the Creative Commons Attribution 4.0 License.
the Creative Commons Attribution 4.0 License.
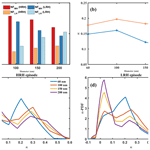
Measurement report: On the difference in aerosol hygroscopicity between high and low relative humidity conditions in the North China Plain
Jingnan Shi
Juan Hong
Nan Ma
Qingwei Luo
Yao He
Hanbing Xu
Haobo Tan
Qiaoqiao Wang
Jiangchuan Tao
Yaqing Zhou
Shuang Han
Long Peng
Linhong Xie
Guangsheng Zhou
Wanyun Xu
Yafang Cheng
Atmospheric processes, including both primary emissions and secondary formation, may exert complex effects on aerosol hygroscopicity, which is of significant importance in understanding and quantifying the effect of aerosols on climate and human health. In order to explore the influence of local emissions and secondary formation processes on aerosol hygroscopicity, we investigated the hygroscopic properties of submicron aerosol particles at a rural site in the North China Plain (NCP) in winter 2018. This was conducted by simultaneous measurements of aerosol hygroscopicity and chemical composition, using a custom-built hygroscopic tandem differential mobility analyzer (HTDMA) and a capture-vaporizer time-of-flight aerosol chemical speciation monitor (CV-ToF-ACSM). The hygroscopicity results showed that the particles during the entire campaign were mainly externally mixed, with a more hygroscopic (MH) mode and a less hygroscopic (LH) mode. The mean hygroscopicity parameter (κmean) values derived from hygroscopicity measurements for particles at 60, 100, 150, and 200 nm were 0.16, 0.18, 0.16, and 0.15, respectively. During this study, we classified two distinct episodes with different relative humidity (RH) and temperature (T) conditions, indicative of different primary emissions and secondary formation processes. It was observed that aerosols at all measured sizes were more hygroscopic under the high-RH (HRH) episode than those under the low-RH (LRH) episode. During the LRH, κ decreased with increasing particle size, which may be explained by the enhanced domestic heating at low temperature, causing large emissions of non-hygroscopic or less hygroscopic primary aerosols. This is particularly obvious for 200 nm particles, with a dominant number fraction (>50 %) of LH mode particles. Using O:C-dependent hygroscopic parameters of secondary organic compounds (κSOA), closure analysis between the HTDMA-measured κ and the ACSM-derived κ was carried out. The results showed that κSOA under the LRH episode was less sensitive to the changes in organic oxidation level, while κSOA under HRH had a relatively stronger dependency on the organic O:C ratio. This feature suggests that the different sources and aerosol evolution processes, partly resulting from the variation in atmospheric RH and T conditions, may lead to significant changes in aerosol chemical composition, which will further influence their corresponding physical properties.
- Article
(4459 KB) - Full-text XML
-
Supplement
(411 KB) - BibTeX
- EndNote
The hygroscopicity of aerosol, describing its tendency to absorb moisture from the environment, plays an important role in understanding and quantifying its effects on climate and human health (Martin et al., 2004; McFiggans et al., 2006; Swietlicki et al., 2008; Tao et al., 2012). Hygroscopic growth alters the global radiative balance directly by modifying the size distribution of aerosol particles and consequently influencing their light scattering and adsorption coefficients (Cheng et al., 2008; Sloane and Wolff, 1985). Hygroscopicity is also closely tied to the activation of particles to form cloud droplets and thus may influence the lifetime and microproperties of clouds, affecting the regional and global climate indirectly (Gunthe et al., 2009; Rose et al., 2010). Moreover, due to the absorbed water by aerosol particles, the hygroscopicity of particles is strongly associated with heterogeneous and multiphase chemistry, regulating the abundance of different species in the gas and particle phases (Hennigan et al., 2009; Ye et al., 2011). Furthermore, aerosol hygroscopicity can also influence human health by changing the deposition of aerosol particles in the respiratory system (Kreyling et al., 2006).
According to the Köhler theory, the propensity of aerosols to take up water at a certain subsaturation or supersaturation is mainly determined by the particle size and various properties of the species within the particles, such as solubility, molecular weight, and density, i.e., their chemical composition (Petters and Kreidenweis, 2008, 2007). Thus, ambient aerosols, owing to their different sources and atmospheric processes, may vary greatly in their chemical compositions and thus show significant differences in their hygroscopicity (Asmi et al., 2010; Cai et al., 2017; Ehn et al., 2007; Fan et al., 2020; Hong et al., 2015). Previous studies have shown that primary emitted aerosol, which normally contains substantial insoluble components, tends to have a lower hygroscopicity. Cai et al. (2017) measured the hygroscopicity at a suburban site in the Pearl River Delta (PRD) in China and a marine site in Okinawa, Japan. They found that the Okinawan aerosols were much more hygroscopic than those of PRD aerosols, which had a stronger influence from the traffic-related exhaust and other directly emitted pollutants. Fan et al. (2020) showed that the hygroscopicity of aerosol in Beijing was lower in winter than those in summer, owing to the larger contribution of less hygroscopic material from coal burning and other primary emissions in the wintertime of Beijing. There are also some other studies which focused on the secondarily formed or aged aerosols, being typically characterized as more hygroscopic. Asmi et al. (2010) measured the hygroscopicity of aerosols in the Antarctica region and concluded that the Antarctic aerosols with such a strong hygroscopicity were associated with the aging of secondary inorganic aerosols. Hong et al. (2015) reported that the aerosols at a boreal forest in southern Finland, typically representing the secondary biogenic sources, were quite hygroscopic, especially during the daytime when the photochemistry was stronger. All the above findings imply that, due to the different types of primary sources and complexity of secondary formation processes in different regions, the understanding of the connections between aerosol sources as well as its evolution processes and its hygroscopicity is still limited.
The North China Plain (NCP), one of the most populated and developed regions in China (Yang et al., 2018), has suffered from severe air pollution over the past decade (Yue et al., 2011; Zhang et al., 2016). Due to the intensive human activities, large amounts of primary air pollutants were emitted to the environment in this region, especially during wintertime (Du et al., 2018; Fan et al., 2020). Coupled with the unfavorable meteorological conditions in the wintertime of NCP, this further led to a fast production of secondary aerosols (Sun et al., 2013). Consequently, particle-phase chemical composition of this region showed distinct features, owing to the complex contribution from both primary emissions and secondary formation, which might result in obvious differences in aerosol hygroscopicity. Previous studies (Massling et al., 2009; Liu et al., 2014; Wu et al., 2016; Qi et al., 2018; Y. Wang et al., 2018a; Shen et al., 2021) have devoted extensive efforts to investigate the hygroscopicity of aerosols in the NCP. However, these studies mainly focused on the statistical analysis of the aerosol hygroscopicity in this region and tried to investigate its characteristics from the point of view of particle-phase chemical composition, but they seldom link to their sources or formation processes. Thus, the representative feature of how different sources, including both the primary emissions and secondary processes, impact the hygroscopicity of NCP aerosols still remains unclear.
In this study, we aim to explore the effects of different atmospheric processes on the aerosol hygroscopicity in the NCP. For this purpose, we measured the hygroscopicity of atmospheric aerosols at a rural measurement station in the NCP with a custom-built hygroscopic tandem differential mobility analyzer (HTDMA) system. We compared the characteristic hygroscopicity of ambient aerosols during different episodes in which aerosols were dominated by either primary sources or secondary formation, in order to reveal the impact of different atmospheric processes or sources on aerosol hygroscopicity.
2.1 Measurement site
Hygroscopicity measurements and chemical characterization of atmospheric aerosols were conducted at a rural measurement station (the Ecological and Environmental Monitoring Station of the Chinese Academy of Meteorological Sciences) in Gucheng, Dingxing County, Hebei Province (39.15∘ N, 115.74∘ E; 22 m a.s.l.) from 11 November to 14 December 2018. The observational site is surrounded by farmland and small residential towns, with a national highway about 1 km away to the west. The nearest large cities are Tianjin (110 km) and Beijing (100 km), two megacities in the NCP. Measurements at this site could well represent the regional conditions of the NCP. Detailed descriptions of the site can be found in Li et al. (2021). During the campaign, ambient meteorological conditions including air pressure, temperature, RH, wind speed, wind direction, and precipitation were measured continuously at the station. All the instruments for aerosol measurements were operated in a container, where temperature was maintained at 24 ∘C, and connected to a sampling inlet system using an isokinetic flow splitter. The sampling air was dried to RH below 20 % using a Nafion diffusion dryer.
