the Creative Commons Attribution 4.0 License.
the Creative Commons Attribution 4.0 License.
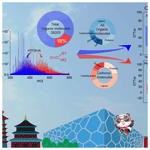
Critical contribution of chemically diverse carbonyl molecules to the oxidative potential of atmospheric aerosols
Feifei Li
Shanshan Tang
Jitao Lv
Shiyang Yu
Xu Sun
Dong Cao
Yawei Wang
Guibin Jiang
Carbonyls have an important effect on atmospheric chemistry and human health because of their high electrophilicity. Here, high-throughput screening of carbonyl molecules in complex aerosol samples was achieved by combining targeted derivatization with non-targeted analysis using Fourier transform ion cyclotron resonance mass spectrometry (FT-ICR MS). Results showed that water-soluble organic matter (WSOM) in PM2.5 contains a large variety of carbonyls (5147 in total), accounting for 17.6 % of all identified organic molecules. Compared with non-carbonyl molecules, carbonyl molecules are more abundant in winter than in summer and have unique molecular composition and chemical parameters. For the first time, a significant positive correlation was found between the abundance of carbonyl molecules and the dithiothreitol (DTT) activities of WSOM, and the elimination of the carbonyl group remarkably reduced the DTT activities, highlighting the pivotal role of carbonyls in determining the oxidative potential (OP) of organic aerosol. Among various molecules, oxidized aromatic compounds containing the carbonyl group produced in winter contributed more to the enhancement of DTT activity, which could be used as potential markers of atmospheric oxidative stress. This study improves our understanding of the chemical diversity and environmental health effects of atmospheric carbonyls, emphasizing the need for targeted strategies to mitigate the health risks associated with carbonyl-rich aerosols.
- Article
(3166 KB) - Full-text XML
-
Supplement
(2735 KB) - BibTeX
- EndNote
Atmospheric carbonyls are important components of atmospheric organic compounds, forming not only from primary sources such as vehicle emissions, coal and biomass fuel combustion, and industrial emissions (Q. Liu et al., 2022) but also through secondary reactions between atmospheric oxidants and volatile organic compounds (VOCs) emitted from anthropogenic and biological sources (Claeys et al., 2004; Kalberer et al., 2004). Meanwhile, carbonyls are also involved in the formation of strong oxidative pollutants such as O3 and peroxyacetyl nitrate (Q. Liu et al., 2022), as well as the formation of secondary organic aerosols (Chen et al., 2023), thus playing a central role in atmospheric chemistry (Lary and Shallcross, 2000). Moreover, carbonyls are reactive and electrophilic compounds, which can react with nucleophilic sites in biomacromolecules, leading to biomacromolecular dysfunction and eventually cytotoxicity (Han et al., 2020; Kumagai et al., 2002; Rappaport et al., 2012).
Due to the key role of carbonyls in atmospheric chemical processes (Ji et al., 2020; Powelson et al., 2014) and their effects on human health (Bora and Shankarrao Adole, 2021; Li et al., 2015), atmospheric carbonyls have received special attention (Chen et al., 2023; Yang et al., 2023; Ye et al., 2023). Currently, high-performance liquid chromatography and ultraviolet–visible detection (HPLC-UV/Vis) (Qian et al., 2019; Shen et al., 2018; Yu et al., 2023) and gas chromatography–mass spectrometry (GC-MS) (Ho and Yu, 2004) coupled with carbonyl chemical derivatization are the dominant methods for the analysis of atmospheric carbonyls. These methods mainly focus on atmospheric VOCs such as formaldehyde, acetaldehyde, acetone, keto acids, glyoxal, and methylglyoxal (Q. Liu et al., 2022). However, a growing number of studies suggest that the concentration of carbonyls in the particulate phase has been grossly underestimated, and plenty of water-soluble and semi-volatile carbonyls with high molecular weight are also present in the particulate phase (Shen et al., 2018; Tang et al., 2022; Wang et al., 2022). Recently, Xu et al. (2023) developed an enhanced method employing ultra-high-performance liquid chromatography and electrospray ionization tandem mass spectrometry (UHPLC-MS/MS) to simultaneously quantify 47 carbonyl compounds, showing that carbonyl compounds missed by the traditional method have a critical contribution to atmospheric photochemical pollution. Until now, only a small number of carbonyls have been detected by target analysis; thus a comprehensive identification of them in the atmosphere is urgent. In fact, this remains challenging due to the diversity and complexity of atmospheric chemical compositions.
