the Creative Commons Attribution 4.0 License.
the Creative Commons Attribution 4.0 License.
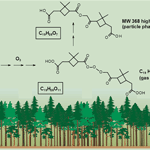
High-molecular-weight esters in α-pinene ozonolysis secondary organic aerosol: structural characterization and mechanistic proposal for their formation from highly oxygenated molecules
Ariane Kahnt
Reinhilde Vermeylen
Yoshiteru Iinuma
Mohammad Safi Shalamzari
Willy Maenhaut
Stable high-molecular-weight esters are present in α-pinene ozonolysis secondary organic aerosol (SOA) with the two most abundant ones corresponding to a hydroxypinonyl ester of cis-pinic acid with a molecular weight (MW) of 368 (C19H28O7) and a diaterpenylic ester of cis-pinic acid with a MW of 358 (C17H26O8). However, their molecular structures are not completely elucidated and their relationship with highly oxygenated molecules (HOMs) in the gas phase is still unclear. In this study, liquid chromatography in combination with positive ion electrospray ionization mass spectrometry has been performed on high-molecular-weight esters present in α-pinene ozonolysis SOA with and without derivatization into methyl esters. Unambiguous evidence could be obtained for the molecular structure of the MW 368 ester in that it corresponds to an ester of cis-pinic acid where the carboxyl substituent of the dimethylcyclobutane ring and not the methylcarboxyl substituent is esterified with 7-hydroxypinonic acid. The same linkage was already proposed in previous work for the MW 358 ester (Yasmeen et al., 2010), but could be supported in the present study. Guided by the molecular structures of these stable esters, we propose a formation mechanism from gas-phase HOMs that takes into account the formation of an unstable C19H28O11 product, which is detected as a major species in α-pinene ozonolysis experiments as well as in the pristine forest atmosphere by chemical ionization–atmospheric pressure ionization–time-of-flight mass spectrometry with nitrate clustering (Ehn et al., 2012, 2014). It is suggested that an acyl peroxy radical related to cis-pinic acid (RO2•) and an alkoxy radical related to 7- or 5-hydroxypinonic acid (R′O•) serve as key gas-phase radicals and combine according to a RO2 + R′O• → RO3R′ radical termination reaction. Subsequently, the unstable C19H28O11 HOM species decompose through the loss of oxygen or ketene from the inner part containing a labile trioxide function and the conversion of the unstable acyl hydroperoxide groups to carboxyl groups, resulting in stable esters with a molecular composition of C19H28O7 (MW 368) and C17H26O8 (MW 358), respectively. The proposed mechanism is supported by several observations reported in the literature. On the basis of the indirect evidence presented in this study, we hypothesize that RO2 + R′O• → RO3R′ chemistry is at the underlying molecular basis of high-molecular-weight ester formation upon α-pinene ozonolysis and may thus be of importance for new particle formation and growth in pristine forested environments.
- Article
(3111 KB) - Full-text XML
-
Supplement
(699 KB) - BibTeX
- EndNote
The molecular characterization of secondary organic aerosol (SOA) has been a topic of interest in atmospheric chemistry for the last decades, owing to the importance of organic aerosol in air quality and climate (for a review, see Nozière et al., 2015). SOA comprises a large number of oxygenated organic compounds and is a major constituent of submicrometer atmospheric particulate matter (PM), and both biogenic (e.g., isoprene, monoterpenes, sesquiterpenes) and anthropogenic (aromatics, n-alkanes) volatile organic compounds (VOCs) serve as precursors for SOA. Abundant biogenic VOCs in the terrestrial atmosphere are monoterpenes, having an annual global emission rate of 155 Tg with α-pinene as the major terpene emitted (Guenther et al., 2012). Several multifunctional SOA compounds, including monomers and dimers from α-pinene oxidation have been structurally identified (for a review, see Nozière et al., 2015). Recently, “extremely low-volatility organic compounds” (ELVOCs), currently termed “highly oxygenated molecules” (HOMs), originating from α-pinene ozonolysis (α-pinene/O3) have been detected in both laboratory and field experiments by chemical ionization–atmospheric pressure ionization–time-of-flight (CI-APi-TOF) mass spectrometry with nitrate clustering (Ehn et al., 2012, 2014; Zhao et al., 2013) and have received much attention because of their role in driving new particle formation and growth in pristine forested environments. Molecular characterization of α-pinene SOA constituents is needed to elucidate the underlying formation mechanism and establish its link with gas-phase HOMs, and efforts in this direction have recently been undertaken (Mutzel et al., 2015; Zhang et al., 2015, 2017; Krapf et al., 2016). However, the relationship of HOMs detected in the gas phase upon α-pinene ozonolysis with stable high-molecular-weight SOA constituents is unclear, so that there is still a missing element in closing the α-pinene SOA system.
High-molecular-weight esters have been reported in α-pinene/O3 SOA but their detailed chemical structures are only partially elucidated and their mechanism of formation is still elusive. A high-molecular-weight compound with a molecular weight (MW) of 358 was reported for the first time by Hoffmann et al. (1998) in α-pinene/O3 SOA using off- and online mass spectrometry (MS). With online atmospheric pressure chemical ionization (APCI) MS it was shown that this compound is formed concomitantly with two monomers, i.e., cis-pinic acid and a MW 172 compound that was tentatively identified as norpinic acid. Tandem MS on the deprotonated compound (m∕z 357) revealed that it has a cis-pinic acid residue (m∕z 185) as well as a m∕z 171 residue. Later work by Müller et al. (2008) focused on the structure of the MW 368 compound. It was shown that this compound is composed of cis-pinic and hydroxypinonic acid parts, which are linked together by an ester bridge. The structure of the MW 358 compound was also addressed by Yasmeen et al. (2010), who revised the structure of this compound and presented evidence that it is a diaterpenylic ester of cis-pinic acid. The same conclusion was reached by Gao et al. (2010), who also showed that the MW 358 ester is a major product in α-pinene ozonolysis experiments performed at low mass loadings. Recent work by Beck and Hoffmann (2016), where use was made of derivatization to the n-butylesters and subsequent tandem MS analysis of the lithiated and ammoniated molecules, supported the structure of the MW 358 ester as a diaterpenylic ester of cis-pinic acid. Furthermore, the MW 358 ester was detected as a major tracer in β-pinene ozonolysis SOA characterization studies (Iinuma et al., 2007; Yasmeen et al., 2010).
It is noted that prior to the studies by Müller et al. (2008) and Yasmeen et al. (2010) several studies dealt with the molecular characterization of high-molecular-weight compounds and that very different possible structures have been advanced. Gao et al. (2004) assigned the MW 358 α-pinene/O3 compound to a dehydration product formed between the gem-diol forms of two norpinonic acid molecules. Iinuma et al. (2004) reported MW 354 and 370 α-pinene/O3 products that were enhanced in acidic conditions and tentatively assigned them to reaction products between the gem-diol of pinonaldehyde and pinonaldehyde, and between pinonaldehyde and hydroxypinonaldehyde, respectively. Docherty et al. (2005) proposed peroxycarboxylic acid dimers for the structure of higher-MW SOA products from the ozonolysis of α-pinene in which peroxypinic acid and the gem-diol of a keto or aldehydic compound are connected via a peroxy bridge. Tolocka et al. (2004) characterized high-molecular-weight compounds in α-pinene ozonolysis SOA and suggested that the products were most likely formed by aldol and/or gem-diol formation. In addition, Witkowski and Gierczak (2014) explained the formation of MW 338 and 352 compounds in α-pinene ozonolysis as aldol reaction products of α-acyloxyhydroperoxy aldehydes. All the abovementioned studies thus provide evidence that the structure elucidation of high-molecular-weight α-pinene/O3 compounds has turned out to be very challenging.