2.2 Hygroscopicity measurements
In this study, the hygroscopic growth of atmospheric particles was measured with a custom-built HTDMA system. A detailed description of the HTDMA system can be found in previous studies (Hong et al., 2018; Tan et al., 2013). Briefly, ambient particles were dried to RH lower than 20 % by passing them through a Nafion dryer (model PD-70T-24 ss, Perma Pure Inc.). After charging to a known charge distribution through a Kr85 neutralizer (TSI, 3077), these particles were introduced into a differential mobility analyzer (DMA1, model 3081, TSI Inc.), where particles at a certain size were selected. Then these quasi-monodisperse particles were humidified to a specific RH (RH=90 % in this study) in a Nafion humidifier (Perma Pure Inc., PD-100T-24MSS). The residence time of the humidification unit is around 3 s. With a second DMA (DMA2, model 3081 L, TSI Inc.) and a condensation particle counter (CPC, model 3772, TSI Inc.), operated at a flow rate of 1 L min−1, the number size distribution of the humidified aerosols was measured. The hygroscopic growth factor (HGF) was then obtained, which is defined as (Eq. 1) the ratio of the particle diameter at a given RH (D(RH), RH=90 %) to the dry diameter (D0):
In this study, particles of four different mobility diameters (D0=60, 100, 150, and 200 nm) were measured with a time resolution of 20 min. Raw data were inverted by the TDMAinv algorithm designed by Gysel (2009). Polystyrene latex (PSL) spheres (Thermo Scientific, Duke Standards) were used to verify the sizing accuracy of both DMAs in the system. Calibration measurements in terms of the accuracy and stability of RH inside the HTDMA were performed using ammonium sulfate particles, of which the deliquescence RH and hygroscopic growth factor at different RH values are well known. Calibration measurements using ammonium sulfate were conducted once a week.
2.3 Chemical composition measurements
An Aerodyne capture-vaporizer time-of-flight aerosol chemical speciation monitor (CV-ToF-ACSM, ACSM hereafter) was used to measure the non-refractory PM1 chemical composition, including , , , Cl−, and organics at a time resolution of 2 min (Zheng et al., 2020). The instrument was operated at a flow rate of 0.1 L min−1 with a 100 % collection efficiency during the sampling period. The oxidation level (O:C ratio) of the organic fractions was estimated by the mass fractions of 44 (f44) in total organics according to Canagaratna et al. (2015). In order to further characterize the origin and evolution of the organic fractions in aerosols (Ng et al., 2010), subsequent analyses of OA mass spectra obtained from ACSM measurements were deployed using positive matrix factor (PMF) (Paatero and Tapper, 1994; Ulbrich et al., 2009). Several OA factors corresponding to different sources and processes, including hydrocarbon-like OA (HOA), cooking OA (COA), coal combustion OA (CCOA), biomass-burning OA (BBOA), and oxygenated OA (OOA) were identified. In particular, HOA, COA, CCOA, and BBOA were typically considered primary factors (POA) and OOA as secondary factor (SOA). Detailed descriptions of the measurements and PMF analysis can be found in Sun et al. (2018).
The mass concentrations of elemental carbon (EC) and organic carbon (OC) were analyzed using an OC/EC aerosol analyzer (Sunset Laboratory, Forest Grove, OR, USA) (Bae et al., 2004) through a PM10 inlet. A detailed description of the instrument can be found in Jeong et al. (2004). The EC (hereafter BC in our study) mass concentration in PM1 was determined from the measured EC in PM10 using a correction factor according to Chen et al. (2019).
3.1 Hygroscopicity parameter
According to the κ-Köhler theory (Petters and Kreidenweis, 2007), the hygroscopic parameter (κ) describes the water uptake ability of particles, which can be calculated at any given RH value or supersaturation. From the HTDMA measurements, κ can be derived using the following equations:
where HGF is the hygroscopic growth factor measured by our HTDMA at a given RH (e.g., 90 % in our study), D0 is the size of particles selected by the first DMA, σs/a is the droplet surface tension and was assumed to be the surface tension of pure water (σs/a=0.0728 N m−2), Mw is the molecular weight of water, R is the universal gas constant, T is the absolute temperature, and ρw is the density of water. According to the derived κ distribution, we defined two hygroscopic groups for the ambient particles measured in our study: κ<0.1 as particles of the less hygroscopic (LH) mode and κ>0.1 as the more hygroscopic (MH) particles (Liu et al., 2011).
3.2 Hygroscopicity chemical composition closure
For ambient mixed particles, κ can also be predicted by the Zdanovskii–Stokes–Robinson (ZSR) mixing rule assuming volume additivity. Specifically, κ of a mixed particle (κmix) (Eq. 4) can be calculated by using the hygroscopicity parameter of the components in the mixture and their respective volume fractions (Petters and Kreidenweis, 2007),
where κi is the hygroscopicity parameter of a component i, and εi is the volume fraction of the component i in the mixed particles which can be derived using particle chemical composition data measured by ACSM and OC/EC. The same approach to calculate εi based on particle chemical composition was described in previous studies with more details (Hong et al., 2014, 2018). The volume fractions of six neutral components, including (NH4)2SO4, NH4HSO4, NH4NO3, H2SO4, organics, and BC were ultimately determined and their corresponding κ measured by previous work (Cross et al., 2007; Gysel et al., 2007; Wu et al., 2016) were summarized in Table 1. Note that the hygroscopicity parameter for organic species (κorg) was not well characterized in contrast to inorganic salts. Alternatively, κorg was typically assumed to be constant, ranging from 0.1 to 0.4, or varied as a function of their oxidation level when predicting κmix in previous work (Asmi et al., 2010; Chang et al., 2010; Gunthe et al., 2009). In our case, a distinct approach to approximate the hygroscopicity of organics (κorg), particularly for that of SOA (κSOA), was introduced, based on the aforementioned material and method. A detailed description on the approximation of κorg will be given in Sect. 4.4; therefore, κorg in Table 1 was not specified.
4.1 Overview of the measurement
Figure 1 displays the time series of meteorological conditions (e.g., the average wind speed, wind direction, temperature, and relative humidity); mass concentration of PM2.5 and BC; the averaged hygroscopicity parameter (κ); κ probability density function (κ-PDF) for particles at dry sizes of 60, 100, 150, and 200 nm; and the mass fractions of chemical components in PM1 measured by ACSM. In general, the wind speed was typically quite low during the nighttime compared to that during daytime, leading to a limited dilution of the air pollutants in the rural atmosphere. Thus, a similar diurnal pattern was also observed for BC (ranging from 0 to 30 µg m−3) and PM2.5 (ranging from 1.72 to 245.14 µg m−3) mass concentration. The ambient temperature (T) increased during the daytime with a relatively low RH and dropped dramatically during the nighttime, leading to a significant enhancement of ambient RH. Clearly, we observed two different episodes with a distinguished difference in ambient RH and T, during which the contribution of primary aerosols and secondary aerosol formation processes might vary significantly. Thus, two distinct episodes (e.g., “high RH (HRH) episode” on 19–27 November and “low RH (LRH) episode” on 7–14 December) were characterized. To be specific, the average RH during the HRH episode was 71±22 %, with an average temperature of 3 ∘C, while during the LRH episode the average RH was 43±17 %, with an average temperature of −6 ∘C. Separate analysis of the hygroscopicity and chemical composition of aerosols for these two episodes will be further discussed in Sect. 4.2–4.4.
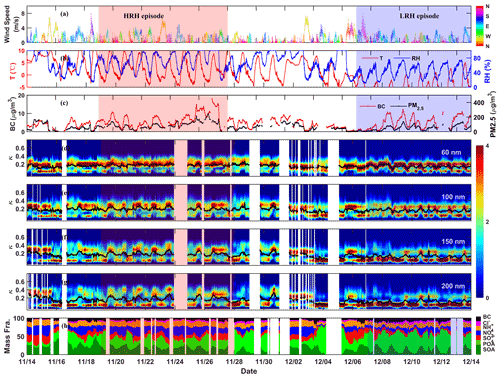
Figure 1Time series of (a) wind speed and direction; (b) temperature and relative humidity; (c) PM2.5 and BC mass concentrations; (d–g) the hygroscopicity parameter (κ) probability density function (κ-PDF) for particles at dry sizes of 60, 100, 150, and 200 nm (the black line is the averaged hygroscopicity parameter κ for particles at each size); and (h) mass fractions of the PM1 chemical components during this field campaign.
