC). Species having p values less than 0.1 in the two-tailed test in all pairwise comparisons are considered to be significantly more prevalent or scarcer in a single fuel compared to all other fuels. These ions are represented as colored circles in Fig. 5. If the species fail to meet the criterion one time or more than one time, those species will be shown as gray circles even though their average p value might be lower than 0.1. A higher −log10 (p value) (i.e., a lower p value) indicates a lower probability that the fractional medians of two species are equal. At the same time, a higher FC (Eq. 4) indicates a higher abundance of the species' fractional contribution in the tested fuel compared to the average of all other fuels, deeming it more exclusive. In the case of beech logs as well as spruce and pine log burning, the colored p value is lower (higher −log10 (p value)) in the dataset of the AMS than that of EESI-TOF, suggesting the results from the AMS are more replicable. However, from the perspective of FC, its absolute value is around 2 to 4 times higher in the dataset of the EESI-TOF than that of the AMS. This shows that the potential markers selected from the EESI-TOF measurement are more unique and in some cases are found only in the spectrum of a given source. On these grounds, the AMS and EESI-TOF are potent complementary tools to provide separation and source apportionment of ambient OA and to capture marker compounds. The selected potential markers, p values, and fold changes are listed in Tables S2 and S3 for EESI-TOF and AMS data, respectively.
Mass defect plots of the selected marker compounds are visualized in Fig. 6. Many more potential markers are identified from spruce and pine burning as well as cow dung open burning in comparison to beech and straw burning. As shown in Fig. 6a with the AMS dataset, potential markers from the CxHy and CxHyOz family have a significantly higher fraction in the POA of beech logs than those in other fuels. By contrast, the selected markers for spruce and pine burning are more oxidized and mainly composed of the CxHyOz family, which is consistent with its bulk chemical composition and relatively higher . The main fragments CO+ and CO have higher contributions in spruce and pine burning (also can be seen in Fig. 1), but their FCs are not very high, which means they are not exclusive in spruce and pine and therefore are not applicable as sole tracers in the complex ambient air. Fragments from cow dung open burning have a considerably higher contribution in the CxHy family and N-containing families but lower contributions in oxygen-containing species, which also agrees with bulk chemical composition characteristics.
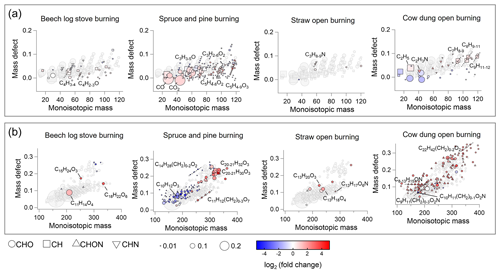
Figure 6The mass defect plot with the dataset from the AMS in (a) and EESI-TOF in (b). The markers denote the fragments or molecules having the same formula. They are sized by the square root of fractional contribution and colored by the log2 (fold change).
Similarly, many marker compounds are determined in the measurement of EESI-TOF for spruce and pine burning as well as cow dung open burning. Compounds with 20–21 carbon atoms as shown in Fig. 6b for spruce and pine burning could be resin and conifer-needle-related, such as C20H32O3 (likely isocupressic acid) (Mofikoya et al., 2020; Wiyono et al., 2006). However, C20H30O2 mentioned in a previous section with notable abundance is not stably emitted in each spruce and pine burn. Therefore, it is not determined as a marker for spruce and pine. Homologues of C11H12(CH2)0−3O7 are also determined, of which C14H18O7 could be picein, which is an important phenolic compound in the needles of spruce (Løkke, 1990). On the other hand, some compounds which are barely present in the POA of spruce and pine burning, such as C14H28(CH2)0−3O2 (likely saturated fatty acids), offer an alternative perspective of exclusion method in source separation. Noticeably, while coniferyl alcohol (C10H12O3) is a major pyrolysis product from softwood (e.g., spruce) lignins (Saiz-Jimenez and De Leeuw, 1986) and has a decent fractional contribution in the POA of the spruce and pine burning, its contribution in spruce and pine burning is smaller than in straw burning. Therefore, it is not recommended as a tracer when other biomass fuels are present. For the hardwood (i.e., beech logs in this study), sinapyl alcohol (C11H14O4) is one of the prominent products from the pyrolysis of lignins (Saiz-Jimenez and De Leeuw, 1986), and it is conspicuous in our beech log stove burning. Interestingly, the nitrogen-containing compound C13H17NO6 is noted as a tracer for straw open burning, and the nitrogen-containing fragments C3H8−9N are also selected from the straw AMS analysis.
Cow dung is a clearly different fuel to other biomass fuels in this study; thus, many markers are identified from cow dung open burning. These potential markers have mostly nitrogen in chemical composition and with generally higher FC. Many series of N-containing homologues are found, such as C10H9NO2 and C11H11NO2, which could be likely assigned to the derivative of indole, i.e., indole acetic acid and indolepropionic acid, respectively. Another series of homologues is C9H11NO2 and C10H13NO2, which have been found especially in the emissions from cow dung cook fire in India compared to brushwood cook fire (Fleming et al., 2018). Homologues without nitrogen atoms in the chemical composition are also seen, for example, C22H42(CH2)0−2O2, likely the homologues of erucic acid, which is a natural fatty oil mainly present in the Brassicaceae family of plants. Nevertheless, it is not very surprising to see the biomass-related species as cows are herbivorous animals.
From the perspective of source apportionment, ions that are primarily associated with a specific emission source and exhibit minimal contribution from other sources can be regarded as potent in use. To show the ability of these markers for source separation, the contribution of two markers for the same source from Tables S2 and S3 that possess a small p value with high FC are plotted among studied fuels. As shown in Fig. S6, these markers measured by the AMS have relatively higher contribution in one specific fuel, which makes the fuel distinctive from others. Nonetheless, one would need to coordinate with more tracers to draw a conclusive diagnosis because the presence of these markers in other fuels. Given this scenario, the markers that have a significantly low contribution (FC < 1) in a specific fuel could shed the lights on. In contrast, markers observed from the EESI-TOF are more robust for utilization as most of them are unique. As the markers listed in Tables S2 and S3 are many, we could not thoroughly discuss here. However, due to the limited fuel types, referencing more markers can provide more confidence in source identification.