With regard to the structure elucidation of the MW 358 ester there is still ambiguity, in that two positional isomers are possible (Fig. 1), and that different positional isomers have been proposed by Yasmeen et al. (2010) (structure a), Gao et al. (2010) (structure b), and Beck and Hoffmann (2016) (structure b). Based on the MS data obtained it is not possible to unambiguously support the structure of one or the other positional isomer. This issue will be further addressed in Sect. 3. The same ambiguity holds for the MW 368 ester (Fig. 1). In addition to the MW 358 and 368 esters, minor high-molecular-weight compounds (i.e., MWs 272, 300, 308, 312, 314, 326, 338, 344, 352, 356, 376, 378 and 400) have also been reported in α-pinene/O3 SOA (Müller et al., 2008; Yasmeen et al., 2010; Kourtchev et al., 2014; Witkowski and Gierczak, 2014; Zhang et al., 2015) but these products will not be addressed in the present paper.
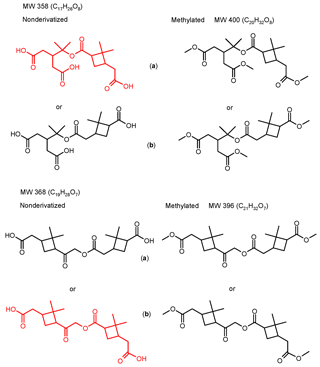
Figure 1Overview of the proposed high-molecular-weight ester compounds present in α-pinene/O3 SOA which were investigated in the present study. The compounds present in underivatized α-pinene/O3 SOA are highlighted in red color.
High-molecular-weight esters have been detected up until now in many field studies that were conducted in forested regions. MW 358 and 368 esters were first reported in ambient nighttime PM with an aerodynamic diameter ≤ 2.5 µM (PM2.5) that was collected at K-puszta, Hungary, during a 2006 summer campaign (Yasmeen et al., 2010). They were later detected in several field studies that were conducted in other forested environments (Kristensen et al., 2013, 2016; Kourtchev et al., 2014, 2015). It was shown by Kourtchev et al. (2016) that oligomers (i.e., hetero-oligomers) are of climatic relevance in that elevated SOA mass is one of the key drivers of oligomer formation not only in laboratory experiments but also in the ambient atmosphere. It was also demonstrated in the latter study that the oligomer content is strongly correlated with cloud condensation nuclei activities of SOA particles. Furthermore, it could be demonstrated in laboratory chamber experiments that the ratio of monomers to oligomers and the oligomer content in α-pinene ozonolysis SOA are enhanced at low temperature and low precursor concentrations, conditions that are relevant for the upper troposphere (Huang et al., 2018).
Efforts to understand ester formation from α-pinene ozonolysis have also been actively undertaken. Yasmeen et al. (2010) proposed that ester formation took place in the particle phase by esterification of cis-pinic acid with terpenylic acid but this mechanism was not retained in later studies. Kristensen et al. (2014) demonstrated their formation through gas-phase ozonolysis and supported the participation of a stabilized Criegee intermediate, as previously suggested for the formation of unstable high-molecular-weight compounds that play a role in new particle formation (Ziemann, 2002; Bonn et al., 2002; Lee and Kamens, 2005). In a study by Zhang et al. (2015), the dynamics of particle-phase components of α-pinene SOA formation were investigated in detail. It was shown that formation of monomeric products like cis-pinic acid is observed after the consumption of α-pinene upon ozonolysis, which cannot be explained solely by a gas-phase mechanism and points to a particle-phase mechanism. A mechanism involving gas-phase radical combination of acyl peroxy radicals and a condensed-phase rearrangement was proposed that potentially explains the α-pinene SOA features in terms of molecular structure, abundance, growth rates, evolution patterns, and responses to variations in temperature, relative humidity, and oxidant type. Furthermore, a recent study by Zhang et al. (2017), using ozonolysis of deuterium-labeled α-pinene, demonstrated that hydroperoxy derivatives of pinonic acid containing the hydroperoxy group at different positions are components of HOMs that are present in the particle phase. In work prior to the above-cited investigations, other studies already suggested the involvement of acyl peroxy radicals in the formation of HOMs upon α-pinene ozonolysis (Ziemann, 2002; Docherty et al., 2005). In addition, the suggestion that peroxy radicals are involved in the formation of dimers also fits to the observation of a suppression of new particle formation from monoterpene oxidation by NOx (Wildt et al., 2014). Furthermore, evidence for peroxyhemiacetal formation upon α-pinene ozonolysis has also been reported (Hall and Johnston, 2012a). All the above-cited studies thus document that establishing the underlying molecular mechanism leading to ester formation in α-pinene ozonolysis is challenging. This is mainly due to a lack of knowledge (or only a partial knowledge, i.e., molecular formulae) of the molecular structures of both gas-phase intermediates and particulate-phase end products.
In the present paper, we focus on the structural characterization of the MW 358 and 368 esters that are present in α-pinene/O3 SOA. To this aim, we have performed liquid chromatography with electrospray ionization mass spectrometry (LC/ESI-MS) in the positive ion mode on α-pinene/O3 SOA with and without derivatization into methyl esters. A soft methylation procedure using ethereal diazomethane was selected to avoid hydrolysis of the ester function present in the targeted hetero-dimers. The aim of the methylation was twofold: on the one hand, to confirm the number of free carboxyl functions, and on the other hand, to obtain mass spectrometric fragmentation that is different from that of intact esters in (+)ESI and to that obtained in previous studies on intact esters in (−)ESI (Müller et al., 2008; Yasmeen et al., 2010; Zhang et al., 2015). Led by the molecular structures of the MW 368 and 358 esters, we propose a formation mechanism that takes into account the detection of a C19 HOM in the gas phase by CI-APi-TOF MS with nitrate clustering (Ehn et al., 2012, 2014; Zhao et al., 2013) and involves the combination of an acyl peroxy radical related to cis-pinic acid with an alkoxy radical related to isomeric hydroxypinonic acids, which are, like cis-pinic acid, major monomers in α-pinene SOA.
2.1 α-pinene/O3 chamber aerosol
Filter samples from α-pinene ozonolysis were obtained from experiments carried out in the 19 m3 TROPOS aerosol chamber at 50 % relative humidity and 21 ∘C. A concentration of 1.6 ppm α-pinene was reacted with 615 ppb ozone without seed particles and no OH radical scavenger was added. The aerosol formed was sampled after about 1 h of reaction time using a quartz fibre filter, and the sample was stored at −22 ∘C before analysis.
2.2 Filter sample preparation for analysis
A quarter of the chamber aerosol filter was extracted using 3 times 10 mL methanol and applying ultrasonic agitation for 3 min. The combined extracts were concentrated to about 1 mL at 35 ∘C with a rotary evaporator, were transferred to a 1 mL reaction glass vial, and were blown to dryness under a stream of nitrogen. The dried residue was reconstituted in 250 µL methanol ∕ water (50 ∕ 50, v ∕ v) and analyzed by LC/(+)ESI-MS to characterize the nonderivatized dimers. Another quarter of the filter was similarly extracted but was further subjected to a methylation procedure using ethereal diazomethane to derivatize free carboxylic acids into their corresponding methyl esters. Diazomethane was freshly prepared using the precursor diazald (99 %, Sigma-Aldrich) according to a standard procedure (Furniss et al., 1989). A total of 500 µL from the ethereal diazomethane solution was added to the dried filter extract. After a reaction time of about 5 min, the sample was dried under a gentle stream of nitrogen and reconstituted in 250 µL methanol ∕ water (50 ∕ 50, v ∕ v) for LC/(+)ESI-MS analysis of methylated compounds.