The averaged hygroscopicity parameter κ for particles at each size (black lines in Fig. 1d–g) shows a clear temporal variation, which is similar to the mass fraction of chemical composition in PM1. Two distinct modes with κ<0.1 as the less hygroscopic (LH) mode and κ>0.1 as the more hygroscopic (MH) mode were mostly observed for all sized particles from the κ-PDF, indicating that the particles were mainly externally mixed during our measurements. This was further confirmed by the clear bimodal distribution from the averaged κ-PDF in Fig. 2. Both the averaged and the time series data of κ-PDF show a strong size dependency. For instance, the number fraction of 60 nm particles was dominated by the MH mode during most of time. However, with increasing particle size, the LH mode became more prominent. This is particularly obvious for 200 nm particles with a clear dominance of LH particles, especially during the LRH episode.
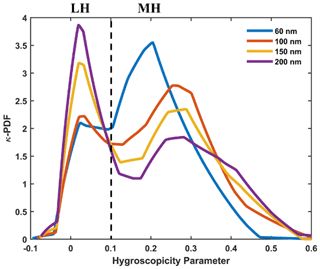
Figure 2The average hygroscopicity parameter probability density functions (κ-PDF) for 60, 100, 150, and 200 nm sized particles over the entire campaign. Two hygroscopic modes of the particles are defined: κ<0.1 as the less hygroscopic (LH) mode and κ>0.1 as the more hygroscopic (MH) mode.
During our study, the ensemble mean hygroscopicity parameter (κmean) values were 0.16, 0.18, 0.16, and 0.15 for 60, 100, 150, and 200 nm particles, respectively, as shown in Fig. 3. The results in our study show that the aerosols at the current station have the lowest hygroscopicity compared with aerosols in other cities or regions in China. This is more likely due to the largest contribution of organics relative to inorganic species in PM1 at our observational site. Slightly higher hygroscopicity was observed for the aerosols in other regions of the NCP, e.g., the urban aerosols in Beijing (Y. Wang et al., 2018b), suggesting similar primary emissions or secondary aerosol formation processes in the NCP. Moreover, a clear increasing trend in κmean with increasing particle size was observed in most of the previous studies in China (Jiang et al., 2016; Wu et al., 2013; Ye et al., 2011; Yuan et al., 2020). However, on the contrary, our particles at larger sizes were less hygroscopic than smaller ones. This phenomenon was also observed for the aerosols in the wintertime of Beijing in 2005 and 2015 (Massling et al., 2009; Y. Wang et al., 2018b). A detailed explanation with respect to the lower hygroscopicity at larger size will be given in the following section.
4.2 Different aerosol hygroscopicity under the HRH and LRH episodes
In order to explore the effects of different atmospheric emissions or processes on the aerosol hygroscopicity in the NCP, we analyzed the hygroscopicity and mixing state of particles at different sizes during the two distinguished episodes. Figure 4 shows the size-dependent number fractions of LH mode (NFLH) and MH mode (NFMH) particles under these two distinct episodes. It can be seen that under the HRH episode, NFLH increased slightly with increasing particle size (e.g., from 20 % for 60 nm particles to 30 % for 200 nm particles), with MH mode still being the dominant one. Under the LRH episode, however, a substantial increase in NFLH with particle size (e.g., from 28 % to 53 %) was observed with an obvious decrease in NFMH in large particles accordingly. As a result, the average κ values for each size, indicated in Fig. 4b, were generally lower under the LRH episode than those under the HRH episode. Moreover, under the LRH episode, an obvious lower κ was observed for larger particles (e.g., 200 nm particles) compared to that of smaller ones, which is probably due to the higher content of LH mode particles in aerosols (see Fig. 4d).
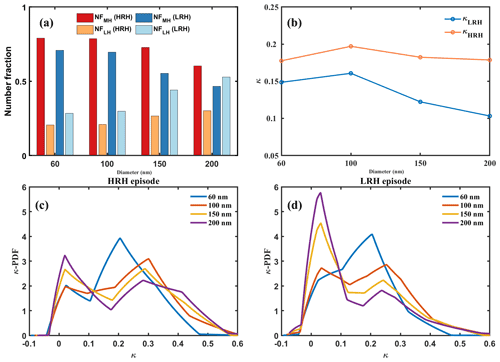
Figure 4Number fractions of the MH mode and LH mode in particles at 60, 100, 150, and 200 nm and the average κ value of particles at each size under the HRH and LRH episodes.
The clear variability in aerosol hygroscopicity between these two episodes is not surprising as at our observational site organics were the dominant species in PM1 under the LRH episode, while inorganic species dominated the PM1 under the HRH, as shown in Fig. 5. It has to be noted that the submicrometer mass concentration illustrated in Fig. 5 represented the PM1 bulk chemical composition, which was normally dominated by particles at the size near the mode size in the mass size distribution. Therefore, we further analyzed our particle number size distribution (7–9701 nm) data and found that the mode size of the mass size distribution of aerosols during our experimental campaign was around 390 nm, assuming a constant particle density of 1600 kg m−3. Based on this result, we considered that the bulk chemical composition measured by our ACSM could nearly reflect the ones for particles near the mode size, e.g., 200–300 nm.
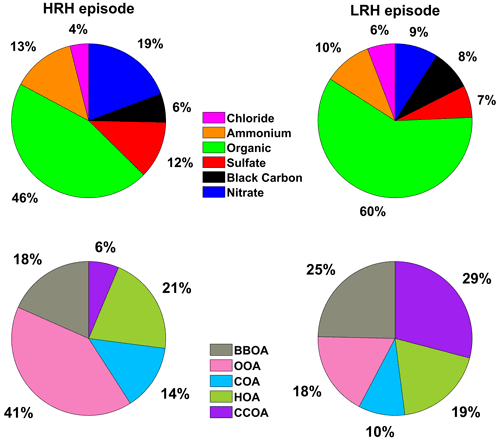
Figure 5A comparison of the PM1 chemical composition during the HRH and LRH episodes. (BBOA: biomass-burning organic aerosols, OOA: oxygenated organic aerosols, COA: cooking organic aerosols, HOA: hydrocarbon organic aerosols, CCOA: coal combustion organic aerosols.)
Back to the discussion on the particle chemical composition, the different contents of organic and inorganic species in particles reveal distinct sources or formation mechanisms of these components under different episodes. This in turn influences the aerosol hygroscopicity, as aerosols with more organics that typically have a lower κ than inorganic species will be less hygroscopic than those having more inorganics. Inorganic species, i.e., sulfate, nitrate, and ammonium, are usually considered to be mainly from secondary formations (Sun et al., 2015). In our study, inorganic species contributed 44 % to the PM1 mass in the HRH episode, which were higher than those under the LRH episode. This was especially obvious for nitrate, which accounted for 19 % of mass in PM1 under the HRH but only 9 % under the LRH. Previous studies (Li et al., 2018; Liu et al., 2020; H. Wang et al., 2018) have found that ambient conditions with substantial nitrate in ambient aerosols were typically associated with elevated ambient RH conditions, which would lead to a higher aerosol liquid water content. This would in turn promote the reactive uptake of precursors and thermodynamic equilibrium of ammonium nitrate and thus result in an increased particulate nitrate formation (Tong et al., 2021; Wang et al., 2020). These findings may be responsible for the significant increase in nitrate content in aerosols during the HRH episode in our study. As nitrate is quite hygroscopic, this feature may be one of the most important reasons for the obvious difference in aerosol hygroscopicity between these two episodes, at least for 200 nm particles.
Organic fraction, which is another major component in aerosols, also varies significantly in hygroscopicity due to the existence of numerous and highly diverse organic compounds. Based on this, we grouped them into several individual factors according to their potentially similar sources under these two different episodes, as illustrated in the bottom panel of Fig. 5. We can see that the mass fraction of POA in the total organics during the HRH episode was 23 % lower than that during the LRH episode. In particular, CCOA, which is the main component of POA, accounted only for 6 % of the total organics under the HRH episode but as much as 29 % under the LRH. Considering the distinct content of total organics between the two episodes, the difference in the mass fraction of CCOA in PM1 would be even more significant, being 2.8 % (46 %×6 %) for the HRH and 17 % (60 %×29 %) for the LRH. The high level of CCOA in aerosols could be explained by the fact that during the LRH episode wintertime residential heating was initiated, during which a significant amount of its pollutants, e.g., CCOA, was emitted to the atmosphere (Hua et al., 2018). Moreover, we observed that the mass fraction of OOA in PM1 during the HRH episode was 19 % (46 %×41 %), being different from that (11 %, e.g., 60 %×18 %) during the LRH, though only slightly higher. Thereby, the varied level of different organics in PM1 could be one of the plausible reasons for the different κ values in 200 nm between these two episodes.