To the best of our knowledge, most of these markers are reported for the first time in POA emissions from the studied fuels with the EESI-MS, which is becoming more commonly used in the measurement of atmospheric aerosols. They could improve the refinement in source separation of fuels in biomass burning. Replicability and specificity are two important criteria for tracers. The p value being less than 0.1 in the two-tailed test can ensure the stability of the results shown. The FC indicates the degree of specificity of markers of one fuel in the presence of other fuels. If the p-value criterion is satisfied and the FC is large, the presence of this marker can directly lead us to the emission source. On the other hand, if the FC is less than 1, the detection of this compound can decisively exclude the related source after verifying this is not due to a detection limit issue. However, if the FC is in-between, more caution is needed because these compounds do not have a distinctive fraction in that fuel compared to other fuels but could have a relatively fixed ratio compared to other markers.
In this study, we conducted 36 burning experiments to simulate typical solid fuel combustion emission, including residential burning (beech or spruce and pine log stove burning), wildfire (spruce and pine branch and needle open burning), agricultural residue in field burning (straw open burning), cow dung open burning, and plastic bag open burning. The emission factors of CO, CO2, THC, PM, OM, and BC were determined. The chemical composition of particles emitted from the combustion processes was comprehensively characterized using the AMS and the EESI-TOF, and the chemical composition of the particles measured by the two instruments were compared. These are the first direct measurements of these source profiles with the EESI-TOF. The utility of traditional markers is discussed, and new potential markers were identified using the Mann–Whitney U test.
The EFs of CO and THC are generally higher during the low combustion efficiency, and the opposite is true for the EF of CO2. The highest EF of PM (16.6 ± 10.8 g kg−1) is from cow dung open burning, which is mostly OM (16.2 ± 10.8 g kg−1), but for residential and plastic bag burning, the eBC accounts for ∼ 30 % of the total PM. The organics measured by the AMS show that the wood (beech, spruce, and pine) burning emission has a relatively higher abundance of CxHyOz fragments, while straw and cow dung burning emissions are dominated by CxHy fragments in their POAs. On the molecular level, C6H10O5 has the highest proportion (∼ 7 % to ∼ 30 %) in the POAs measured by the EESI-TOF (except for the plastic bag burning), followed by C8H12O6 with fractions of ∼ 2 % to ∼ 9 %. The chemical composition measured with the AMS covers a wide range of non-refractory organic and inorganic components. However, the extensive fragmentation concentrates the measured mass-to-charge ratio below ∼ 120 and limits its chemical resolution. The chemical groups used to deduce the composition of particles could originate from different compounds, which impedes us from seeing the full picture. The formula-based mass spectrum from the EESI-TOF overcomes this deficiency and thus reveals the detailed characteristics.
However, many compounds are present ubiquitously in all of the fuels used here, making it challenging to identify atmospheric sources solely by visual comparisons of the full mass spectra. By using the Mann–Whitney U test to identify potential markers among the studied fuels, we find that the markers identified by the AMS have greater replicability and those identified by EESI-TOF are more distinctive, thus providing an important reference for the source apportionment. Overall, this work highlights the complex characteristics of POAs emitted from the burning of solid fuels and proposes the markers for separating different sources using the AMS and EESI-TOF. This work shows mass spectral profiles of burning emissions on bulk and molecular level, which improves our understanding of POA from different fuels. The markers provided in this study are crucial for distinguishing the sources of aerosols in the atmosphere and enhancing the interpretation of source apportionment. In the future, the volatility and chemical reactivity of the proposed markers should be tested to determine their atmospheric stability and their ability to be a robust marker. More burning fuels such as coal and grass could be conducted to enrich the spectral database.
Future studies will probe the usefulness of these markers if they are long-lived enough in the atmosphere to provide useful separation between the complex emission sources shown here. This will either focus on online measurements in polluted regions or from offline filter analysis from similar regions. Clearly, the dominant biomass burning markers (levoglucosan and others) are not robust enough to be used to separate different biomass sources, though they are robust enough for identification of general biomass burning aerosols. Nitrogen containing compounds emitted from cow dung emissions can provide a very unique set of markers for separating this source from other biomass sources. Additionally, resin acids observed in the emissions from spruce and pine provide unique species associated with these emissions (and were observed previously).
At the present moment, to provide insight into the usefulness of these markers within the context of ambient measurements or against source apportionment methods, we would require a robust dataset of comparable data to test these markers and average emission profiles against.
The data presented in the text and figures are available in the Zenodo online repository (https://doi.org/10.5281/zenodo.10126682, Zhang et al., 2023).
The supplement related to this article is available online at: https://doi.org/10.5194/acp-23-14561-2023-supplement.
JZ, TTW, KL, DMB, EG, RKYC, and SB conducted the burning experiments. JZ analyzed the data and wrote the paper. DSW, MS, TQC, LQ, and DB participated in the campaign. DMB, KL, IEH, HL, JGS, and ASHP participated in the interpretation of data.
The contact author has declared that none of the authors has any competing interests.
Publisher’s note: Copernicus Publications remains neutral with regard to jurisdictional claims made in the text, published maps, institutional affiliations, or any other geographical representation in this paper. While Copernicus Publications makes every effort to include appropriate place names, the final responsibility lies with the authors.
We would like to thank Pascal André Schneider from the Paul Scherrer Institute for building the holding tank and burning platform.