2.3 Chemical analysis
LC/ESI-MS analysis was carried out using a Surveyor Plus system (pump and autosampler) (Thermo Scientific, San Jose, CA, USA) and the chromatographic separation for both the nonderivatized and the derivatized filter extracts was performed on an Atlantis T3 column (2.1 × 150 nm, 3 µm particle size, Waters, Milford, MA, USA). An injection volume of 10 µL was used and a flow rate of 0.2 mL min−1 was applied. The mobile phases consisted of (A) 50 mM ammonium formate buffer with pH 3 and (B) methanol. A 65 min gradient was applied using the following program: (B) was kept at 3 % for 5 min, increased to 95 % in 15 min and kept for 25 min, followed by the reconditioning to 3 % in 10 min and keeping (B) at 3 % for 10 min. A linear ion trap mass spectrometer (LXQ, Thermo Scientific, San Jose, CA, USA) operated in the positive mode was used as the mass analyzer and details regarding operational and optimization procedures can be found in Kahnt et al. (2014). Under the LC conditions used, ammoniated adducts were detected owing to the presence of ammonium formate in mobile phase (A). The ion abundance ratios [M + NH4]+ ∕ [M + H]+ were 13.0, 15.6, 38 and 7.7, for the MW 358 ester, MW 368 ester, MW 358 ester trimethylated derivative and MW 368 ester dimethylated derivative, respectively. In the ion trap MSn experiments, ammonia adducts were selected as precursor ions because these ions were more abundant than the protonated molecules and lose ammonia upon MS2, resulting in protonated molecules of which the fragmentation can be readily explained.
3.1 Characterization of the MW 358 and 368 high-molecular weight esters
3.1.1 Previous studies on [M − H]−, [M + NH4]+ and [M + Li]+ molecular species
For clarity, here we summarize selected MS data already reported in a previous study (Yasmeen et al., 2010) that led to the structural characterization of the MW 358 ester from α-pinene ozonolysis SOA as a diaterpenylic ester of cis-pinic acid. The data are given in Sect. 1 of the Supplement (Fig. S1 and Scheme S1). Only one MW 358 isomer was detected in α-pinene/O3 SOA; upon MS2 its deprotonated molecule [M − H]− fragments to product ions at m∕z 185 and 171, which are attributed to cis-pinic and diaterpenylic acid, respectively. However, based on this information alone the ester linkage cannot be firmly established since two positional isomers are possible (Fig. 1). A minor MW 358 isomer was also detected in β-pinene ozonolysis SOA, which was very similar to that observed from α-pinene but showed an additional MS2 [M − H]− product ion at m∕z 189, which could best be explained with a positional isomeric structure (structure b; Fig. 1). MS2 data for the latter product are presented in Fig. S2 and Scheme S2. More recent work by Beck and Hoffmann (2016) involving fragmentation of lithiated and ammoniated molecular species of the n-butylated derivative supported the structure of the MW 358 ester from α-pinene/O3 SOA as a diaterpenylic ester of cis-pinic acid; however, these authors suggested a positional isomeric structure (structure b; Fig. 1) which is different from that proposed by Yasmeen et al. (2010) (structure a; Fig. 1). The MS data obtained for the [M + NH4]+ and [M + Li]+ molecular species of the n-butylated derivative also do not enable unambiguous differentiation between positional isomeric structures.
For both the MW 358 and 368 esters accurate mass measurements to obtain the molecular compositions have also been performed in previous studies using (−)ESI (e.g., Zhang et al., 2015), and are not repeated in the present study. The molecular compositions of the MW 358 and 368 esters are C17H26O8 and C19H28O7, respectively.
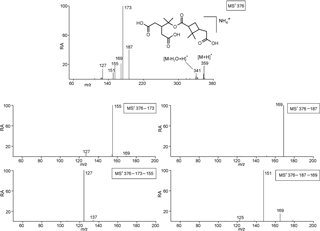
Figure 2Selected LC/(+)ESI-MS data for the nonderivatized MW 358 compound eluting at 24.7 min (Fig. S3) with its proposed structure in α-pinene/O3 SOA, showing the MS2 data for its ammonium adduct ion at m∕z 376, m∕z 376 → m∕z 173 MS3 data, m∕z 376 → m∕z 173 → m∕z 155 MS4 data, m∕z 376 → m∕z 187 MS3 data and m∕z 376 → m∕z 187 → m∕z 169 MS4 data.
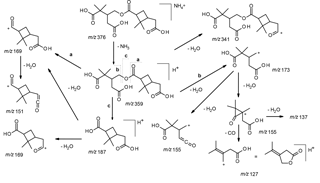
Scheme 1Proposed fragmentation mechanism for the ammoniated nonderivatized MW 358 ester present in α-pinene/O3 SOA.
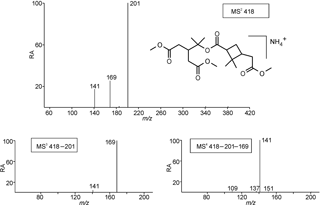
Figure 3Selected LC/(+)ESI-MS data for the trimethylated MW 358 compound eluting at 28.0 min (Fig. S4) with its proposed structure in α-pinene/O3 SOA, showing the MS2 data for its ammonium adduct ion at m∕z 418, m∕z 418 → m∕z 201 MS3 data and m∕z 418 → m∕z 201 → m∕z 169 MS4 data.
3.1.2 Mass spectrometric behavior of the ammoniated underivatized MW 358 ester
LC chromatographic data obtained for underivatized α-pinene/O3 SOA are provided in Fig. S3. It can be seen that the MW 358 product signal in both the negative (m∕z 357) and positive ion mode (m∕z 376) has about half the intensity of the m∕z 367 (MW 368) signal, and shows intensities in the same range as the monomers detected at m∕z 171 (MW 172; terpenylic acid), m∕z 185 (MW 186; cis-pinic acid), and m∕z 199 (MW 200; hydroxypinonic acids).
Selected LC/(+)ESI-MS data for the nonderivatized MW 358 ester with its proposed structure in α-pinene/O3 SOA are presented in Fig. 2 and Scheme 1. Fragmentation of the [M + NH4]+ leads to the loss of ammonia (m∕z 359), yielding [M + H]+, and further loss of a molecule of water (m∕z 341), which can occur at different positions. Abundant product ions are observed at m∕z 173 and 187, which can be rationalized by processes located in the internal ester linkage. The m∕z 169 product ion can be explained through protonation of the ester group (pathway a) or through a hydrogen rearrangement (pathway c) resulting in protonated cis-pinic acid (m∕z 187) and subsequent loss of a molecule of water. However, it is noted that with a positional isomeric structure product ions at the same m∕z values could be expected. The m∕z 173 ion results from protonation of the inner ester group (pathway b) and can subsequently lose one or two molecules of water, giving rise to m∕z 155 and 137, respectively. It can also be seen that m∕z 155 can lead to the loss of CO, giving rise to m∕z 127. The m∕z 169 ion fragments further through the loss of water, leading to m∕z 151; here, we expect that the loss of water proceeds more readily from structure (a) (Fig. 1) as water elimination from structure (b) would lead to strain in the dimethylcyclobutane ring. We therefore retain structure (a) as the most likely structure for the major MW 358 ester present in α-pinene/O3 SOA.