In the past decade, non-targeted analysis methods based on ultra-high resolution mass spectrometry (UHRMS), such as Fourier transform ion cyclotron resonance mass spectrometry (FT-ICR-MS) and Orbitrap mass spectrometry, have been widely used for molecular characterization of soluble components in atmospheric aerosols from different sources (Bianco et al., 2018; Ma et al., 2022; Song et al., 2019, 2022; Yue et al., 2022; Zeng et al., 2020). Based on non-targeted analysis, our understanding of the molecular composition and chemical diversity of atmospheric organic aerosols has greatly improved (Jiang et al., 2021; F. Li et al., 2023; Tang et al., 2022). However, molecular formulas in organic matter (OM) can only be obtained by direct UHRMS detection, and the assessment of molecular characteristic groups is empirically dependent on their elementary composition. Chemical derivatization is a common technique used in targeted analysis for carbonyl compounds (Baluha et al., 2013; Liu et al., 2020). Recently, Wang et al. (2022) employed Girard's reagent T derivatization with +ESI Orbitrap MS analysis to detect the carbonyls in atmospheric fine particulate matter (PM2.5). However, all molecules containing three N atoms were assigned to derivative products, which may overestimate the contribution of carbonyls (Liu et al., 2020). In order to accurately and rapidly identify carbonyls from natural organic matter, we have recently developed a novel set of detection methods and screening procedures based on O-(2,3,4,5,6-pentafluorobenzyl)hydroxylamine (PFBHA) derivatization and ESI FT-ICR MS analysis (Yu et al., 2024). This method provides the opportunity to reveal the molecular composition and chemical diversity of atmospheric carbonyl molecules.
Oxidative potential (OP) is one of the most widely used indicators to assess the atmospheric health hazards of PM2.5. Previous studies have reported the important role of water-soluble organic matter (WSOM), such as quinones and humic-like substances (HULIS), in determining the OP of atmospheric aerosols (Jiang and Jang, 2018; Verma et al., 2012). Due to the chemical diversity of WSOM, it is still unclear which fractions contributed to the OP of atmospheric aerosols. Carbonyls are known as major electrophilic compounds in atmospheric WSOM (Graber and Rudich, 2006; Huang et al., 2020; Huo et al., 2021). Previous studies have reported the correlation between the theoretical electrophilicity of model carbonyl compounds and dithiothreitol (DTT) activities (J. Y. Chen et al., 2019). Therefore, it is expected that atmospheric carbonyls may have an important contribution to the OP of WSOM in PM2.5. However, until now, the relationship between carbonyls and DTT activities in actual atmospheric samples has not been addressed, mainly due to methodological limitations.
For the above reasons, in this study, we selected three stations in downtown, suburban, and mountainous sites of Beijing to collect atmospheric PM2.5 samples for typical pollution processes in summer and winter. The carbonyl molecules and non-carbonyl molecules in WSOM extracted from PM2.5 samples were studied by PFBHA derivatization and ESI FT-ICR MS analysis. DTT assay was employed to measure the OP of the collected samples, and the contribution of carbonyls to the OP of WSOM extracted from real atmospheric samples was investigated.