This research has been supported by the Schweizerischer Nationalfonds zur Förderung der Wissenschaftlichen Forschung (grant no. 200020_188624), Horizon 2020 (grant no. 101008004), the H2020 Marie Skłodowska-Curie Actions (grant no. 884104), and the Swiss National Science Foundation under the SNSF Joint Research Project (grant no. 189883) and the SNSF project (grant no. 206021_198140). PSI's atmospheric simulation chamber is a facility of the ACTRIS ERIC and receives funding from the Swiss State Secretariat for Education, Research and Innovation (SERI grant). Funding was also provided by the European Union's Horizon 2020 Research and Innovation program under a Marie Skłodowska-Curie grant agreement (grant no. 701647) and the Schweizerischer Nationalfonds zur Förderung der Wissenschaftlichen Forschung (HAZECHINA (grant no. IZLCZ2_169986)).
This paper was edited by Arthur Chan and reviewed by Yutong Liang and three anonymous referees.
Adam, M. G., Tran, P. T. M., Bolan, N., and Balasubramanian, R.: Biomass burning-derived airborne particulate matter in Southeast Asia: A critical review, J. Hazard Mater., 407, 124760, https://doi.org/10.1016/j.jhazmat.2020.124760, 2021.
Aiken, A. C., DeCarlo, P. F., and Jimenez, J. L.: Elemental Analysis of Organic Species with Electron Ionization High-Resolution Mass Spectrometry, Anal. Chem., 79, 8350–8358, https://doi.org/10.1021/ac071150w, 2007.
Alfarra, M. R., Prevot, A. S. H., Szidat, S., Sandradewi, J., Weimer, S., Lanz, V. A., Schreiber, D., Mohr, M., and Baltensperger, U.: Identification of the Mass Spectral Signature of Organic Aerosols from Wood Burning Emissions, Environ. Sci. Technol., 41, 5770–5777, https://doi.org/10.1021/es062289b, 2007.
Andreae, M. O.: Emission of trace gases and aerosols from biomass burning – an updated assessment, Atmos. Chem. Phys., 19, 8523–8546, https://doi.org/10.5194/acp-19-8523-2019, 2019.
Bahreini, R., Keywood, M. D., Ng, N. L., Varutbangkul, V., Gao, S., Flagan, R. C., Seinfeld, J. H., Worsnop, D. R., and Jimenez, J. L.: Measurements of Secondary Organic Aerosol from Oxidation of Cycloalkenes, Terpenes, and m-Xylene Using an Aerodyne Aerosol Mass Spectrometer, Environ. Sci. Technol., 39, 5674–5688, https://doi.org/10.1021/es048061a, 2005.
Bell, D. M., Wu, C., Bertrand, A., Graham, E., Schoonbaert, J., Giannoukos, S., Baltensperger, U., Prevot, A. S. H., Riipinen, I., El Haddad, I., and Mohr, C.: Particle-phase processing of α-pinene NO3 secondary organic aerosol in the dark, Atmos. Chem. Phys., 22, 13167–13182, https://doi.org/10.5194/acp-22-13167-2022, 2022.
Bertrand, A., Stefenelli, G., Bruns, E. A., Pieber, S. M., Temime-Roussel, B., Slowik, J. G., Prévôt, A. S. H., Wortham, H., El Haddad, I., and Marchand, N.: Primary emissions and secondary aerosol production potential from woodstoves for residential heating: Influence of the stove technology and combustion efficiency, Atmos. Environ., 169, 65–79, 2017.
Bertrand, A., Stefenelli, G., Jen, C. N., Pieber, S. M., Bruns, E. A., Ni, H., Temime-Roussel, B., Slowik, J. G., Goldstein, A. H., El Haddad, I., Baltensperger, U., Prévôt, A. S. H., Wortham, H., and Marchand, N.: Evolution of the chemical fingerprint of biomass burning organic aerosol during aging, Atmos. Chem. Phys., 18, 7607–7624, https://doi.org/10.5194/acp-18-7607-2018, 2018.
Bond, T. C., Bhardwaj, E., Dong, R., Jogani, R., Jung, S., Roden, C., Streets, D. G., and Trautmann, N. M.: Historical emissions of black and organic carbon aerosol from energy-related combustion, 1850–2000, Global Biogeochem. Cy., 21, https://doi.org/10.1029/2006GB002840, 2007.
Brown, W. L., Day, D. A., Stark, H., Pagonis, D., Krechmer, J. E., Liu, X., Price, D. J., Katz, E. F., DeCarlo, P. F., Masoud, C. G., Wang, D. S., Hildebrandt Ruiz, L., Arata, C., Lunderberg, D. M., Goldstein, A. H., Farmer, D. K., Vance, M. E., and Jimenez, J. L.: Real-time organic aerosol chemical speciation in the indoor environment using extractive electrospray ionization mass spectrometry, Indoor Air, 31, 141–155, 2021.
Bruns, E. A., Slowik, J. G., El Haddad, I., Kilic, D., Klein, F., Dommen, J., Temime-Roussel, B., Marchand, N., Baltensperger, U., and Prévôt, A. S. H.: Characterization of gas-phase organics using proton transfer reaction time-of-flight mass spectrometry: fresh and aged residential wood combustion emissions, Atmos. Chem. Phys., 17, 705–720, https://doi.org/10.5194/acp-17-705-2017, 2017.
Canagaratna, M. R., Jimenez, J. L., Kroll, J. H., Chen, Q., Kessler, S. H., Massoli, P., Hildebrandt Ruiz, L., Fortner, E., Williams, L. R., Wilson, K. R., Surratt, J. D., Donahue, N. M., Jayne, J. T., and Worsnop, D. R.: Elemental ratio measurements of organic compounds using aerosol mass spectrometry: characterization, improved calibration, and implications, Atmos. Chem. Phys., 15, 253–272, https://doi.org/10.5194/acp-15-253-2015, 2015.