3.1.3 Mass spectrometric behavior of the ammoniated MW 358 ester trimethylated derivative
LC chromatographic data obtained for methylated α-pinene/O3 SOA are provided in Fig. S4. It can be seen that the signal corresponding to the MW 358 ester detected at m∕z 418 has a comparable intensity as that corresponding to the MW 368 ester detected at m∕z 414. The mass shifts observed due to derivatization into methyl esters confirm that the MW 358 compound contains three carboxyl groups, while the MW 368 compound contains two such groups. The corresponding methylated monomers, i.e., terpenylic acid (detected at m∕z 204), cis-pinic acid (detected at m∕z 232) and hydroxypinonic acid (detected at m∕z 232), show intensities in the same range as the methylated MW 358 and 368 esters.
Selected LC/(+)ESI-MS data for the derivatized MW 358 ester with its proposed structure in α-pinene/O3 SOA are presented in Fig. 3 and Scheme 2. Fragmentation of the [M + NH4]+ ion (m∕z 418) leads to the formation of three product ions at m∕z 201, 169 and 141, while further fragmentation of m∕z 201 upon MS3 mainly leads to m∕z 169, and MS4 of the generated m∕z 169 mainly results in m∕z 141. Two different structures can be proposed for m∕z 201; structure (a) can be explained following the loss of ammonia and ionization (protonation) at the inner ester linkage, while structure (b) can be rationalized by a hydrogen rearrangement in the inner ester linkage and loss of ammonia. Further loss of methanol (32 u) from m∕z 201 results in m∕z 169, with two possible structures (c) and (d). It can be seen that structures (c) and (d) can give rise to the loss of CO, resulting in m∕z 141. The weak ion at m∕z 137 can be explained by fragmentation of m∕z 169 (structure c) through loss of methanol. It is noted that the same product ions could be explained from the positional isomeric structure of the derivatized MW 358 ester; however, in this case we would expect a more abundant m∕z 151 product ion, due to a more favorable loss of water in the carboxymethyl terminus. Loss of a molecule of water from m∕z 169 (structure d) leads to a weak product ion at m∕z 151, while further loss of a molecule of ketene also results in m∕z 109.
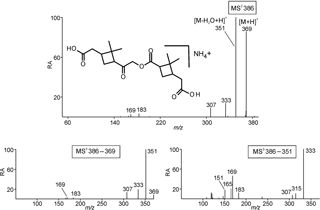
Figure 4Selected LC/(+)ESI-MS data for the nonderivatized MW 368 compound eluting at 25.9 min (Fig. S3) with its proposed structure in α-pinene/O3 SOA, showing the MS2 data for its ammonium adduct ion at m∕z 386, m∕z 386 → m∕z 369 MS3 data and m∕z 386 → m∕z 351 MS3 data.
3.1.4 Mass spectrometric behavior of the ammoniated underivatized MW 368 ester
Selected LC/(+)ESI-MS data for the ammoniated nonderivatized MW 368 ester with its proposed structure in α-pinene/O3 SOA are presented in Fig. 4 and Scheme 3. Fragmentation of the [M + NH4]+ upon MS2 leads to the loss of ammonia (m∕z 369), yielding [M + H]+, and product ions at m∕z 351, 333, 307, 183 and 169, of which m∕z 351 is the base peak, and essentially the same pattern is seen upon MS3 of m∕z 369. The product ions at m∕z 351 and 333 in the higher mass range can simply be explained by the loss of one and two molecules of water, respectively. The loss of CO2 (44 u) leading to m∕z 307 is difficult to explain from a carboxy terminus and likely takes place from the inner ester linkage. The product ion at m∕z 169 can be rationalized through protonation at the inner ester function (route a) and further fragments through loss of water (m∕z 151), as can be seen upon MS3. Similarly, the product ion at m∕z 183 can arise through protonation at the inner ester function (route b) and further loss of water results in m∕z 165. A positional isomeric structure (due to loss of water from the left terminus) can also be suggested for m∕z 351. The ions at m∕z 169 and 183 can also occur after loss of water from the left and right carboxyl terminus, respectively. It is noted that most ions discussed above can also be explained with a positional isomeric structure (Fig. 1; structure a), although we would expect that such a structure would lead to a less pronounced loss of water from m∕z 369 resulting in m∕z 351, as it would result in strain in the dimethylcyclobutane ring. In addition, the formation of m∕z 333, involving a second loss of water, supports the proposed isomeric structure (b) (Fig. 1), as this process is more difficult to explain with isomeric structure (a).
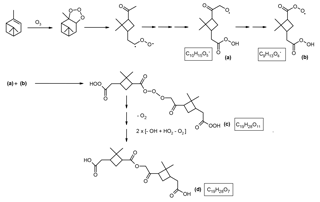
Scheme 5Proposed simplified mechanism leading to the formation of the MW 368 ester with structure (b) (Fig. 1). The mechanisms suggested for the formation of the alkoxy radical related to 7-hydroxypinonic acid (a) and the acyl peroxy radical related to cis-pinic acid (b) are provided in Scheme S4. It is proposed that the latter radicals serve as key intermediates. Radical termination according to a RO2• + R′O• → RO3R′ reaction results in a HOM with a molecular composition of C19H28O11 (c), a major gas-phase species upon α-pinene ozonolysis which has been detected by CI-APi-TOF MS (Ehn et al., 2012, 2014; Krapf et al., 2016). Further degradation of the labile inner part containing a linear trioxide bridge through the loss of oxygen and conversion of the acyl hydroperoxide groups to carboxyl groups results in the MW 368 ester.
3.1.5 Mass spectrometric behavior of the ammoniated MW 368 ester dimethylated derivative
Selected LC/(+)ESI-MS data for the ammoniated MW 368 ester dimethyl derivative with its proposed structure in α-pinene/O3 SOA are presented in Fig. 5 and Scheme 4. Fragmentation of the [M + NH4]+ (m∕z 414) upon MS2 leads to the loss of ammonia (m∕z 397), yielding [M + H]+, and product ions at m∕z 379, 365, 269, 251, 201, 197, 183, 179, 165, 139 and 119, and essentially the same pattern is seen upon MS3 of m∕z 397. The product ions at m∕z 379 and 365 in the higher mass range can simply be explained by the loss of a molecule of water and methanol, respectively, of which the loss of water is due to an enolized keto group, and that of methanol can occur at one of the two methyl ester termini. The product ions at m∕z 201 and 183, observed upon MS2 of m∕z 414 and MS3 of m∕z 397, can be explained through ionization at the inner ester linkage and a hydrogen rearrangement. It is noted that these two product ions do not allow differentiation between positional isomers of the MW 368 ester dimethyl derivative. The product ions at m∕z 269 and 251, observed upon MS2 of m∕z 414 and MS3 of m∕z 397, can be explained by a cross-ring cleavage in the dimethylbutane ring, a fragmentation that has been observed in previous studies for deprotonated cis-pinic acid (Yasmeen et al., 2011) and deprotonated cis-norpinic acid (Yasmeen et al., 2010), both containing a keto group in α-position to the dimethylcyclobutane ring. This fragmentation can be regarded as characteristic for one of the positional isomeric forms, namely, structure (b) (Fig. 1), as it cannot be explained with the other positional isomeric form (a). Further fragmentation upon MS3 of m∕z 379 leads to m∕z 251, 179 and 119, which can be rationalized by the loss of propenoic acid (72 u), and the subsequent combined loss of methanol and carbon monoxide. Thus, the MS data obtained for the ammoniated MW 368 ester dimethylated derivative unambiguously support structure (b).