2.1 PM2.5 sample collection and extraction
PM2.5 samples were collected in 2022 during three periods: winter (19 January to 3 February), the Winter Olympics (4 February to 20 February), and summer (10 July to 27 July). The sampling sites were the monitoring sites set up by the Beijing Urban Ecosystem Research Station, including a downtown site at the Beijing Teaching Botanical Garden (39°88′ N, 116°44′ E); a suburban site at Caiyu, Daxing District (39°67′ N, 116°71′ E); and a mountainous site at Mangshan National Forest Park with an altitude of about 659 m (40°28′ N, 116°29′ E). Samples were collected on pre-baked (550 °C, 6 h) quartz filters (Pallflex, 90 mm diameter) using medium-volume samplers with PM2.5 cutting heads (Qingdao Laoying Corp, Qingdao, China) at a 24 h sampling period and then stored in a refrigerator at −20 °C. Particulate matter concentrations and meteorological data during the sampling period were provided by the Beijing Urban Ecosystem Research Station. Detailed sampling information is recorded in Table S1 in the Supplement.
The water-soluble fractions were extracted from PM2.5 samples (each quartz filter membrane) twice with ultrapure water (10 mL) and sonicated for 30 min each time. Then, the solution was filtered through the polytetrafluoroethylene syringe filter (PTFE; 0.22 µm, Jinteng, Tianjin, China). The filtrate was further purified and desalted by solid phase extraction (SPE; Varian Bond Elute PPL cartridges) to obtain WSOM (F. Li et al., 2023). The quartz filters after water extraction were dried and extracted twice (30 min each) with 5 mL of methanol (MeOH) by ultrasonication. Since inorganic salts and metals were removed during ultrapure water extraction, the MeOH extracted fraction is considered water-insoluble organic matter (WISOM) (Yue et al., 2022). The same extraction operation was performed for the travel blank.
Detailed information on all chemicals used in the study is provided in Sect. S1 in the Supplement, and more details about WSOM and WISOM extraction are provided in Sect. S2.
2.2 Derivatization of WSOM by PFBHA
Each WSOM sample was divided into three parts. One part was dried with N2 and redissolved in ultrapure water for total organic carbon (TOC) analysis (Shimadzu 5000-A, Germany). Another part was dried with N2 and redissolved in 50:50 MeOHwater () for FT-ICR MS analysis. The third part was used for PFBHA derivatization before FT-ICR MS analysis. Briefly, PFBHA (300 mg L−1) and WSOM samples (100 mg L−1) were dissolved in a mixture of acetonitrile (ACN) and water (70 % and 30 %, ) and then reacted in a shaker for 1 h and then incubated in a water bath at 50 °C for 12 h. Subsequently, the samples were blown dry with N2, and the solvent was replaced with ACN and water (50 % and 50 %, ) and stored at −4 °C. Here, ACN was used as a solvent to avoid the formation of potential solvent artifacts by reacting MeOH with conjugated carbonyl groups during the derivatization reaction (Chen et al., 2022). Furthermore, six model carbonyl molecules with different structures (including quinones, aldehydes, ketones, and acids; detailed information is provided in Sect. S1) were employed to test the efficiencies of finding carbonyl compounds applying the method used in this study. Six model compounds and their derivatized products can be detected in treatments, and derivatized products displayed considerable or higher SN than original model carbonyls, indicating high derivatization and detection efficiencies of derivatized products using our method (Fig. S1 in the Supplement).
2.3 ESI-FT-ICR MS analysis and carbonyl molecule identification
WSOM samples before and after derivatization were both detected using ESI-FT-ICR MS with the negative ion mode (15.0 T SolariX, Bruker, Billerica, MA, USA). The detection mass range was firstly set to 99–2000; considering the mass range of all original and derived samples and the calibration range of sodium formate internal standard, the collected mass range was finally set to 122–928. Molecular formulas assigned to mass peaks with a signal-to-noise ratio (SN) > 4 are considered valid. Detailed analysis methods are described in the Supplement (Sect. S3).