Moreover, we observed that both the mass fraction and the absolute mass concentration of OOA during the HRH episode was higher than that during the LRH. Kuang et al. (2020) specifically studied the formation mechanism of secondary organic aerosols for the current campaign. They found that the daytime OOA formation rates correlated quite well with nitrate formation rates and thus suggested that they probably shared similar formation pathways. They further investigated the formation pathways of nitrate and concluded that during the low RH conditions nitrate was mainly formed by the gas-phase oxidation of NO2, while at the high RH conditions both the aqueous-phase processes and gas-phase oxidation dominated its formation. Furthermore, they considered that gas-phase formation of OOA would mainly add mass to the condensation mode of aerosol size distribution, while aqueous-phase formation of OOA may elevate the mass in the droplet mode. After studying the diurnal evolution of aerosol mass distribution for high RH and low RH conditions, together with the indirect evidence from nitrate formation, they concluded that gas-phase formation contributed dominantly to the OOA under the low RH conditions, while at high RH conditions, when aerosol water content was high, aqueous-phase photo-oxidation were mainly responsible for the rapid OOA formation, besides the gas-phase formation. According to their further comparison with laboratory experiments (Sun et al., 2010; Yu et al., 2014), they suggested that the OOA formed through aqueous-phase reactions that were normally more oxidized compared to those formed by gaseous processes. It needs to be specified that the average RH values for the HRH and LRH episodes during our campaign were 71 % and 43 %, respectively, being quite close to the ones (53 % and 38 %) for the two defined RH conditions in Kuang et al. (2020). Taken all together, it is reasonable to presume that the observed lower κ values for 200 nm particles under the LRH episode are due to not only the elevated POA fraction in aerosols but also a different hygroscopic nature of OOA compared to that under HRH. All these above features with respect to both inorganic and organic fractions demonstrate that the complexity in primary and secondary aerosol formation processes under these two distinct episodes led to a particular variability in aerosol chemical composition, which further resulted in different characteristics in aerosol hygroscopicity.
4.3 Diurnal variation
To better understand the influence of human activities and secondary formation on the aerosol hygroscopicity of current study on a daily scale, we compared the diurnal variation of the number fractions and individual κ of LH and MH mode particles under these two episodes, as shown in Fig. 6. Under the HRH episode, particles at all four sizes (60, 100, 150, 200 nm) were dominated by the MH mode, which has been already discussed in Sect. 4.2. The general pattern for the number fraction of LH mode particles under the HRH episode did not vary significantly among particles of different sizes, with a sharp decrease after 07:00 LT in the morning and a slow increase around noon till 20:00 in the evening. Similar trends were also observed for particles at 100, 150, and 200 nm under the LRH episode except 60 nm particles. Concurrently, the obtained κ of LH mode particles at all four sizes, no matter which episode was considered, increased slightly in the morning, reached the peak around noon, and decreased back until the evening.
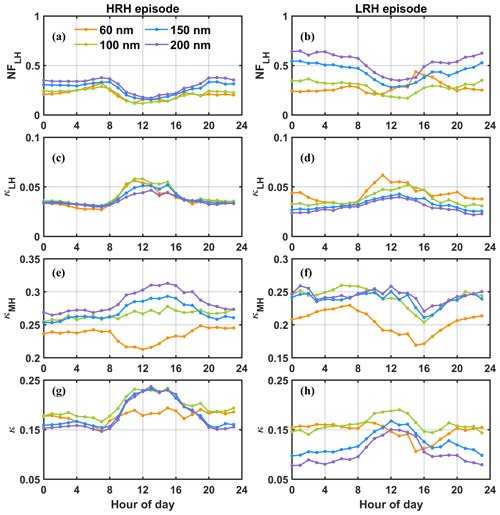
Figure 6Diurnal variation of the number fractions and individual κ of LH and MH mode particles under these two episodes.
The higher hygroscopicity of LH mode particles and lower degree of external mixing during daytime (except for the 60 nm particles under the LRH episode) could be explained by the fact that these LH mode particles started to age in the atmosphere during the daytime either by photochemistry processes or multiphase reactions. These aged particles became more hygroscopic; thus, the increases in κ were observed in the daytime for LH particles. However, a part of these aged LH particles became much more hygroscopic, with the obtained κ beyond the range for LH mode but reached that of MH mode particles. Therefore, these particles were classified into MH fractions and thus a lower number fraction of LH particles was observed.
The elevated hygroscopicity during daytime for MH mode particles was also observed, especially apparent during the HRH episode, except for that of 60 nm particles, as illustrated in Fig. 6e. During daytime when solar radiation is stronger, higher concentration of atmospheric oxidant was typically observed (Hong et al., 2015). Thus, the associated photochemistry and other aging processes would be more favorable, leading to a more oxidized material condensing or partition into the existing aerosols and thus an elevated aerosol hygroscopicity being expected.
Particularly for 60 nm particles, both the number fraction of LH mode particles under the LRH episode and the hygroscopicity of MH mode under HRH show a different diurnal trend compared to that of particles at the other three sizes. Higher fractions of their LH mode particles were observed in the afternoon, peaking around 15:00. This indicates a different source for the LH group of Aitken mode particles, e.g., residential heating as well as local traffic emissions. The diurnal cycles of the POA mass fraction in Fig. 7 were consistent with those for the number fractions of LH mode particles at larger sizes. As POA is non-hygroscopic or less hygroscopic, this further suggests that the likely candidate for these LH mode particles could be soot or water-insoluble organics, e.g., similar component as POA. On the other hand, the hygroscopicity of MH mode particles decreased in the daytime under the HRH episode, opposite to the other sizes. The reason for this pattern is not quite clear and indicates a very different atmospheric process of these Aitken mode particles, which should be analyzed further in future work.
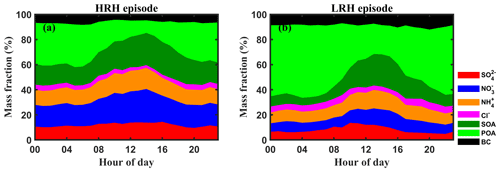
Figure 7Diurnal variation of the mass fraction of , , Cl−, , BC, POA, and SOA in particle phase during the HRH and LRH episodes.
The daily variations of all the factors, including the relative number fraction of LH or MH mode particles and their corresponding hygroscopicity, contribute together to the diurnal pattern of the overall ensemble mean κ for particles at each size, with generally higher values under HRH compared to that under the LRH episode (see Fig. 6g–h). This pattern is reasonable as there were more inorganic species as well as OOA in particles under the HRH episode (Fig. 7); their observed mean κ, at least for larger particles, was supposed to be larger compared to that under LRH.
4.4 Relating the SOA hygroscopicity to its oxidation level for the two episodes
As introduced in Sect. 3.2, the hygroscopicity of particles can also be derived on the basis of chemical composition measurements according to the ZSR mixing rule. The κorg is the biggest unknown in the composition-based derivations and the different choices of κorg could lead to large deviations in the hygroscopicity for the studied mixtures. This is particularly difficult for secondary organics, as POA was normally considered to be non-hygroscopic. However, coupling HTDMA-measured κ with particle-phase chemical composition data, we may be able to approximate the hygroscopicity for organics, especially the secondary organics. Specifically, κSOA can be calculated by subtracting κ of inorganic species, POA, and BC from HTDMA-measured κ (Eq. 5):
where κInorg represents κ of all the inorganic species, POA and BC are measured by ACSM and assuming the ZSR mixing rule, and εi is the volume fraction of different components. As POA and BC are not hygroscopic, values for κPOA and κBC of 0 were assumed. This approach for the approximation of κSOA has also been applied in previous studies (Wu et al., 2016, 2013). As the bulk chemical composition measured by ACSM may deviate significantly from that of size-resolved ones, we plotted the particle mass distribution of aerosols averaged over the entire campaign (see Fig. S1 in the Supplement). From Fig. S1, we found that the mode size of the mass size distribution of aerosols during our experimental campaign was around 390 nm. Thereby, we considered that the bulk chemical composition measured by our ACSM could nearly reflect or at least be close to that of 200 nm particles.