Census of India: Households by Availability of Separate Kitchen and Type of Fuel Used for Cooking, available at: https://censusindia.gov.in/census.website/data/census-tables (last access: 2 November 2023), 2011.
Chen, G., Sosedova, Y., Canonaco, F., Fröhlich, R., Tobler, A., Vlachou, A., Daellenbach, K. R., Bozzetti, C., Hueglin, C., Graf, P., Baltensperger, U., Slowik, J. G., El Haddad, I., and Prévôt, A. S. H.: Time-dependent source apportionment of submicron organic aerosol for a rural site in an alpine valley using a rolling positive matrix factorisation (PMF) window, Atmos. Chem. Phys., 21, 15081–15101, https://doi.org/10.5194/acp-21-15081-2021, 2021.
Chen, J., Li, C., Ristovski, Z., Milic, A., Gu, Y., Islam, M. S., Wang, S., Hao, J., Zhang, H., He, C., Guo, H., Fu, H., Miljevic, B., Morawska, L., Thai, P., Lam, Y. F., Pereira, G., Ding, A., Huang, X., and Dumka, U. C.: A review of biomass burning: Emissions and impacts on air quality, health and climate in China, Sci. Total Environ., 579, 1000–1034, 2017.
Colombini, M. P., Modugno, F., and Ribechini, E.: Direct exposure electron ionization mass spectrometry and gas chromatography/mass spectrometry techniques to study organic coatings on archaeological amphorae, J. Mass Spectrom., 40, 675–687, https://doi.org/10.1002/jms.841, 2005.
Crippa, M., Canonaco, F., Lanz, V. A., Äijälä, M., Allan, J. D., Carbone, S., Capes, G., Ceburnis, D., Dall'Osto, M., Day, D. A., DeCarlo, P. F., Ehn, M., Eriksson, A., Freney, E., Hildebrandt Ruiz, L., Hillamo, R., Jimenez, J. L., Junninen, H., Kiendler-Scharr, A., Kortelainen, A.-M., Kulmala, M., Laaksonen, A., Mensah, A. A., Mohr, C., Nemitz, E., O'Dowd, C., Ovadnevaite, J., Pandis, S. N., Petäjä, T., Poulain, L., Saarikoski, S., Sellegri, K., Swietlicki, E., Tiitta, P., Worsnop, D. R., Baltensperger, U., and Prévôt, A. S. H.: Organic aerosol components derived from 25 AMS data sets across Europe using a consistent ME-2 based source apportionment approach, Atmos. Chem. Phys., 14, 6159–6176, https://doi.org/10.5194/acp-14-6159-2014, 2014.
Cubison, M. J., Ortega, A. M., Hayes, P. L., Farmer, D. K., Day, D., Lechner, M. J., Brune, W. H., Apel, E., Diskin, G. S., Fisher, J. A., Fuelberg, H. E., Hecobian, A., Knapp, D. J., Mikoviny, T., Riemer, D., Sachse, G. W., Sessions, W., Weber, R. J., Weinheimer, A. J., Wisthaler, A., and Jimenez, J. L.: Effects of aging on organic aerosol from open biomass burning smoke in aircraft and laboratory studies, Atmos. Chem. Phys., 11, 12049–12064, https://doi.org/10.5194/acp-11-12049-2011, 2011.
Daellenbach, K. R., Uzu, G., Jiang, J., Cassagnes, L.-E., Leni, Z., Vlachou, A., Stefenelli, G., Canonaco, F., Weber, S., Segers, A., Kuenen, J. J. P., Schaap, M., Favez, O., Albinet, A., Aksoyoglu, S., Dommen, J., Baltensperger, U., Geiser, M., El Haddad, I., Jaffrezo, J.-L., and Prévôt, A. S. H.: Sources of particulate-matter air pollution and its oxidative potential in Europe, Nature, 587, 414–419, https://doi.org/10.1038/s41586-020-2902-8, 2020.
de Oliveira Alves, N., Vessoni, A. T., Quinet, A., Fortunato, R. S., Kajitani, G. S., Peixoto, M. S., Hacon, S. S., Artaxo, P., Saldiva, P., Menck, C. F. M., and Batistuzzo de Medeiros, S. R.: Biomass burning in the Amazon region causes DNA damage and cell death in human lung cells, Sci. Rep., 7, 10937, https://doi.org/10.1038/s41598-017-11024-3, 2017.
DeCarlo, P. F., Kimmel, J. R., Trimborn, A., Northway, M. J., Jayne, J. T., Aiken, A. C., Gonin, M., Fuhrer, K., Horvath, T., Docherty, K. S., Worsnop, D. R., and Jimenez, J. L.: Field-Deployable, High-Resolution, Time-of-Flight Aerosol Mass Spectrometer, Anal. Chem., 78, 8281–8289, https://doi.org/10.1021/ac061249n, 2006.
Dzepina, K., Arey, J., Marr, L. C., Worsnop, D. R., Salcedo, D., Zhang, Q., Onasch, T. B., Molina, L. T., Molina, M. J., and Jimenez, J. L.: Detection of particle-phase polycyclic aromatic hydrocarbons in Mexico City using an aerosol mass spectrometer, Int. J. Mass Spectrom., 263, 152–170, https://doi.org/10.1016/j.ijms.2007.01.010, 2007.
Fang, Z., Deng, W., Zhang, Y., Ding, X., Tang, M., Liu, T., Hu, Q., Zhu, M., Wang, Z., Yang, W., Huang, Z., Song, W., Bi, X., Chen, J., Sun, Y., George, C., and Wang, X.: Open burning of rice, corn and wheat straws: primary emissions, photochemical aging, and secondary organic aerosol formation, Atmos. Chem. Phys., 17, 14821–14839, https://doi.org/10.5194/acp-17-14821-2017, 2017.