Molecular formula assignment in the original WSOM was conducted following our previous study (Tang et al., 2022; F. Li et al., 2023). For carbonyl molecule identification, a new automatic screening procedure developed in our group was employed (Yu et al., 2024), and the detailed screening process is provided in Sect. S4. Briefly, the mass difference of the reactant–product ion pair formed by the PFBHA derivatization should be 195.01074 (C7H2NF5) or its multiples (C14H4N2F10, C21H6N3F15) according to the reaction equation (Fig. S2). The molecular formulas of carbonyls can be identified if the above mass difference was observed in the sample before and after PFBHA derivatization. As a result, carbonyl and non-carbonyl molecules in the original WSOM can be screened separately. Additionally, the molecular formulas of the control group (blank membrane samples) were subtracted for further analysis.
Molecular parameters such as double-bond equivalence (DBE), modified aromaticity index (AImod), the nominal oxidation state of carbon (NOSC) (Wang et al., 2021), and the average carbon oxidation state () (Kroll et al., 2011) of the identified molecular formulas were calculated as documented in Sect. S3. The magnitude-weighted average parameters (e.g., DBEw) are the average of the compositional value in all formulas weighted by the relative intensity of each formula (Lv et al., 2016). In the van Krevelen (VK) diagrams, all the detected molecular formulas were divided into five categories according to their degree of aromatization and alkylation (Jiang et al., 2022; Su et al., 2021), which are polycyclic aromatic-like molecules (AImod>0.67), highly aromatic-like molecules (), highly unsaturated-like molecules (AImod≤0.5 and ), unsaturated aliphatic-like molecules (), and saturated-like molecules ( or ).
2.4 DTT activity assay
The OP was assessed based on the DTT activity assay (Dou et al., 2015; Lin and Yu, 2020; Wang et al., 2018). The DTT activity of the whole PM2.5 samples was first assessed. The travel blanks and PM2.5 samples (8 cm2) were first ultrasonicated with 8 mL diethylenetriaminepentaacetic acid (DTPA; 1 mM, dissolved in 0.1 M phosphate buffer saline (PBS)) for 1 h, and then the residual samples were added with 8 mL methanol for 1 h. After filtering through 0.22 µm PTFE membrane, PBS extract and methanol extract were each taken in a volume of 475 µL, mixed well, and then incubated with 50 µL DTT (2 mM, dissolved in 0.1 M PBS) in a cell incubator (37 °C) for 0, 30, 60, 90, and 120 min. Finally, 100 µL 5,5-dithiobis(2-nitrobenzoic acid) (DTNB, 1 mM, dissolved in 0.1 M PBS) was added and then the absorbance at 412 nm was measured by a microplate reader (SpectraMax iD3, Molecular Devices, USA).
For the WSOM and WISOM samples, 50 µL of the samples was dissolved in 900 µL of DTPA (1 mM, dissolved in 0.1 M PBS) and then incubated with 50 µL DTT and measured as described above. The whole experiment was conducted away from light, and the consumption of DTT was controlled within 90 %. Three repetitions were set for each sample, and the experimental blank was deducted. As shown in Fig. S3, the DTT concentrations varied linearly with time in this experiment, indicating a zero-level kinetic reaction, as reported in other studies (Q. Chen et al., 2019; Ma et al., 2018). As DTPA was added in this experiment to inhibit the consumption of DTT by metal ions, the measured values only reflect the response of OM to DTT (Lin and Yu, 2011). The volume-normalized DTT consumption rate (DTTv), the mass-normalized DTT consumption rate (DTTm), and the TOC-normalized DTT consumption rate (DTTOC) are calculated by the following equations.
where RDTT (%) represents the ratio of DTT consumed in reaction time. nDTT is the amount of initial DTT, t is the reaction time, and air volume (m3) is the sampling volume corresponding to the extracted PM2.5 samples.