Previous studies suggested that the hygroscopicity of organic components varies linearly with their oxidation level (O:C). In order to investigate the different characteristics of secondary organic aerosols during these two distinct episodes, we separately examined the relationship between the organic hygroscopicity (κSOA) and their oxidation level. Figure 8 shows the positive correlations between κSOA and O:C ratios for organics during both episodes but with different O:C dependencies. It is important to note that even though we considered that the chemical composition of 200 nm particles was close to that of PM1 measured by ACSM, there still potentially remained deviations between size-resolved chemical composition and bulk ones (Xu et al., 2021). Therefore, current correlations were only used for characteristic comparison of organic hygroscopicity between these two episodes. Parameterization using these correlations for organic aerosols at the current site from a broader perspective, e.g., for climate modelers, is not suggested.
Specifically, under the HRH episode, the O:C ratio of organic components ranged between 0.30 and 1.29 with an average value of 0.76. It was noted that due to the availability of hygroscopicity data, not all of the measured O:C values were shown, and a smaller data coverage is displayed in Fig. 8. The hygroscopicity of secondary organic aerosols during this episode shows a relatively stronger O:C dependency as . Considering this relation, the average O:C ratio of this episode corresponds to a mean κSOA of 0.17, which is moderately hygroscopic. This feature is similar to the suburban organic aerosols in Guangzhou measured by Hong et al. (2018). The average RH during their study in Guangzhou was 72 %, being also quite high and thus suggesting a potentially similar formation mechanism for secondary organic aerosols, e.g., aqueous-phase photochemistry as speculated previously.
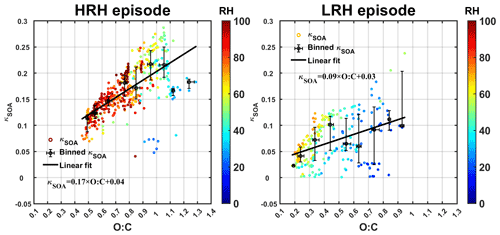
Figure 8The plots of κSOA vs. O:C ratios during the HRH and LRH episodes. The black line is the fitting to the measured data. The black point is the data that κSOA values were binned by O:C with an increment of 0.1. The color bars indicate RH.
In contrast, the hygroscopicity of SOA during the LRH episode shows a weak O:C dependency as . Considering that the average O:C ratio was 0.52 over the entire LRH episode, spreading from 0.18 to 1.24, the average κSOA of the LRH organics was approximately 0.08, which was much lower than that under HRH. Similarly, Wu et al. (2016), performed in the urban area of the NCP, showed marginal increase in hygroscopicity with the O:C ratio, approximate to the ones for our LRH aerosols. We found that during the study by Wu et al. (2016), ambient RH was 40 % on average. Thereby, we speculate that the similar characteristics in hygroscopicity of secondary organic aerosols in the NCP of both studies were likely due to the comparable low RH conditions, indicative of similar formation pathways. Moreover, the difference in κSOA under these two episodes is physically reasonable under the HRH episode, as previously suggested, and the rapid OOA formation were mainly produced through aqueous-phase photo-oxidation, which tends to form more oxidized organics. On the contrary, relatively less oxidized organics, which were less hygroscopic, were mostly formed through gas-phase reactions under the LRH episode.
As the ambient RH had a large diurnal variation during our campaign, which implies that low or high RH conditions may also occur during any individual day of the HRH or LRH episodes, we further grouped the data points of Fig. 8 into two categories according to their absolute RH values for these two episodes, as shown in Fig. 9. The threshold at RH of 60 % was set for these two categories due to the RH intensity spread in Fig. 8. At conditions of RH larger than 60 %, the hygroscopicity of SOA under the HRH and LRH episodes both show a strong O:C dependency, with the fitting under LRH being more skewed. At RH values lower than 60 %, the relationship between κSOA and the O:C for these two episodes became even closer. However, we observed that the absolute value of κSOA still varied between these two episodes, even at similar RH ranges, though their individual behavior towards the variation of O:C was similar. This implies that there still remain differences in these SOAs at different episodes but similar RH conditions, e.g., their chemical composition, indicating that the formation pathways of these SOA or the relevant reaction precursors might still be different under these two episodes. Thus, separation of these two episodes as previously defined was kept as the main conclusion, and distinct groups with respect to their RH will not be merged further.
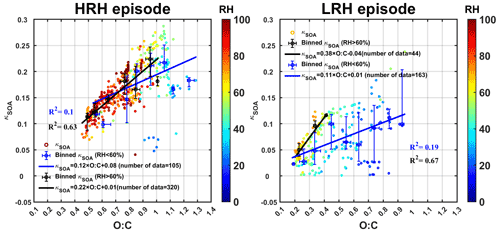
Figure 9The plots of κSOA vs. O:C ratios during the HRH and LRH episodes. The black line and blue line are the fitting to the measured data at RH<60 % and RH>60 %, respectively. The blue and black points show that κSOA values were usually binned by O:C with an increment of 0.1. The color bars indicate RH.
Simultaneous measurements of hygroscopicity and chemical fractions were performed at a rural observational site of the NCP during the winter of 2018. The hygroscopic probability density function (κ-PDF) distribution indicates that aerosols in this region were usually externally mixed. Particle hygroscopicity decreased with increasing aerosol size, with κmean values of 0.16, 0.18, 0.16, and 0.15 for 60, 100, 150, and 200 nm particles, respectively. Different RH and T conditions, indicating distinctive primary emissions and secondary aerosol formation processes, were distinguished, namely, the HRH episodes and the LRH episodes, respectively. The mean κ value of particles at each size was generally lower under the LRH episode than that under the HRH episode. During the HRH episode, NFLH increased slightly with increasing particle size, while under the LRH episodes this trend was opposite with the LH mode particles being dominant for 200 nm particles. The different contributions of less hygroscopic material from primary emissions and the complexity in the secondary aerosol formation, such as the formation of nitrate and OOA, were the main reasons for the distinct hygroscopicity of aerosols between the two episodes.
The HTDMA-measured κ values were compared against the ones predicted by ACSM to obtain a relationship between the κSOA and their oxidation level (O:C). The results show that κSOA under LRH conditions was less sensitive to the changes in organic oxidation levels, while κSOA under HRH conditions had a stronger O:C dependency. Based on these findings, we concluded that intricate atmospheric processes or emission sources could exert a significant influence on the chemical composition of atmospheric aerosols, leading to synthetic variation of aerosol hygroscopicity. The variability of aerosol hygroscopicity, on the other hand, may in turn benefit our understanding of the formation and evolution of complex atmospheric processes.
The detailed data can be obtained from https://doi.org/10.5281/zenodo.5758744 (Shi, 2021).
The supplement related to this article is available online at: https://doi.org/10.5194/acp-22-4599-2022-supplement.
JS analyzed the data, plotted the figures, and wrote the original draft. JH planned the study, collected the resources, and wrote and finalized the article. NM collected the resources and reviewed and finalized the article. HX contributed to the instrument maintenance. HT, QL, YH, JT, YZ, LP, LX, GZ, WX, YS, and SH discussed the results. QW, YC ,and HS contributed to fund acquisition.
At least one of the (co-)authors is a member of the editorial board of Atmospheric Chemistry and Physics. The peer-review process was guided by an independent editor, and the authors also have no other competing interests to declare.
Publisher's note: Copernicus Publications remains neutral with regard to jurisdictional claims in published maps and institutional affiliations.
The authors acknowledge financial support from the National Natural Science Foundation of China, Special Fund Project for Science and Technology Innovation Strategy of Guangdong Province, and Guangdong Innovative and Entrepreneurial Research Team Program.
This research has been supported by the National Natural Science Foundation of China (grant nos. 41705099 and 91644218), the National Key Research and Development Program of China (grant no. 2017YFC0210104), and the Guangdong Innovative and Entrepreneurial Research Team Program (grant no. 2016ZT06N263).
This paper was edited by Markus Petters and reviewed by two anonymous referees.
Asmi, E., Frey, A., Virkkula, A., Ehn, M., Manninen, H. E., Timonen, H., Tolonen-Kivimäki, O., Aurela, M., Hillamo, R., and Kulmala, M.: Hygroscopicity and chemical composition of Antarctic sub-micrometre aerosol particles and observations of new particle formation, Atmos. Chem. Phys., 10, 4253–4271, https://doi.org/10.5194/acp-10-4253-2010, 2010.
Bae, M. S., Schauer, J. J., DeMinter, J. T., Turner, J. R., Smith, D., and Cary, R. A.: Validation of a semi-continuous instrument for elemental carbon and organic carbon using a thermal-optical method, Atmos. Environ., 38, 2885–2893, https://doi.org/10.1016/j.atmosenv.2004.02.027, 2004.