Farmer, D. K., Matsunaga, A., Docherty, K. S., Surratt, J. D., Seinfeld, J. H., Ziemann, P. J., and Jimenez, J. L.: Response of an aerosol mass spectrometer to organonitrates and organosulfates and implications for atmospheric chemistry, P. Natl. Acad. Sci. USA, 107, 6670–6675, 2010.
Favez, O., El Haddad, I., Piot, C., Boréave, A., Abidi, E., Marchand, N., Jaffrezo, J.-L., Besombes, J.-L., Personnaz, M.-B., Sciare, J., Wortham, H., George, C., and D'Anna, B.: Inter-comparison of source apportionment models for the estimation of wood burning aerosols during wintertime in an Alpine city (Grenoble, France), Atmos. Chem. Phys., 10, 5295–5314, https://doi.org/10.5194/acp-10-5295-2010, 2010.
Fleming, L. T., Lin, P., Laskin, A., Laskin, J., Weltman, R., Edwards, R. D., Arora, N. K., Yadav, A., Meinardi, S., Blake, D. R., Pillarisetti, A., Smith, K. R., and Nizkorodov, S. A.: Molecular composition of particulate matter emissions from dung and brushwood burning household cookstoves in Haryana, India, Atmos. Chem. Phys., 18, 2461–2480, https://doi.org/10.5194/acp-18-2461-2018, 2018.
Font-Palma, C.: Methods for the Treatment of Cattle Manure – A Review, C, 5, 27–47, https://doi.org/10.3390/c5020027, 2019.
Goetz, J. D., Giordano, M. R., Stockwell, C. E., Christian, T. J., Maharjan, R., Adhikari, S., Bhave, P. V., Praveen, P. S., Panday, A. K., Jayarathne, T., Stone, E. A., Yokelson, R. J., and DeCarlo, P. F.: Speciated online PM1 from South Asian combustion sources – Part 1: Fuel-based emission factors and size distributions, Atmos. Chem. Phys., 18, 14653–14679, https://doi.org/10.5194/acp-18-14653-2018, 2018.
Haque, Md. M., Kawamura, K., Deshmukh, D. K., Fang, C., Song, W., Mengying, B., and Zhang, Y.-L.: Characterization of organic aerosols from a Chinese megacity during winter: predominance of fossil fuel combustion, Atmos. Chem. Phys., 19, 5147–5164, https://doi.org/10.5194/acp-19-5147-2019, 2019.
Hennigan, C. J., Miracolo, M. A., Engelhart, G. J., May, A. A., Presto, A. A., Lee, T., Sullivan, A. P., McMeeking, G. R., Coe, H., Wold, C. E., Hao, W.-M., Gilman, J. B., Kuster, W. C., de Gouw, J., Schichtel, B. A., Collett Jr., J. L., Kreidenweis, S. M., and Robinson, A. L.: Chemical and physical transformations of organic aerosol from the photo-oxidation of open biomass burning emissions in an environmental chamber, Atmos. Chem. Phys., 11, 7669–7686, https://doi.org/10.5194/acp-11-7669-2011, 2011.
Hoffer, A., Jancsek-Turóczi, B., Tóth, Á., Kiss, G., Naghiu, A., Levei, E. A., Marmureanu, L., Machon, A., and Gelencsér, A.: Emission factors for PM10 and polycyclic aromatic hydrocarbons (PAHs) from illegal burning of different types of municipal waste in households, Atmos. Chem. Phys., 20, 16135–16144, https://doi.org/10.5194/acp-20-16135-2020, 2020.
Holmbom, B.: Improved gas chromatographic analysis of fatty and resin acid mixtures with special reference to tall oil, J. Am. Oil Chem. Soc., 54, 289–293, https://doi.org/10.1007/BF02671098, 1977.
Huang, R. J., Zhang, Y., Bozzetti, C., Ho, K. F., Cao, J. J., Han, Y., Daellenbach, K. R., Slowik, J. G., Platt, S. M., Canonaco, F., Zotter, P., Wolf, R., Pieber, S. M., Bruns, E. A., Crippa, M., Ciarelli, G., Piazzalunga, A., Schwikowski, M., Abbaszade, G., Schnelle-Kreis, J., Zimmermann, R., An, Z., Szidat, S., Baltensperger, U., El Haddad, I., and Prevot, A. S.: High secondary aerosol contribution to particulate pollution during haze events in China, Nature, 514, 218–222, 2014.
Islam, M. R., Welker, J., Salam, A., and Stone, E. A.: Plastic Burning Impacts on Atmospheric Fine Particulate Matter at Urban and Rural Sites in the USA and Bangladesh, ACS Environ. Au, https://doi.org/10.1021/acsenvironau.1c00054, 2022.
Jayarathne, T., Stockwell, C. E., Bhave, P. V., Praveen, P. S., Rathnayake, C. M., Islam, Md. R., Panday, A. K., Adhikari, S., Maharjan, R., Goetz, J. D., DeCarlo, P. F., Saikawa, E., Yokelson, R. J., and Stone, E. A.: Nepal Ambient Monitoring and Source Testing Experiment (NAMaSTE): emissions of particulate matter from wood- and dung-fueled cooking fires, garbage and crop residue burning, brick kilns, and other sources, Atmos. Chem. Phys., 18, 2259–2286, https://doi.org/10.5194/acp-18-2259-2018, 2018.
Jen, C. N., Hatch, L. E., Selimovic, V., Yokelson, R. J., Weber, R., Fernandez, A. E., Kreisberg, N. M., Barsanti, K. C., and Goldstein, A. H.: Speciated and total emission factors of particulate organics from burning western US wildland fuels and their dependence on combustion efficiency, Atmos. Chem. Phys., 19, 1013–1026, https://doi.org/10.5194/acp-19-1013-2019, 2019.