3.2 Possible formation mechanism for the MW 368 and 358 esters
3.2.1 General mechanistic considerations
It is noted that formation mechanisms involving unstable intermediates are generally hard to formulate as unstable compounds cannot be isolated and structurally characterized; however, the molecular structure of the gas-phase precursor (in this case, α-pinene), its known gas-phase chemistry, the molecular composition of unstable intermediates and the molecular structure of stable end products observed in the particle phase can provide crucial insights. Guided by the molecular structures of the MW 368 (Fig. 1; structure b) and MW 358 esters (Fig. 1; structure a), a formation mechanism is suggested, thereby taking into account that a C19 HOM has been detected as a major high-molecular-weight species in the gas phase upon α-pinene ozonolysis by CI-APi-TOF MS with nitrate clustering (Ehn et al., 2012, 2014; Zhao et al., 2013; Krapf et al., 2016). It is noted that the CI-APi-TOF MS technique does not reveal C19 HOM species that correspond to direct analogues of the MW 358 and 368 esters. In an effort to propose pathways that lead to the formation of the MW 368 and 358 esters, we have tried to formulate a uniform mechanism in that it involves the same acyl peroxy radical related to cis-pinic acid and an alkoxy radical related to isomeric hydroxypinonic acids.
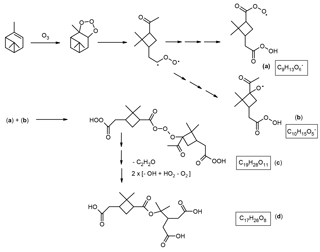
Scheme 6Proposed simplified mechanism leading to the formation of the MW 358 ester with structure (a) (Fig. 1). The mechanisms suggested for the formation of the acyl peroxy radical related to cis-pinic acid (a) and the alkoxy radical related to 5-hydroxypinonic acid (b) are provided in Scheme S4. It is proposed that the latter radicals serve as key intermediates. Radical termination according to a RO2• + R′O• → RO3R′ reaction results in a HOM with a molecular composition of C19H28O11 (c), a major gas-phase species upon α-pinene ozonolysis which has been detected by CI-APi-TOF MS (Ehn et al., 2012, 2014; Krapf et al., 2016). Further degradation of the labile inner part containing a linear trioxide bridge through the loss of ketene and conversion of the acyl hydroperoxide groups to carboxyl groups results in the MW 358 ester.
3.2.2 Formation mechanism proposed for the MW 368 ester
A possible formation mechanism leading to the MW 368 ester is outlined in Scheme 5. It is suggested that an alkoxy radical related to 7-hydroxypinonic acid (a) (cis-2,2-dimethyl-3-hydroxyacetylcyclobutylethanoic acid; for labeling, see Scheme S3) (R′O•) and an acyl peroxy radical related to cis-pinic acid (b) (RO2•) serve as key intermediates. Radical termination according to a RO2• + R′O• → RO3R′ reaction leads to a HOM with a molecular composition of C19H28O11 (c), which corresponds to a major gas-phase species upon α-pinene ozonolysis (Ehn et al., 2012, 2014; Krapf et al., 2016). The proposed mechanism is inspired by the mechanism suggested by Zhang et al. (2015) to explain the formation of a MW 326 ester, where two peroxy radicals related to cis-pinic acid combine according to a RO2• + RO2• → ROOR + O2 reaction. Further degradation of C19H28O11 (c), involving the labile inner part containing a linear trioxide bridge through the loss of oxygen and conversion of the acyl hydroperoxide groups to carboxyl groups, results in the MW 368 ester (Fig. 1; structure b) with a molecular composition of C19H28O7 (d). It is noted that the formation of C19H28O7 corresponds to a RO2• + R′O• → ROR′ + O2 reaction, bearing similarity with the RO2• + RO2• → ROOR + O2 reaction where the R groups are alkyl peroxy groups, which is known to involve a tetroxide intermediate (e.g., Bohr et al., 1999). As to the formation of a linear trioxide intermediate (c), trioxides containing a –(C = O)OOO– function have been reported in the literature, e.g. tertiary alkyl peroxy hydrogen phthalates have been synthesized and are used as catalysts for the polymerization of vinyl compounds (Komai, 1971). Unstable intermediates formed from species (c) can also be considered, owing to the conversion of one acyl hydroperoxy group (C19H28O10), the conversion of two acyl hydroperoxy groups (C19H28O9), the loss of oxygen (C19H28O9) and the loss of oxygen combined with the conversion of one acyl hydroperoxy group (C19H28O8). In this context, such species have been detected in the gas phase by CI-APi-TOF MS with nitrate clustering in an α-pinene ozonolysis flow tube experiment by Krapf et al. (2016). The alternative mechanism leading to C19H28O11 (c) involving an acyloxy radical related to cis-pinic acid and an alkyl peroxy radical related to 7-hydroxypinonic acid is also theoretically possible but is not likely because of the mesomeric stabilization in the acyloxy radical.
With regard to the suggestion that an alkoxy radical related to 7-hydroxypinonic acid is a key gas-phase radical, it should be noted that hydroxypinonic acids are major monomers in α-pinene/O3 SOA (Fig. S3). The detailed mechanism leading to the peroxy radical related to cis-pinic acid (RO2•) and the alkoxy radical related to 7-hydroxypinonic acid (R′O•) are given in Scheme S4. The proposed RO2• + R′O• → RO3R′ radical termination reaction leads to a MW 368 ester with structure (b) (Fig. 1; species d in Scheme 5), namely, an ester of cis-pinic acid where the carboxyl substituent of the dimethylcyclobutane ring and not the carboxymethyl group is esterified with 7-hydroxypinonic acid. It can also be seen that the labile gas-phase intermediate (c) contains cis-pinic acid and 7-hydroxypinonic acid residues and thus can serve as a precursor for these monomers and their corresponding hydroperoxides. In this context, a recent study by Zhang et al. (2017) provided evidence for the formation of 7-hydroperoxypinonic acid from degradation of an unstable dimer precursor in α-pinene/O3 SOA. It is also worth mentioning that both cis-pinic acid (e.g., Yu et al., 1999; Glasius et al., 2000; Larsen et al., 2001; Winterhalter et al., 2003) and 7-hydroxypinonic acid (e.g., Glasius et al., 1999; Larsen et al., 2001; Yasmeen et al., 2012) are known to be present in α-pinene/O3 SOA. The proposed mechanism is consistent with the observation made by Zhang et al. (2015) that cis-pinic acid is still generated after consumption of α-pinene upon ozonolysis, suggesting a particle-phase production pathway. It is also in agreement with observations made by Lopez-Hilfiker et al. (2015) and Huang et al. (2018), who examined the thermal behavior of α-pinene/O3 SOA and found that cis-pinic acid and hydroxypinonic acid can also arise from thermal decomposition of unstable hetero-oligomers. In addition, it is consistent with the findings by Mutzel et al. (2015) that intact HOMs detected in the gas phase are carbonyl-containing compounds. Recent work has also established that hydroperoxides present in α-pinene/O3 SOA are unstable and quickly convert to more stable products (Krapf et al., 2016). Furthermore, monomers including cis-pinic acid and terpenylic acid were found to be major constituents of the 10 and 20 nm particles from α-pinene ozonolysis in a flow reactor (Winkler et al., 2012), which are likely fragments of high-molecular-weight compounds due to the thermal decomposition of unstable hetero-oligomers during resistive heating of particles in the thermal desorption chemical ionization MS measurements (Hall and Johnston, 2012b).