Cai, M., Tan, H., Chan, C. K., Mochida, M., Hatakeyama, S., Kondo, Y., Schurman, M. I., Xu, H., Li, F., Shimada, K., Li, L., Deng, Y., Yai, H., Matsuki, A., Qin, Y., and Zhao, J.: Comparison of aerosol hygroscopcity, volatility, and chemical composition between a suburban site in the Pearl River Delta region and a marine site in Okinawa, Aerosol Air Qual. Res., 17, 3194–3208, https://doi.org/10.4209/aaqr.2017.01.0020, 2017.
Canagaratna, M. R., Jimenez, J. L., Kroll, J. H., Chen, Q., Kessler, S. H., Massoli, P., Hildebrandt Ruiz, L., Fortner, E., Williams, L. R., Wilson, K. R., Surratt, J. D., Donahue, N. M., Jayne, J. T., and Worsnop, D. R.: Elemental ratio measurements of organic compounds using aerosol mass spectrometry: characterization, improved calibration, and implications, Atmos. Chem. Phys., 15, 253–272, https://doi.org/10.5194/acp-15-253-2015, 2015.
Chang, R. Y.-W., Slowik, J. G., Shantz, N. C., Vlasenko, A., Liggio, J., Sjostedt, S. J., Leaitch, W. R., and Abbatt, J. P. D.: The hygroscopicity parameter (κ) of ambient organic aerosol at a field site subject to biogenic and anthropogenic influences: relationship to degree of aerosol oxidation, Atmos. Chem. Phys., 10, 5047–5064, https://doi.org/10.5194/acp-10-5047-2010, 2010.
Chen, W., Tian, H., and Qin, K.: Black carbon aerosol in the industrial city of xuzhou, china: Temporal characteristics and source appointment, Aerosol Air Qual. Res., 19, 794–811, https://doi.org/10.4209/aaqr.2018.07.0245, 2019.
Cheng, Y. F., Wiedensohler, A., Eichler, H., Heintzenberg, J., Tesche, M., Ansmann, A., Wendisch, M., Su, H., Althausen, D., Herrmann, H., Gnauk, T., Brüggemann, E., Hu, M., and Zhang, Y. H.: Relative humidity dependence of aerosol optical properties and direct radiative forcing in the surface boundary layer at Xinken in Pearl River Delta of China: An observation based numerical study, Atmos. Environ., 42, 6373–6397, https://doi.org/10.1016/j.atmosenv.2008.04.009, 2008.
Cross, E. S., Slowik, J. G., Davidovits, P., Allan, J. D., Worsnop, D. R., Jayne, J. T., Lewis, D. K., Canagaratna, M., and Onasch, T. B.: Laboratory and ambient particle density determinations using light scattering in conjunction with aerosol mass spectrometry, Aerosol Sci. Technol., 41, 343–359, https://doi.org/10.1080/02786820701199736, 2007.
Du, P., Gui, H., Zhang, J., Liu, J., Yu, T., Wang, J., Cheng, Y., and Shi, Z.: Number size distribution of atmospheric particles in a suburban Beijing in the summer and winter of 2015, Atmos. Environ., 186, 32–44, https://doi.org/10.1016/j.atmosenv.2018.05.023, 2018.
Ehn, M., Petäjä, T., Aufmhoff, H., Aalto, P., Hämeri, K., Arnold, F., Laaksonen, A., and Kulmala, M.: Hygroscopic properties of ultrafine aerosol particles in the boreal forest: diurnal variation, solubility and the influence of sulfuric acid, Atmos. Chem. Phys., 7, 211–222, https://doi.org/10.5194/acp-7-211-2007, 2007.
Fan, X., Liu, J., Zhang, F., Chen, L., Collins, D., Xu, W., Jin, X., Ren, J., Wang, Y., Wu, H., Li, S., Sun, Y., and Li, Z.: Contrasting size-resolved hygroscopicity of fine particles derived by HTDMA and HR-ToF-AMS measurements between summer and winter in Beijing: the impacts of aerosol aging and local emissions, Atmos. Chem. Phys., 20, 915–929, https://doi.org/10.5194/acp-20-915-2020, 2020.
Gunthe, S. S., King, S. M., Rose, D., Chen, Q., Roldin, P., Farmer, D. K., Jimenez, J. L., Artaxo, P., Andreae, M. O., Martin, S. T., and Pöschl, U.: Cloud condensation nuclei in pristine tropical rainforest air of Amazonia: size-resolved measurements and modeling of atmospheric aerosol composition and CCN activity, Atmos. Chem. Phys., 9, 7551–7575, https://doi.org/10.5194/acp-9-7551-2009, 2009.
Gysel, M., Crosier, J., Topping, D. O., Whitehead, J. D., Bower, K. N., Cubison, M. J., Williams, P. I., Flynn, M. J., McFiggans, G. B., and Coe, H.: Closure study between chemical composition and hygroscopic growth of aerosol particles during TORCH2, Atmos. Chem. Phys., 7, 6131–6144, https://doi.org/10.5194/acp-7-6131-2007, 2007.
Gysel, M., McFiggans, G. B., and Coe, H.: Inversion of tandem differential mobility analyser (TDMA) measurements, J. Aerosol Sci., 40, 134–151, https://doi.org/10.1016/j.jaerosci.2008.07.013, 2009.
Hennigan, C. J., Bergin, M. H., Russell, A. G., Nenes, A., and Weber, R. J.: Gas/particle partitioning of water-soluble organic aerosol in Atlanta, Atmos. Chem. Phys., 9, 3613–3628, https://doi.org/10.5194/acp-9-3613-2009, 2009.
Hong, J., Häkkinen, S. A. K., Paramonov, M., Äijälä, M., Hakala, J., Nieminen, T., Mikkilä, J., Prisle, N. L., Kulmala, M., Riipinen, I., Bilde, M., Kerminen, V.-M., and Petäjä, T.: Hygroscopicity, CCN and volatility properties of submicron atmospheric aerosol in a boreal forest environment during the summer of 2010, Atmos. Chem. Phys., 14, 4733–4748, https://doi.org/10.5194/acp-14-4733-2014, 2014.
Hong, J., Kim, J., Nieminen, T., Duplissy, J., Ehn, M., Äijälä, M., Hao, L. Q., Nie, W., Sarnela, N., Prisle, N. L., Kulmala, M., Virtanen, A., Petäjä, T., and Kerminen, V.-M.: Relating the hygroscopic properties of submicron aerosol to both gas- and particle-phase chemical composition in a boreal forest environment, Atmos. Chem. Phys., 15, 11999–12009, https://doi.org/10.5194/acp-15-11999-2015, 2015.
Hong, J., Xu, H., Tan, H., Yin, C., Hao, L., Li, F., Cai, M., Deng, X., Wang, N., Su, H., Cheng, Y., Wang, L., Petäjä, T., and Kerminen, V.-M.: Mixing state and particle hygroscopicity of organic-dominated aerosols over the Pearl River Delta region in China, Atmos. Chem. Phys., 18, 14079–14094, https://doi.org/10.5194/acp-18-14079-2018, 2018.
Hua, Y., Wang, S., Jiang, J., Zhou, W., Xu, Q., Li, X., Liu, B., Zhang, D., and Zheng, M.: Characteristics and sources of aerosol pollution at a polluted rural site southwest in Beijing, China, Sci. Total Environ., 626, 519–527, https://doi.org/10.1016/j.scitotenv.2018.01.047, 2018.
Jeong, C. H., Hopke, P. K., Kim, E., and Lee, D. W.: The comparison between thermal-optical transmittance elemental carbon and Aethalometer black carbon measured at multiple monitoring sites, Atmos. Environ., 38, 5193–5204, https://doi.org/10.1016/j.atmosenv.2004.02.065, 2004.
Jiang, R., Tan, H., Tang, L., Cai, M., Yin, Y., Li, F., Liu, L., Xu, H., Chan, P. W., Deng, X., and Wu, D.: Comparison of aerosol hygroscopicity and mixing state between winter and summer seasons in Pearl River Delta region, China, Atmos. Res., 169, 160–170, https://doi.org/10.1016/j.atmosres.2015.09.031, 2016.
Kreyling, W. G., Semmler-Behnke, M., and Möller, W.: Health implications of nanoparticles, J. Nanopart. Res., 8, 543–562, https://doi.org/10.1007/s11051-005-9068-z, 2006.
Kuang, Y., He, Y., Xu, W., Yuan, B., Zhang, G., Ma, Z., Wu, C., Wang, C., Wang, S., Zhang, S., Tao, J., Ma, N., Su, H., Cheng, Y., Shao, M., and Sun, Y.: Photochemical Aqueous-Phase Reactions Induce Rapid Daytime Formation of Oxygenated Organic Aerosol on the North China Plain, Environ. Sci. Technol., 54, 3849–3860, https://doi.org/10.1021/acs.est.9b06836, 2020.