Ji, X., Le Bihan, O., Ramalho, O., Mandin, C., D'Anna, B., Martinon, L., Nicolas, M., Bard, D., and Pairon, J. C.: Characterization of particles emitted by incense burning in an experimental house, Indoor Air, 20, 147–158, 2010.
Kong, X., Salvador, C. M., Carlsson, S., Pathak, R., Davidsson, K. O., Le Breton, M., Gaita, S. M., Mitra, K., Hallquist, A. M., Hallquist, M., and Pettersson, J. B. C.: Molecular characterization and optical properties of primary emissions from a residential wood burning boiler, Sci. Total Environ., 754, 142143, 2021.
Kumar, V., Giannoukos, S., Haslett, S. L., Tong, Y., Singh, A., Bertrand, A., Lee, C. P., Wang, D. S., Bhattu, D., Stefenelli, G., Dave, J. S., Puthussery, J. V., Qi, L., Vats, P., Rai, P., Casotto, R., Satish, R., Mishra, S., Pospisilova, V., Mohr, C., Bell, D. M., Ganguly, D., Verma, V., Rastogi, N., Baltensperger, U., Tripathi, S. N., Prévôt, A. S. H., and Slowik, J. G.: Highly time-resolved chemical speciation and source apportionment of organic aerosol components in Delhi, India, using extractive electrospray ionization mass spectrometry, Atmos. Chem. Phys., 22, 7739–7761, https://doi.org/10.5194/acp-22-7739-2022, 2022.
Li, C.-T., Zhuang, H.-K., Hsieh, L.-T., Lee, W.-J., and Tsao, M.-C.: PAH emission from the incineration of three plastic wastes, Environ. Int., 27, 61–67, 2001.
Li, X., Wang, S., Duan, L., Hao, J., Li, C., Chen, Y., and Yang, L.: Particulate and Trace Gas Emissions from Open Burning of Wheat Straw and Corn Stover in China, Environ. Sci. Technol., 41, 6052–6058, https://doi.org/10.1021/es0705137, 2007.
Li, Y.-C. and Kuo, Y.-H.: Labdane-type diterpenoids from the wood of Cunninghamia konishii, Chem. Pharm. Bull., 50, 498–500, https://doi.org/10.1248/cpb.50.498, 2002.
Liang, Y., Jen, C. N., Weber, R. J., Misztal, P. K., and Goldstein, A. H.: Chemical composition of PM2.5 in October 2017 Northern California wildfire plumes, Atmos. Chem. Phys., 21, 5719–5737, https://doi.org/10.5194/acp-21-5719-2021, 2021.
Løkke, H.: Picein and piceol concentrations in Norway spruce, Ecotox. Environ. Safe., 19, 301–309, 1990.
Lopez-Hilfiker, F. D., Pospisilova, V., Huang, W., Kalberer, M., Mohr, C., Stefenelli, G., Thornton, J. A., Baltensperger, U., Prevot, A. S. H., and Slowik, J. G.: An extractive electrospray ionization time-of-flight mass spectrometer (EESI-TOF) for online measurement of atmospheric aerosol particles, Atmos. Meas. Tech., 12, 4867–4886, https://doi.org/10.5194/amt-12-4867-2019, 2019.
Lopez-Hilfiker, F. D., Mohr, C., Ehn, M., Rubach, F., Kleist, E., Wildt, J., Mentel, Th. F., Lutz, A., Hallquist, M., Worsnop, D., and Thornton, J. A.: A novel method for online analysis of gas and particle composition: description and evaluation of a Filter Inlet for Gases and AEROsols (FIGAERO), Atmos. Meas. Tech., 7, 983–1001, https://doi.org/10.5194/amt-7-983-2014, 2014.
Mann, H. B. and Whitney, D. R.: On a test of whether one of two random variables is stochastically larger than the other, Ann. Mathe. Stat., 18, 50–60, https://doi.org/10.1214/aoms/1177730491, 1947.
Mofikoya, O. O., Mäkinen, M., and Jänis, J.: Chemical Fingerprinting of Conifer Needle Essential Oils and Solvent Extracts by Ultrahigh-Resolution Fourier Transform Ion Cyclotron Resonance Mass Spectrometry, ACS Omega, 5, 10543–10552, https://doi.org/10.1021/acsomega.0c00901, 2020.
Mohr, C., DeCarlo, P. F., Heringa, M. F., Chirico, R., Slowik, J. G., Richter, R., Reche, C., Alastuey, A., Querol, X., Seco, R., Peñuelas, J., Jiménez, J. L., Crippa, M., Zimmermann, R., Baltensperger, U., and Prévôt, A. S. H.: Identification and quantification of organic aerosol from cooking and other sources in Barcelona using aerosol mass spectrometer data, Atmos. Chem. Phys., 12, 1649–1665, https://doi.org/10.5194/acp-12-1649-2012, 2012.
Neves, L., Ferreira, V., and Oliveira, R.: Co-composting cow manure with food waste: The influence of lipids content, Bioresour. Technol., 100, 1957–1962, https://doi.org/10.1016/j.biortech.2008.10.030, 2009a.
Neves, L., Oliveira, R., and Alves, M. M.: Fate of LCFA in the co-digestion of cow manure, food waste and discontinuous addition of oil, Water Res., 43, 5142–5150, 2009b.
Ng, N. L., Canagaratna, M. R., Zhang, Q., Jimenez, J. L., Tian, J., Ulbrich, I. M., Kroll, J. H., Docherty, K. S., Chhabra, P. S., Bahreini, R., Murphy, S. M., Seinfeld, J. H., Hildebrandt, L., Donahue, N. M., DeCarlo, P. F., Lanz, V. A., Prévôt, A. S. H., Dinar, E., Rudich, Y., and Worsnop, D. R.: Organic aerosol components observed in Northern Hemispheric datasets from Aerosol Mass Spectrometry, Atmos. Chem. Phys., 10, 4625–4641, https://doi.org/10.5194/acp-10-4625-2010, 2010.