3.2.3 Formation mechanism proposed for the MW 358 ester
A possible formation mechanism leading to the MW 358 ester is provided in Scheme 6. Compared to the mechanism proposed for the MW 368 ester, an alkoxy radical related to 5-hydroxypinonic acid instead of an alkoxy radical related to 7-hydroxypinonic acid participates in the RO2• + R′O• → RO3R′ radical termination reaction. The detailed mechanism leading to the alkoxy radical related to 5-hydroxypinonic acid is given in Scheme S4. It is noted that the C19H28O11 dimeric HOM species is a positional isomer of that implicated in the formation of the MW 368 ester (Scheme 5). With regards to the suggestion that an isomeric alkoxy radical is involved, a recent study by Zhang et al. (2017) provided evidence for the formation of the corresponding hydroperoxy product, 5-hydroperoxypinonic acid, in α-pinene/O3 SOA. As mentioned above, hydroxypinonic acids are major monomers in α-pinene/O3 SOA (Fig. S3), and it can be seen that at least two positional isomeric hydroxypinonic acids are present. To arrive at the formation of the MW 358 ester (Fig. 1; structure a), a complex rearrangement, involving the labile inner part containing a linear trioxide function, has to be invoked. A detailed rearrangement mechanism is provided in Scheme S5. It can also be seen that the labile intermediate (c) (Scheme 6) can serve as a precursor for cis-pinic acid, as it contains a cis-pinic acid residue. In addition, it can be explained that this labile intermediate can also give rise to the formation of terpenylic acid, a major monomer observed in α-pinene/O3 SOA (Fig. S3) (Claeys et al., 2009) but here again a complex rearrangement has to be invoked (Scheme S6). In this context, there is evidence that unstable hetero-oligomers present in α-pinene/O3 SOA produce terpenylic acid upon heating (Lopez-Hilfiker et al., 2015). As already mentioned above, terpenylic acid was also found to be a major constituent of the 10 and 20 nm particles from α-pinene ozonolysis in a flow reactor (Winkler et al., 2012), which is likely formed by decomposition of unstable hetero-oligomeric species in the thermal desorption chemical ionization MS measurements (Hall and Johnston, 2012b).
Unambiguous mass spectrometric evidence has been obtained in this study for the linkage in the MW 368 (C19H28O7) hydroxypinonyl ester of cis-pinic acid, which is an abundant compound present in α-pinene/O3 SOA; more specifically, the MW 368 compound corresponds to an ester of cis-pinic acid where the carboxyl substituent of the dimethylcyclobutane ring and not the methylcarboxyl substituent is esterified with 7-hydroxypinonic acid. This linkage was already proposed in previous work for the MW 358 (C17H26O8) diaterpenylic ester of cis-pinic acid, another major compound present in α-pinene/O3 SOA (Yasmeen et al., 2010), but could be supported in the present study. Guided by the molecular structures of these stable esters, we propose a formation mechanism from highly oxygenated molecules in the gas phase that takes into account the detection of an unstable C19H28O11 HOM as a major species by CI-APi-TOF MS with nitrate clustering (Ehn et al., 2012, 2014; Zhao et al., 2013; Krapf et al., 2016). It is suggested that an acyl peroxy radical related to cis-pinic acid (RO2•) and an alkoxy radical related to 7- or 5-hydroxypinonic acid (R′O•) serve as key gas-phase radicals and combine according to a RO2• + R′O• → RO3R′ radical termination reaction. Subsequently, the unstable C19H28O11 dimeric HOM species decompose by the loss of oxygen or ketene from the inner part containing a labile linear trioxide bridge and the conversion of the unstable acyl hydroperoxide groups to carboxyl groups, resulting in stable esters with a molecular composition of C19H28O7 (MW 368) and C17H26O8 (MW 358), respectively. The proposed mechanism is supported by several observations reported in the literature, one of them being that cis-pinic acid is still generated after the consumption of α-pinene upon ozonolysis, suggesting its formation from an unstable HOM species (Zhang et al., 2015).
Further theoretical investigations are warranted to examine the proposed mechanism leading to the MW 368 and 358 esters present in α-pinene/O3 SOA. The mechanism is assumed to be energetically favorable as small stable molecules such as oxygen and ketene are expelled and a stable ester bridge is generated. The mechanism involves the combination of an acyl peroxy with an alkoxy radical according to a RO2• + R′O• → RO3R′ reaction and thus differs from that proposed to explain the formation of a MW 326 ester, where two acyl peroxy radicals related to cis-pinic acid combine according to a RO2• + RO2• → ROOR + O2 reaction (Zhang et al., 2015). We hypothesize that RO2• + R′O• → RO3R′ chemistry is at the underlying molecular basis of high-molecular-weight hetero-dimer formation in the gas phase upon α-pinene ozonolysis and may thus be of importance for new particle formation and growth in pristine forested environments.
The data are available upon request from the corresponding author but some data can be found in the Supplement.
The supplement related to this article is available online at: https://doi.org/10.5194/acp-18-8453-2018-supplement.
The authors declare that they have no conflict of interest.
Research at the University of Antwerp and TROPOS was supported by the
Belgian Federal Science Policy Office through the network project “Biogenic
Influence on Oxidants and Secondary Organic Aerosol: theoretical, laboratory
and modelling investigations (BIOSOA)”. Research at the University of
Antwerp was also supported by the Research Foundation – Flanders (FWO),
whereas research at TROPOS was also supported by the European Commission
through the EUROCHAMP-2 project (228335). We also would like to thank Anke Mutzel,
Torsten Berndt and Hartmut Herrmann from TROPOS for valuable
discussions on the proposed mechanism, and Olaf Böge for his help in the
preparation of the α-pinene/O3 SOA sample.
Edited by: Alexander Laskin
Reviewed by: three anonymous referees
Beck, M. and Hoffmann, T.: A detailed MSn study for the molecular identification of a dimer formed from oxidation of pinene, Atmos. Environ., 130, 120–126, 2016.
Bohr, F., Henon, E., Garcia, I., and Castro, M.: Theoretical study of the peroxy radicals RO2 self-reaction: Structures and stabilization energies of the intermediate RO4R for various R, Int. J. Quantum Chem., 75, 671–682, 1999.
Bonn, B., Schuster, G., and Moortgat, G.: Influence of water vapor on the process of new particle formation during monoterpene ozonolysis, J. Phys. Chem. A, 106, 2869–2881, 2002.
Claeys, M., Iinuma, Y., Szmigielski, R., Surratt, J. D., Blockhuys, F., Van Alsenoy, C., Böge, O., Sierau, B., Gómez-González, Y., Vermeylen, R., Van der Veken, P., Shahgholi, M., Chan, A. W. H., Herrmann, H., Seinfeld, J. H., and Maenhaut, W.: Terpenylic acid and related compounds from the oxidation of α-pinene: Implications for new particle formation and growth above forests, Environ. Sci. Technol., 43, 6976–6982, 2009.
Docherty, K. S., Wu, W., Lim, Y. B., and Ziemann, P. J.: Contributions of organic peroxides to secondary aerosol formed from reactions of monoterpenes with O3, Environ. Sci. Technol., 39, 4049–4059, 2005.