Li, G., Su, H., Ma, N., Tao, J., Kuang, Y., Wang, Q., Hong, J., Zhang, Y., Kuhn, U., Zhang, S., Pan, X., Lu, N., Tang, M., Zheng, G., Wang, Z., Gao, Y., Cheng, P., Xu, W., Zhou, G., Zhao, C., Yuan, B., Shao, M., Ding, A., Zhang, Q., Fu, P., Sun, Y., Poschl, U., and Cheng, Y.: Multiphase chemistry experiment in Fogs and Aerosols in the North China Plain (McFAN): Integrated analysis and intensive winter campaign 2018, Faraday Discuss., 226, 207–222, https://doi.org/10.1039/d0fd00099j, 2021.
Li, L., Hoffmann, M. R., and Colussi, A. J.: Role of Nitrogen Dioxide in the Production of Sulfate during Chinese Haze-Aerosol Episodes, Environ. Sci. Technol., 52, 2686–2693, https://doi.org/10.1021/acs.est.7b05222, 2018.
Liu, H. J., Zhao, C. S., Nekat, B., Ma, N., Wiedensohler, A., van Pinxteren, D., Spindler, G., Müller, K., and Herrmann, H.: Aerosol hygroscopicity derived from size-segregated chemical composition and its parameterization in the North China Plain, Atmos. Chem. Phys., 14, 2525–2539, https://doi.org/10.5194/acp-14-2525-2014, 2014.
Liu, P., Ye, C., Xue, C., Zhang, C., Mu, Y., and Sun, X.: Formation mechanisms of atmospheric nitrate and sulfate during the winter haze pollution periods in Beijing: gas-phase, heterogeneous and aqueous-phase chemistry, Atmos. Chem. Phys., 20, 4153–4165, https://doi.org/10.5194/acp-20-4153-2020, 2020.
Liu, P. F., Zhao, C. S., Göbel, T., Hallbauer, E., Nowak, A., Ran, L., Xu, W. Y., Deng, Z. Z., Ma, N., Mildenberger, K., Henning, S., Stratmann, F., and Wiedensohler, A.: Hygroscopic properties of aerosol particles at high relative humidity and their diurnal variations in the North China Plain, Atmos. Chem. Phys., 11, 3479–3494, https://doi.org/10.5194/acp-11-3479-2011, 2011.
Martin, S. T., Hung, H.-M., Park, R. J., Jacob, D. J., Spurr, R. J. D., Chance, K. V., and Chin, M.: Effects of the physical state of tropospheric ammonium-sulfate-nitrate particles on global aerosol direct radiative forcing, Atmos. Chem. Phys., 4, 183–214, https://doi.org/10.5194/acp-4-183-2004, 2004.
Massling, A., Stock, M., Wehner, B., Wu, Z. J., Hu, M., Brüggemann, E., Gnauk, T., Herrmann, H., and Wiedensohler, A.: Size segregated water uptake of the urban submicrometer aerosol in Beijing, Atmos. Environ., 43, 1578–1589, https://doi.org/10.1016/j.atmosenv.2008.06.003, 2009.
McFiggans, G., Artaxo, P., Baltensperger, U., Coe, H., Facchini, M. C., Feingold, G., Fuzzi, S., Gysel, M., Laaksonen, A., Lohmann, U., Mentel, T. F., Murphy, D. M., O'Dowd, C. D., Snider, J. R., and Weingartner, E.: The effect of physical and chemical aerosol properties on warm cloud droplet activation, Atmos. Chem. Phys., 6, 2593–2649, https://doi.org/10.5194/acp-6-2593-2006, 2006.
Ng, N. L., Canagaratna, M. R., Zhang, Q., Jimenez, J. L., Tian, J., Ulbrich, I. M., Kroll, J. H., Docherty, K. S., Chhabra, P. S., Bahreini, R., Murphy, S. M., Seinfeld, J. H., Hildebrandt, L., Donahue, N. M., DeCarlo, P. F., Lanz, V. A., Prévôt, A. S. H., Dinar, E., Rudich, Y., and Worsnop, D. R.: Organic aerosol components observed in Northern Hemispheric datasets from Aerosol Mass Spectrometry, Atmos. Chem. Phys., 10, 4625–4641, https://doi.org/10.5194/acp-10-4625-2010, 2010.
Paatero, P. and Tapper, U.: Positive matrix factorization: A non-negative factor model with optimal utilization of error estimates of data values, Environmetrics, 5, 111–126, https://doi.org/10.1002/env.3170050203, 1994.
Petters, M. D. and Kreidenweis, S. M.: A single parameter representation of hygroscopic growth and cloud condensation nucleus activity, Atmos. Chem. Phys., 7, 1961–1971, https://doi.org/10.5194/acp-7-1961-2007, 2007.
Petters, M. D. and Kreidenweis, S. M.: A single parameter representation of hygroscopic growth and cloud condensation nucleus activity – Part 2: Including solubility, Atmos. Chem. Phys., 8, 6273–6279, https://doi.org/10.5194/acp-8-6273-2008, 2008.
Qi, X., Sun, J., Zhang, L., Shen, X., Zhang, X., Zhang, Y., Wang, Y., Che, H., Zhang, Z., Zhong, J., Tan, K., Zhao, H., and Ren, S.: Aerosol Hygroscopicity during the Haze Red-Alert Period in December 2016 at a Rural Site of the North China Plain, J. Meteorol. Res., 32, 38–48, https://doi.org/10.1007/s13351-018-7097-7, 2018.
Rose, D., Nowak, A., Achtert, P., Wiedensohler, A., Hu, M., Shao, M., Zhang, Y., Andreae, M. O., and Pöschl, U.: Cloud condensation nuclei in polluted air and biomass burning smoke near the mega-city Guangzhou, China – Part 1: Size-resolved measurements and implications for the modeling of aerosol particle hygroscopicity and CCN activity, Atmos. Chem. Phys., 10, 3365–3383, https://doi.org/10.5194/acp-10-3365-2010, 2010.
Sloane, C. S. and Wolff, G. T.: Prediction of ambient light scattering using a physical model responsive to relative humidity: Validation with measurements from detroit, Atmos. Environ., 19, 669–680, https://doi.org/10.1016/0004-6981(85)90046-0, 1985.
Shen, C., Zhao, G., Zhao, W., Tian, P., and Zhao, C.: Measurement report: aerosol hygroscopic properties extended to 600 nm in the urban environment, Atmos. Chem. Phys., 21, 1375–1388, https://doi.org/10.5194/acp-21-1375-2021, 2021.
Shi, J. N.: Data for “On the difference of aerosol hygroscopicity between high and low RH conditions in the North China Plain”, Zenodo [data set], https://doi.org/10.5281/zenodo.5758744, 2021.
Sun, Y., Xu, W., Zhang, Q., Jiang, Q., Canonaco, F., Prévôt, A. S. H., Fu, P., Li, J., Jayne, J., Worsnop, D. R., and Wang, Z.: Source apportionment of organic aerosol from 2 year highly time-resolved measurements by an aerosol chemical speciation monitor in Beijing, China, Atmos. Chem. Phys., 18, 8469–8489, https://doi.org/10.5194/acp-18-8469-2018, 2018.
Sun, Y. L., Zhang, Q., Anastasio, C., and Sun, J.: Insights into secondary organic aerosol formed via aqueous-phase reactions of phenolic compounds based on high resolution mass spectrometry, Atmos. Chem. Phys., 10, 4809–4822, https://doi.org/10.5194/acp-10-4809-2010, 2010.
Sun, Y. L., Wang, Z. F., Fu, P. Q., Yang, T., Jiang, Q., Dong, H. B., Li, J., and Jia, J. J.: Aerosol composition, sources and processes during wintertime in Beijing, China, Atmos. Chem. Phys., 13, 4577–4592, https://doi.org/10.5194/acp-13-4577-2013, 2013.
Sun, Y. L., Wang, Z. F., Du, W., Zhang, Q., Wang, Q. Q., Fu, P. Q., Pan, X. L., Li, J., Jayne, J., and Worsnop, D. R.: Long-term real-time measurements of aerosol particle composition in Beijing, China: seasonal variations, meteorological effects, and source analysis, Atmos. Chem. Phys., 15, 10149–10165, https://doi.org/10.5194/acp-15-10149-2015, 2015.