Pathak, G., Nichter, M., Hardon, A., Moyer, E., Latkar, A., Simbaya, J., Pakasi, D., Taqueban, E., and Love, J.: Plastic pollution and the open burning of plastic wastes, Global Environ. Change, 80, 102648, https://doi.org/10.1016/j.gloenvcha.2023.102648, 2023.
Qi, L., Bozzetti, C., Corbin, J. C., Daellenbach, K. R., El Haddad, I., Zhang, Q., Wang, J., Baltensperger, U., Prevot, A. S. H., Chen, M., Ge, X., and Slowik, J. G.: Source identification and characterization of organic nitrogen in atmospheric aerosols at a suburban site in China, Sci. Total Environ., 818, 151800, 2022.
Radke, L. and Ward, D., Crutzen, P. J., and Goldammer, J. G. (Eds.): The Ecological, Atmospheric, and Climatic Importance of Vegetation Fires., Environmental Sciences Research Report, John Wiley and Sons, Inc., New York, ISBN 0-471-93604-9, 1993.
Rugiel, M. M., Setkowicz, Z. K., Drozdz, A. K., Janeczko, K. J., Kutorasińska, J., and Chwiej, J. G.: The Use of Fourier Transform Infrared Microspectroscopy for the Determination of Biochemical Anomalies of the Hippocampal Formation Characteristic for the Kindling Model of Seizures, ACS Chem. Neurosci., 12, 4564–4579, https://doi.org/10.1021/acschemneuro.1c00642, 2021.
Saiz-Jimenez, C. and De Leeuw, J. W.: Lignin pyrolysis products: Their structures and their significance as biomarkers, Org. Geochem., 10, 869–876, 1986.
Simoneit, B. R. T.: Biomass burning – a review of organic tracers for smoke from incomplete combustion, Appl. Geochem., 17, 129–162, 2002.
Simoneit, B. R. T., Rogge, W. F., Mazurek, M. A., Standley, L. J., Hildemann, L. M., and Cass, G. R.: Lignin pyrolysis products, lignans, and resin acids as specific tracers of plant classes in emissions from biomass combustion, Environ. Sci. Technol., 27, 2533–2541, https://doi.org/10.1021/es00048a034, 1993.
Smith, K. R., Bruce, N., Balakrishnan, K., Adair-Rohani, H., Balmes, J., Chafe, Z., Dherani, M., Hosgood, H. D., Mehta, S., Pope, D., and Rehfuess, E.: Millions Dead: How Do We Know and What Does It Mean? Methods Used in the Comparative Risk Assessment of Household Air Pollution, Annu. Rev. Public Health, 35, 185–206, https://doi.org/10.1146/annurev-publhealth-032013-182356, 2014.
Stark, H., Yatavelli, R. L. N., Thompson, S. L., Kang, H., Krechmer, J. E., Kimmel, J. R., Palm, B. B., Hu, W., Hayes, P. L., Day, D. A., Campuzano-Jost, P., Canagaratna, M. R., Jayne, J. T., Worsnop, D. R., and Jimenez, J. L.: Impact of Thermal Decomposition on Thermal Desorption Instruments: Advantage of Thermogram Analysis for Quantifying Volatility Distributions of Organic Species, Environ. Sci. Technol., 51, 8491–8500, 2017.
Stockwell, C. E., Christian, T. J., Goetz, J. D., Jayarathne, T., Bhave, P. V., Praveen, P. S., Adhikari, S., Maharjan, R., DeCarlo, P. F., Stone, E. A., Saikawa, E., Blake, D. R., Simpson, I. J., Yokelson, R. J., and Panday, A. K.: Nepal Ambient Monitoring and Source Testing Experiment (NAMaSTE): emissions of trace gases and light-absorbing carbon from wood and dung cooking fires, garbage and crop residue burning, brick kilns, and other sources, Atmos. Chem. Phys., 16, 11043–11081, https://doi.org/10.5194/acp-16-11043-2016, 2016.
Surdu, M., Lamkaddam, H., Wang, D. S., Bell, D. M., Xiao, M., Lee, C. P., Li, D., Caudillo, L., Marie, G., Scholz, W., Wang, M., Lopez, B., Piedehierro, A. A., Ataei, F., Baalbaki, R., Bertozzi, B., Bogert, P., Brasseur, Z., Dada, L., Duplissy, J., Finkenzeller, H., He, X. C., Hohler, K., Korhonen, K., Krechmer, J. E., Lehtipalo, K., Mahfouz, N. G. A., Manninen, H. E., Marten, R., Massabo, D., Mauldin, R., Petaja, T., Pfeifer, J., Philippov, M., Rorup, B., Simon, M., Shen, J., Umo, N. S., Vogel, F., Weber, S. K., Zauner-Wieczorek, M., Volkamer, R., Saathoff, H., Mohler, O., Kirkby, J., Worsnop, D. R., Kulmala, M., Stratmann, F., Hansel, A., Curtius, J., Welti, A., Riva, M., Donahue, N. M., Baltensperger, U., and El Haddad, I.: Molecular Understanding of the Enhancement in Organic Aerosol Mass at High Relative Humidity, Environ. Sci. Technol., 57, 2297–2309, 2023.
Szmigielski, R., Surratt, J. D., Gómez-González, Y., Van der Veken, P., Kourtchev, I., Vermeylen, R., Blockhuys, F., Jaoui, M., Kleindienst, T. E., Lewandowski, M., Offenberg, J. H., Edney, E. O., Seinfeld, J. H., Maenhaut, W., and Claeys, M.: 3-methyl-1,2,3-butanetricarboxylic acid: An atmospheric tracer for terpene secondary organic aerosol, Geophys. Res. Lett., 34, L24811, https://doi.org/10.1029/2007GL031338, 2007.