Ehn, M., Kleist, E., Junninen, H., Petäjä, T., Lönn, G., Schobesberger, S., Dal Maso, M., Trimborn, A., Kulmala, M., Worsnop, D. R., Wahner, A., Wildt, J., and Mentel, T. F.: Gas phase formation of extremely oxidized pinene reaction products in chamber and ambient air, Atmos. Chem. Phys., 12, 5113–5127, https://doi.org/10.5194/acp-12-5113-2012, 2012.
Ehn, M., Thornton, J. A., Kleist, E., Sipilä, M., Junninen, H., Pullinen, I., Springer, M., Rubach, F., Tillmann, R., Lee, B., Lopez-Hilfiker, F., Andres, S., Ismail-Hakki, A., Rissanen, M., Jokinen, T., Schobesberger, S., Kangasluoma, J., Kontkanen, J., Nieminen, T., Kurtén, T., Nielsen, L. B., Jørgensen, S., Kjaergaard, H. G., Canagaratna, M., Dal Maso, M., Berndt, T., Petäjä, T., Wahner, A., Kerminen, V.-M., Kulmala, M., Worsnop, D. R., Wildt, J., and Mentel, T. F.: A large source of low-volatility secondary organic aerosol, Nature, 506, 476–479, 2014.
Furniss, B. S., Hannaford, A. J., Smith, P. W. G., and Tatchell, A. R.: Vogel's Textbook of Practical Organic Chemistry, John Wiley, New York, 1989.
Gao, S., Ng, N. L., Keywood, M., Varutbangkul, V., Bahreini, R., Nenes, A., He, J., Yoo, K. Y., Beauchamp, J. L., Hodyss, R., Flagan, R. C., and Seinfeld, J. H.: Particle phase acidity and oligomer formation in secondary organic aerosol, Environ. Sci. Technol., 38, 6582–6589, 2004.
Gao, Y., Hall, W. A., and Johnston, M. V.: Molecular composition of monoterpene secondary organic aerosol at low mass loading, Environ. Sci. Technol., 44, 7897–7902, 2010.
Glasius, M., Duane, M., and Larsen, B. R.: Determination of polar terpene oxidation products in aerosols by liquid chromatography – ion trap mass spectrometry, J. Chrom. A, 833, 121–135, 1999.
Glasius, M., Lahaniati, M., Calogirou, A., Di Bella, D., Jensen, N. R., Hjorth, J., Kotzias, D., and Larsen, B. R.: Carboxylic acids in secondary aerosols from oxidation of cyclic monoterpenes by ozone, Environ. Sci. Technol., 34, 1001–1010, 2000.
Guenther, A. B., Jiang, X., Heald, C. L., Sakulyanontvittaya, T., Duhl, T., Emmons, L. K., and Wang, X.: The Model of Emissions of Gases and Aerosols from Nature version 2.1 (MEGAN2.1): an extended and updated framework for modeling biogenic emissions, Geosci. Model Dev., 5, 1471–1492, https://doi.org/10.5194/gmd-5-1471-2012, 2012.
Hall, W. A. and Johnston, M. V.: Oligomer formation pathways in secondary organic aerosol from MS and MS/MS measurements with high mass accuracy and resolving power, J. Am. Soc. Mass Spectrom., 23, 1097–1108, 2012a.
Hall, W. A. and Johnston, M. V.: The thermal-stability of oligomers in alpha-pinene secondary organic aerosol, Aerosol Sci. Tech., 46, 983–989, 2012b.
Hoffmann, T., Bandur, R., Marggraf, U., and Linscheid, M.: Molecular composition of organic aerosols formed in the alpha-pinene/O3 reaction: Implications for new particle formation processes, J. Geophys. Res., 103, 25569–25578, 1998.
Huang, W., Saathoff, H., Pajunoja, A., Shen, X., Naumann, K.-H., Wagner, R., Virtanen, A., Leisner, T., and Mohr, C.: α-Pinene secondary organic aerosol at low temperature: chemical composition and implications for particle viscosity, Atmos. Chem. Phys., 18, 2883–2898, https://doi.org/10.5194/acp-18-2883-2018, 2018.
Iinuma, Y., Böge, O., Gnauk, T., and Herrmann, H.: Aerosol chamber study of the α-pinene/O3 reaction: influence of particle acidity on aerosol yields and products, Atmos. Environ., 38, 761–773, 2004.
Iinuma, Y., Müller, C., Berndt, T., Böge, O., Claeys, M., and Herrmann, H.: Evidence for the existence of organosulfates from beta-pinene ozonolysis in ambient secondary organic aerosol, Environ. Sci. Technol., 41, 6678–6683, 2007.
Kahnt, A., Iinuma, Y., Mutzel, A., Böge, O., Claeys, M., and Herrmann, H.: Campholenic aldehyde ozonolysis: a mechanism leading to specific biogenic secondary organic aerosol constituents, Atmos. Chem. Phys., 14, 719–736, https://doi.org/10.5194/acp-14-719-2014, 2014.
Komai, T.: Water-soluble tertiary-alkyperoxy hydrogen phthalates, Patent, Jpn. Tokkyo Koho, 1971.
Kourtchev, I., Fuller, S. J., Giorio, C., Healy, R. M., Wilson, E., O'Connor, I., Wenger, J. C., McLeod, M., Aalto, J., Ruuskanen, T. M., Maenhaut, W., Jones, R., Venables, D. S., Sodeau, J. R., Kulmala, M., and Kalberer, M.: Molecular composition of biogenic secondary organic aerosols using ultrahigh-resolution mass spectrometry: comparing laboratory and field studies, Atmos. Chem. Phys., 14, 2155–2167, https://doi.org/10.5194/acp-14-2155-2014, 2014.
Kourtchev, I., Doussin, J.-F., Giorio, C., Mahon, B., Wilson, E. M., Maurin, N., Pangui, E., Venables, D. S., Wenger, J. C., and Kalberer, M.: Molecular composition of fresh and aged secondary organic aerosol from a mixture of biogenic volatile compounds: a high-resolution mass spectrometry study, Atmos. Chem. Phys., 15, 5683–5695, https://doi.org/10.5194/acp-15-5683-2015, 2015.
Kourtchev, I., Giorio, C., Manninen, A., Wilson, E., Mahon, B., Aalto, J., Kajos, M., Venable, D., Ruuskanen, T., Levula, J., Loponen, M., Connors, S., Harris, N., Zhao, D., Kiendler-Scharr, A., Mentel, T., Rudich, Y., Hallquist, M., Doussin, J.-F., Maenhaut, W., Bäck, J., Petäjä, T., Wenger, J., Kulmala, M., and Kalberer, M.: Enhanced volatile organic compounds emissions and organic aerosol mass increase the oligomer content of atmospheric aerosols, Sci. Rep., 6, 35038, https://doi.org/10.103/srep35038, 2016.
Krapf, M., El Haddad, I., Bruns, E. A., Molteni, U., Daellenbach, K. R., Prévôt, A. S. H., Baltensperger, U., and Dommen, J.: Labile peroxides in secondary organic aerosol, Chemistry, 1, 603–616, 2016.
Kristensen, K., Enggrob, K. L., King, S. M., Worton, D. R., Platt, S. M., Mortensen, R., Rosenoern, T., Surratt, J. D., Bilde, M., Goldstein, A. H., and Glasius, M.: Formation and occurrence of dimer esters of pinene oxidation products in atmospheric aerosols, Atmos. Chem. Phys., 13, 3763–3776, https://doi.org/10.5194/acp-13-3763-2013, 2013.