Swietlicki, E., Hansson, H. C., Hämeri, K., Svenningsson, B., Massling, A., McFiggans, G., McMurry, P. H., Petäjä, T., Tunved, P., Gysel, M., Topping, D., Weingartner, E., Baltensperger, U., Rissler, J., Wiedensohler, A., and Kulmala, M.: Hygroscopic properties of submicrometer atmospheric aerosol particles measured with H-TDMA instruments in various environments – a review, Tellus B, 60, 432–469, https://doi.org/10.1111/j.1600-0889.2008.00350.x, 2008.
Tan, H., Xu, H., Wan, Q., Li, F., Deng, X., Chan, P. W., Xia, D., and Yin, Y.: Design and application of an unattended multifunctional H-TDMA system, J. Atmos. Ocean. Tech., 30, 1136–1148, https://doi.org/10.1175/JTECH-D-12-00129.1, 2013.
Tao, W. K., Chen, J. P., Li, Z., Wang, C., and Zhang, C.: Impact of Aerosols on Convective Clouds and Precipitation, Rev. Geophys., 50, 1–62, https://doi.org/10.1029/2011RG000369, 2012.
Tong, Y., Pospisilova, V., Qi, L., Duan, J., Gu, Y., Kumar, V., Rai, P., Stefenelli, G., Wang, L., Wang, Y., Zhong, H., Baltensperger, U., Cao, J., Huang, R.-J., Prévôt, A. S. H., and Slowik, J. G.: Quantification of solid fuel combustion and aqueous chemistry contributions to secondary organic aerosol during wintertime haze events in Beijing, Atmos. Chem. Phys., 21, 9859–9886, https://doi.org/10.5194/acp-21-9859-2021, 2021.
Ulbrich, I. M., Canagaratna, M. R., Zhang, Q., Worsnop, D. R., and Jimenez, J. L.: Interpretation of organic components from Positive Matrix Factorization of aerosol mass spectrometric data, Atmos. Chem. Phys., 9, 2891–2918, https://doi.org/10.5194/acp-9-2891-2009, 2009.
Wang, H., Lu, K., Chen, X., Zhu, Q., Wu, Z., Wu, Y., and Sun, K.: Fast particulate nitrate formation via N2O5 uptake aloft in winter in Beijing, Atmos. Chem. Phys., 18, 10483–10495, https://doi.org/10.5194/acp-18-10483-2018, 2018.
Wang, X., Shen, X. J., Sun, J. Y., Zhang, X. Y., Wang, Y. Q., Zhang, Y. M., Wang, P., Xia, C., Qi, X. F., and Zhong, J. T.: Size-resolved hygroscopic behavior of atmospheric aerosols during heavy aerosol pollution episodes in Beijing in December 2016, Atmos. Environ., 194, 188–197, https://doi.org/10.1016/j.atmosenv.2018.09.041, 2018.
Wang, Y., Li, Z., Zhang, Y., Du, W., Zhang, F., Tan, H., Xu, H., Fan, T., Jin, X., Fan, X., Dong, Z., Wang, Q., and Sun, Y.: Characterization of aerosol hygroscopicity, mixing state, and CCN activity at a suburban site in the central North China Plain, Atmos. Chem. Phys., 18, 11739–11752, https://doi.org/10.5194/acp-18-11739-2018, 2018a.
Wang, Y., Wu, Z., Ma, N., Wu, Y., Zeng, L., Zhao, C., and Wiedensohler, A.: Statistical analysis and parameterization of the hygroscopic growth of the sub-micrometer urban background aerosol in Beijing, Atmos. Environ., 175, 184–191, https://doi.org/10.1016/j.atmosenv.2017.12.003, 2018b.
Wang, Y., Chen, Y., Wu, Z., Shang, D., Bian, Y., Du, Z., Schmitt, S. H., Su, R., Gkatzelis, G. I., Schlag, P., Hohaus, T., Voliotis, A., Lu, K., Zeng, L., Zhao, C., Alfarra, M. R., McFiggans, G., Wiedensohler, A., Kiendler-Scharr, A., Zhang, Y., and Hu, M.: Mutual promotion between aerosol particle liquid water and particulate nitrate enhancement leads to severe nitrate-dominated particulate matter pollution and low visibility, Atmos. Chem. Phys., 20, 2161–2175, https://doi.org/10.5194/acp-20-2161-2020, 2020.
Wu, Z. J., Poulain, L., Henning, S., Dieckmann, K., Birmili, W., Merkel, M., van Pinxteren, D., Spindler, G., Müller, K., Stratmann, F., Herrmann, H., and Wiedensohler, A.: Relating particle hygroscopicity and CCN activity to chemical composition during the HCCT-2010 field campaign, Atmos. Chem. Phys., 13, 7983–7996, https://doi.org/10.5194/acp-13-7983-2013, 2013.
Wu, Z. J., Zheng, J., Shang, D. J., Du, Z. F., Wu, Y. S., Zeng, L. M., Wiedensohler, A., and Hu, M.: Particle hygroscopicity and its link to chemical composition in the urban atmosphere of Beijing, China, during summertime, Atmos. Chem. Phys., 16, 1123–1138, https://doi.org/10.5194/acp-16-1123-2016, 2016.
Xu, W., Chen, C., Qiu, Y., Xie, C., Chen, Y., Ma, N., Xu, W., Fu, P., Wang, Z., Pan, X., Zhu, J., Ng, N. L., and Sun, Y.: Size-resolved characterization of organic aerosol in the North China Plain: new insights from high resolution spectral analysis, Environ. Sci. Atmos., 1, 346–358, https://doi.org/10.1039/d1ea00025j, 2021.
Yang, Y., Wang, H., Smith, S. J., Zhang, R., Lou, S., Qian, Y., Ma, P. L., and Rasch, P. J.: Recent intensification of winter haze in China linked to foreign emissions and meteorology, Sci. Rep., 8, 1–10, https://doi.org/10.1038/s41598-018-20437-7, 2018.
Ye, X., Ma, Z., Hu, D., Yang, X., and Chen, J.: Size-resolved hygroscopicity of submicrometer urban aerosols in Shanghai during wintertime, Atmos. Res., 99, 353–364, https://doi.org/10.1016/j.atmosres.2010.11.008, 2011.
Yeung, M., Lee, B., Li, Y., and Chan, C.: Simultaneous HTDMA and HR-ToF-AMS measurements at the HKUST supersite in Hong Kong in 2011, J. Geophys. Res.-Atmos., 119, 9864–9883, https://doi.org/10.1002/2013JD021146, 2014.
Yu, L., Smith, J., Laskin, A., Anastasio, C., Laskin, J., and Zhang, Q.: Chemical characterization of SOA formed from aqueous-phase reactions of phenols with the triplet excited state of carbonyl and hydroxyl radical, Atmos. Chem. Phys., 14, 13801–13816, https://doi.org/10.5194/acp-14-13801-2014, 2014.
Yuan, L., Zhang, X., Feng, M., Liu, X., Che, Y., Xu, H., Schaefer, K., Wang, S., and Zhou, Y.: Size-resolved hygroscopic behaviour and mixing state of submicron aerosols in a megacity of the Sichuan Basin during pollution and fireworks episodes, Atmos. Environ., 226, 117393, https://doi.org/10.1016/j.atmosenv.2020.117393, 2020.
Yue, D. L., Hu, M., Zhang, R. Y., Wu, Z. J., Su, H., Wang, Z. B., Peng, J. F., He, L. Y., Huang, X. F., Gong, Y. G., and Wiedensohler, A.: Potential contribution of new particle formation to cloud condensation nuclei in Beijing, Atmos. Environ., 45, 6070–6077, https://doi.org/10.1016/j.atmosenv.2011.07.037, 2011.
Zhang, S. L., Ma, N., Kecorius, S., Wang, P. C., Hu, M., Wang, Z. B., Größ, J., Wu, Z. J., and Wiedensohler, A.: Mixing state of atmospheric particles over the North China Plain, Atmos. Environ., 125, 152–164, https://doi.org/10.1016/j.atmosenv.2015.10.053, 2016.
Zheng, Y., Cheng, X., Liao, K., Li, Y., Li, Y. J., Huang, R.-J., Hu, W., Liu, Y., Zhu, T., Chen, S., Zeng, L., Worsnop, D. R., and Chen, Q.: Characterization of anthropogenic organic aerosols by TOF-ACSM with the new capture vaporizer, Atmos. Meas. Tech., 13, 2457–2472, https://doi.org/10.5194/amt-13-2457-2020, 2020.