2.5 Reduction of the carbonyl group by sodium borohydride (NaBH4)
NaBH4 has been reported to irreversibly reduce ketone, aldehyde, and quinone to alcohols (Baluha et al., 2013; Phillips and Smith, 2015). Therefore, carbonyl molecules in samples can be reduced by NaBH4 treatment (Phillips and Smith, 2014; Baluha et al., 2013). Here, a standard humic substance, Suwannee River natural organic matter (SRNOM), obtained from the International Humic Substances Society (IHSS), and a diesel soot sample collected from heavy-duty diesel vehicles were used to study the changes of carbonyls and DTT activity before and after NaBH4 reduction. WSOM of diesel soot (WSOMds) was extracted with ultrapure water twice (10 mL, 30 min each) by ultrasonic extraction and filtered through the 0.22 µm filter membrane. Then the SRNOM and WSOMds were treated by NaBH4 according to previous studies with minor modifications (Phillips and Smith, 2014, 2015). Briefly, the samples were deoxidized with N2 for 15 min, then 10 times the sample mass of NaBH4 was added, and the reaction was performed in the dark for 12 h; the specific steps are recorded in Sect. S5 and Fig. S4. Afterward, the inorganic salts in the treated SRNOM and diesel soot samples, including unreacted NaBH4 and the boron salts generated after the reaction, were removed by SPE (Sect. S2) (Lv et al., 2016). Then, the desalted samples of SRNOM and diesel soot were further subjected to carbonyl derivatization, FT-ICR MS analysis, and DTT activity assay.
2.6 Statistical analysis
The Kruskal–Wallis tests in IBM SPSS Statistics 25 were used to perform the significance test of difference for more than two groups of samples. The pro-oxidative carbonyls were screened stepwise by Spearman correlation analysis, correcting false discovery rates (FDRs), and partial least-squares regression (PLSR) modeling (Zhang et al., 2023). Spearman correlation tests and FDR correction were performed using the packages “rcorr” and “fdrtool” in R v.3.6.2, respectively. The PLSR model, principal component analysis (PCA) model, and orthogonal partial least-squares discriminant analysis (OPLS-DA) model (Papazian et al., 2022) were performed and validated by SIMCA 14.1 software. Detailed description and model validation are recorded in Sect. S6 and Figs. S5–S7.
3.1 The organic molecular composition of WSOM in PM2.5
According to the daily average PM2.5 concentrations at each site during the sampling periods (Fig. S8), air quality is worse in winter (3–146 µg m−3) than in summer (2–64 µg m−3). An obvious improvement in PM 2.5 pollution was observed during the Winter Olympics (5–43 µg m−3), mainly due to the reduction of transportation and industrial emissions (Y. Liu et al., 2022). Based on the PM2.5 concentration levels (>80 %, 40 %–60 %, and <20 %) in three periods, a total of 27 representative PM2.5 samples (Table S1) were selected to further investigate the composition of organic molecules in WSOM by ESI FT-ICR MS. The number of identified molecules in a single sample ranged from 7369 to 10 401 (Table S2). It seems that even in very clean air, WSOM extracted from PM2.5 contained more than 7000 molecules. In total, 29 203 unique molecules were identified in all samples, of which 5147 were carbonyl molecules and 24 056 were non-carbonyl molecules (Fig. 1a). The number of carbonyls ranged from 11 % to 22 % in each sample, whereas the normalized molecular intensity of these carbonyls was higher (20 %–35 %), likely due to the higher concentration levels of carbonyl molecules than non-carbonyl molecules. Significantly more carbonyl molecules were detected in winter than in summer (Kruskal–Wallis tests, p<0.05), while non-carbonyl molecules detected in winter and summer showed no significant difference (Fig. 1b), probably because more carbonyl compounds tended to be released into the gas phase in summer due to higher temperature (Qian et al., 2019; Shen et al., 2018). At the same time, the fuel combustion emissions in winter may release more carbonyl molecules into the air (Fu et al., 2008).