Kristensen, K., Cui, T., Zhang, H., Gold, A., Glasius, M., and Surratt, J. D.: Dimers in α-pinene secondary organic aerosol: effect of hydroxyl radical, ozone, relative humidity and aerosol acidity, Atmos. Chem. Phys., 14, 4201–4218, https://doi.org/10.5194/acp-14-4201-2014, 2014.
Kristensen, K., Watne, Å. K., Hammes, J., Lutz, A., Petäjä, T., Hallquist, M., Bilde, M., and Glasius, M.: High-molecular weight dimer esters are major products in aerosols from α-pinene ozonolysis and the boreal forest, Environ. Sci. Technol. Lett., 3, 280–285, 2016.
Larsen, B. R., Di Bella, D., Glasius, M., Winterhalter, R., Jensen, N. R., and Hjorth, J.: Gas-phase OH oxidation of monoterpenes: gaseous and particulate products, J. Atmos. Chem., 38, 231–276, 2001.
Lee, S. and Kamens, R. M.: Particle nucleation from the reaction of α-pinene and O3, Atmos. Environ., 39, 6822–6832, 2005.
Lopez-Hilfiker, F. D., Mohr, C., Ehn, M., Rubach, F., Kleist, E., Wildt, J., Mentel, T. F., Carrasquillo, A. J., Daumit, K. E., Hunter, J. F., Kroll, J. H., Worsnop, D. R., and Thornton, J. A.: Phase partitioning and volatility of secondary organic aerosol components formed from α-pinene ozonolysis and OH oxidation: the importance of accretion products and other low volatility compounds, Atmos. Chem. Phys., 15, 7765–7776, https://doi.org/10.5194/acp-15-7765-2015, 2015.
Müller, L., Reinnig, M.-C., Warnke, J., and Hoffmann, T.: Unambiguous identification of esters as oligomers in secondary organic aerosol formed from cyclohexene and cyclohexene/a-pinene ozonolysis, Atmos. Chem. Phys., 8, 1423–1433, https://doi.org/10.5194/acp-8-1423-2008, 2008.
Mutzel, A., Poulain, L., Berndt, T., Iinuma, Y., Rodigast, M., Böge, O., Richters, S., Spindler, G., Sipilä, M., Jokinen, T., Kulmala, M., and Herrmann, H.: Highly oxidized multifunctional organic compounds observed in tropospheric particles: A field and laboratory study, Environ. Sci. Technol., 49, 7754–7761, 2015.
Nozière, B., Kalberer, M., Claeys, M., Allan, J., D'Anna, B., Decesari, S., Finessi, E., Glasius, M., Grgíc, I., Hamilton, J. F., Hoffmann, T., Iinuma, Y., Jaoui, M., Kahnt, A., Kampf, C. J., Kourtchev, I., Maenhaut, W., Marsden, N., Saarikoski, S., Schnelle-Kreis, J., Surratt, J. D., Szidat, S., Szmigielski, R., and Wisthaler, A.: The molecular identification of organic compounds in the atmosphere: State of the art and challenges, Chem. Rev., 115, 3919–3983, 2015.
Tolocka, M. P., Jang, M., Ginter, J. M., Cox, F. J., Kamens, R. M., and Johnston, M. V.: Formation of oligomers in secondary organic aerosol, Environ. Sci. Technol., 38, 1428–1434, 2004.
Wildt, J., Mentel, T. F., Kiendler-Scharr, A., Hoffmann, T., Andres, S., Ehn, M., Kleist, E., Müsgen, P., Rohrer, F., Rudich, Y., Springer, M., Tillmann, R., and Wahner, A.: Suppression of new particle formation from monoterpene oxidation by NOx, Atmos. Chem. Phys., 14, 2789–2804, https://doi.org/10.5194/acp-14-2789-2014, 2014.
Winkler, P. M., Ortega, J., Karl, T., Cappellin, L., Friedli, H. R., Barsanti, K., McMurry, P. H., and Smith, J. N.: Identification of the biogenic compounds responsible for size-dependent nanoparticle growth, Geophys. Res. Lett., 39, L20815, https://doi.org/10.1029/2012GL053253, 2012.
Winterhalter, R., Van Dingenen, R., Larsen, B. R., Jensen, N. R., and Hjorth, J.: LC-MS analysis of aerosol particles from the oxidation of α-pinene by ozone and OH-radicals, Atmos. Chem. Phys. Discuss., 3, 1–39, https://doi.org/10.5194/acpd-3-1-2003, 2003.
Witkowski, B. and Gierczak, T.: Early stage composition of SOA produced by α-pinene/ozone reaction: α-Acyloxyhydroperoxy aldehydes and acidic dimers, Atmos. Environ., 95, 59–70, 2014.
Yasmeen, F., Vermeylen, R., Szmigielski, R., Iinuma, Y., Böge, O., Herrmann, H., Maenhaut, W., and Claeys, M.: Terpenylic acid and related compounds: precursors for dimers in secondary organic aerosol from the ozonolysis of α- and β-pinene, Atmos. Chem. Phys., 10, 9383–9392, https://doi.org/10.5194/acp-10-9383-2010, 2010.
Yasmeen, F., Szmigielski, R., Vermeylen, R., Gómez-González, Y., Surratt, J. D., Chan, A. W. H., Seinfeld, J. H., Maenhaut, W., and Claeys, M.: Mass spectrometric characterization of isomeric terpenoic acids from the oxidation of α-pinene, β-pinene, d-limonene, and Δ3-carene in fine forest aerosol, J. Mass Spectrom., 46, 425–442, 2011.
Yasmeen, F., Vermeylen, R., Maurin, N., Perraudin, E., Doussin, J.-F., and Claeys, M.: Characterisation of tracers for aging of α-pinene secondary organic aerosol using liquid chromatography/negative ion electrospray ionisation mass spectrometry, Environ. Chem., 9, 236–246, 2012.
Yu, J., Cocker III, D. R., Griffin, R. J., Flagan, R. C., and Seinfeld, J. H.: Gas-phase ozone oxidation of monoterpenes: Gaseous and particulate products, J. Atmos. Chem., 34, 207–258, 1999.
Zhang, X., McVay, R. C., Huang, D. D., Dalleska, N. F., Aumont, B., Flagan, R. C., and Seinfeld, J. H.: Formation and evolution of molecular products in α-pinene secondary organic aerosol, P. Natl. Acad. Sci. USA, 112, 14168–14173, 2015.
Zhang, X., Lambe, A. T., Upshur, M. A., Brooks, W. A., Gray Beì, A., Thomson, R. J., Geiger, F. M., Surratt, J. D., Zhang, Z., Gold, A., Graf, S., Cubison, M. J., Groessl, M., Jayne, J. T., Worsnop, D. R., and Canagaratna, M. R.: Highly oxygenated multifunctional compounds in α-pinene secondary organic aerosol, Environ. Sci. Technol., 51, 5932–5940, 2017.
Zhao, J., Ortega, J., Chen, M., McMurry, P. H., and Smith, J. N.: Dependence of particle nucleation and growth on high-molecular-weight gas-phase products during ozonolysis of α-pinene, Atmos. Chem. Phys., 13, 7631–7644, https://doi.org/10.5194/acp-13-7631-2013, 2013.
Ziemann, P. J.: Evidence for low-volatility diacyl peroxides as a nucleating agent and major component of aerosol formed from reactions of ozone with cyclohexene and homologous compounds, J. Phys. Chem. A, 106, 4390–4402, 2002.