Tai, K. Y., Dhaliwal, J., and Balasubramaniam, V.: Leveraging Mann–Whitney U test on large-scale genetic variation data for analysing malaria genetic markers, Malar. J., 21, 79, https://doi.org/10.1186/s12936-022-04104-x, 2022.
Tissari, J., Lyyränen, J., Hytönen, K., Sippula, O., Tapper, U., Frey, A., Saarnio, K., Pennanen, A. S., Hillamo, R., Salonen, R. O., Hirvonen, M. R., and Jokiniemi, J.: Fine particle and gaseous emissions from normal and smouldering wood combustion in a conventional masonry heater, Atmos. Environ., 42, 7862–7873, 2008.
Tobler, A., Bhattu, D., Canonaco, F., Lalchandani, V., Shukla, A., Thamban, N. M., Mishra, S., Srivastava, A. K., Bisht, D. S., Tiwari, S., Singh, S., Močnik, G., Baltensperger, U., Tripathi, S. N., Slowik, J. G., and Prévôt, A. S. H.: Chemical characterization of PM2.5 and source apportionment of organic aerosol in New Delhi, India, Sci. Total Environ., 745, 140924, 2020.
Tong, Y., Pospisilova, V., Qi, L., Duan, J., Gu, Y., Kumar, V., Rai, P., Stefenelli, G., Wang, L., Wang, Y., Zhong, H., Baltensperger, U., Cao, J., Huang, R.-J., Prévôt, A. S. H., and Slowik, J. G.: Quantification of solid fuel combustion and aqueous chemistry contributions to secondary organic aerosol during wintertime haze events in Beijing, Atmos. Chem. Phys., 21, 9859–9886, https://doi.org/10.5194/acp-21-9859-2021, 2021.
Tuet, W. Y., Liu, F., de Oliveira Alves, N., Fok, S., Artaxo, P., Vasconcellos, P., Champion, J. A., and Ng, N. L.: Chemical Oxidative Potential and Cellular Oxidative Stress from Open Biomass Burning Aerosol, Environ. Sci. Technol. Lett., 6, 126–132, https://doi.org/10.1021/acs.estlett.9b00060, 2019.
Wang, Y., Wang, Q., Ye, J., Yan, M., Qin, Q., Prévôt, A. S. H., and Cao, J.: A Review of Aerosol Chemical Composition and Sources in Representative Regions of China during Wintertime, Atmosphere, 10, 277, 2019.
Ward, D. E. and Hardy, C. C.: Smoke emissions from wildland fires, Environ. Int., 17, 117–134, 1991.
Wilcoxon, F.: Individual Comparisons by Ranking Methods, Biometrics Bull., 1, 80–83, 1945.
Wiyono, B., Tachibana, S., and Tinambunan, D.: Chemical Compositions of Pine Resin, Rosin and Turpentine Oil from West Java, Indo. J. Forest. Res., 3, 7–17, https://doi.org/10.20886/ijfr.2006.3.1.7-17, 2006.
Wu, D., Li, Q., Shang, X., Liang, Y., Ding, X., Sun, H., Li, S., Wang, S., Chen, Y., and Chen, J.: Commodity plastic burning as a source of inhaled toxic aerosols, J. Hazard Mater., 416, 125820, 2021.
Xu, W., He, Y., Qiu, Y., Chen, C., Xie, C., Lei, L., Li, Z., Sun, J., Li, J., Fu, P., Wang, Z., Worsnop, D. R., and Sun, Y.: Mass spectral characterization of primary emissions and implications in source apportionment of organic aerosol, Atmos. Meas. Tech., 13, 3205–3219, https://doi.org/10.5194/amt-13-3205-2020, 2020.
Yee, L. D., Kautzman, K. E., Loza, C. L., Schilling, K. A., Coggon, M. M., Chhabra, P. S., Chan, M. N., Chan, A. W. H., Hersey, S. P., Crounse, J. D., Wennberg, P. O., Flagan, R. C., and Seinfeld, J. H.: Secondary organic aerosol formation from biomass burning intermediates: phenol and methoxyphenols, Atmos. Chem. Phys., 13, 8019–8043, https://doi.org/10.5194/acp-13-8019-2013, 2013.
Zetra, Y., B. Sosrowidjojo, I., and Perry Burhan, R. Y.: AROMATIC BIOMARKER FROM BROWN COAL, SANGATTA COALFIELD, EAST BORNEO OF MIDDLE MIOCENE TO LATE MIOCENE AGE, Jurnal Teknologi, 78, https://doi.org/10.11113/jt.v78.8822, https://journals.utm.my/jurnalteknologi/article/view/8822 (last access: 3 November 2023), 2016.
Zhang, J., Wang, X., Li, R., Dong, S., Chen, J., Zhang, Y., Zheng, P., Li, M., Chen, T., Liu, Y., Xue, L., Zhou, X., Du, L., Zhang, Q., and Wang, W.: Significant impacts of anthropogenic activities on monoterpene and oleic acid-derived particulate organic nitrates in the North China Plain, Atmos. Res., 256, 105585, https://doi.org/10.1016/j.atmosres.2021.105585, 2021.
Zhang, J., Li, K., Wang, T., Gammelsæter, E., Cheung, R. K. Y., Surdu, M., Bogler, S., Bhattu, D., Wang, D. S., Cui, T., Qi, L., Lamkaddam, H., El Haddad, I., Slowik, J. G., Prevot, A. S. H., and Bell, D. M.: Bulk and molecular-level composition of primary organic aerosol from wood, straw, cow dung, and plastic burning, Zenodo [data set], https://doi.org/10.5281/zenodo.10126682, 2023.
Zhou, H., Wu, C., Onwudili, J. A., Meng, A., Zhang, Y., and Williams, P. T.: Polycyclic aromatic hydrocarbons (PAH) formation from the pyrolysis of different municipal solid waste fractions, Waste Manag., 36, 136–146, 2015.