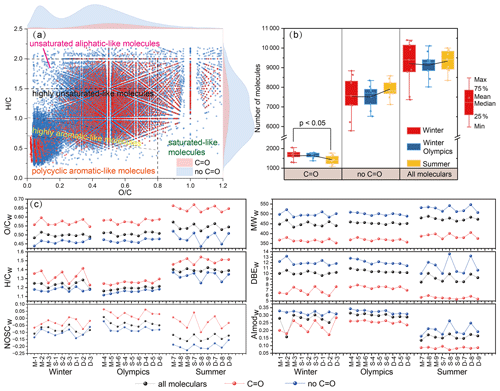
Figure 1The molecular composition of WSOM in PM2.5 samples was analyzed by FT-ICR MS. (a) Van Krevelen (VK) diagram of carbonyl (C=O) and non-carbonyl (no C=O) molecules in all samples. (b) The distribution of total carbonyl and non-carbonyl molecules was detected in winter, the Winter Olympics, and summer. (c) The magnitude-weighted average of and , the nominal oxidation state of carbon (NOSC), molecular weight (MW), double-bond equivalence (DBE), and modified aromaticity index (AImod) of the samples. PM2.5 samples are shown as from the mountainous (M), suburban (S), and downtown site (D) in the winter (1–3), Winter Olympics (4–6), and summer (7–9) samples.
The magnitude-weighted average parameters such as molecular weight and elemental ratio of organic molecules in each sample are summarized in Fig. 1c. Compared to non-carbonyl molecules, carbonyl molecules generally have a lower molecular weight (MWw=374 ± 14), DBEw (6.63 ± 0.89), and AImodw (0.19 ± 0.08) values but higher (0.60 ± 0.04), (1.36 ± 0.11), and NOSCw (−0.01 ± 0.04), indicating that the carbonyl group was mainly present in smaller organic molecules with low aromaticity and a high oxidation degree.
For all detected molecular formulas of five categories, the proportion of molecules in each group was estimated based on the molecular numbers (Figs. S9 and S10). Highly unsaturated-like and unsaturated aliphatic-like molecules are the most dominant components in both carbonyl and non-carbonyl molecules. Compared to non-carbonyl molecules, carbonyl molecules showed a significant decrease in polycyclic aromatic-like and highly aromatic-like molecules (p<0.01) but a significant increase in highly unsaturated-like, unsaturated aliphatic-like, and saturated-like molecules (p<0.001).
Additionally, molecular formulas can be categorized into four groups based on elemental composition and their proportions in carbonyls and non-carbonyls, and all molecules were estimated based on the molecular numbers (Figs. S11 and S12). On average, CHON is the predominant element in both carbonyl and non-carbonyl molecules, but the percentage of CHON-containing carbonyls (38.5 % ± 5.2 %) is significantly lower than that of non-carbonyls (49.7 % ± 4.2 %) (p<0.001). Conversely, the percentages of CHO-containing carbonyls (27.7 % ± 5.0 %) are significantly higher than those of non-carbonyls (24.0 % ± 3.4 %) (p<0.01). In particular, the percentages of sulfur-containing molecules (CHOS) are significantly higher in carbonyls (22.1 % ± 4.5 %) compared to non-carbonyls (11.8 % ± 1.6 %) (p<0.05), as well as in CHONS, indicating a substantial contribution of carbonyl groups to sulfur-containing organic compounds.
3.2 Contributions of different organic fractions to the oxidative potential of PM2.5
DTT activity is a commonly used cell-free assay to detect the OP of atmospheric samples (Bates et al., 2019; He and Zhang, 2022), wherein DTTv can reflect the DTT activity caused by PM2.5 exposure, and DTTm can reflect the intrinsic DTT activity of the contaminants in PM2.5 (Ma et al., 2018; Q. Chen et al., 2019; Daellenbach et al., 2020). Figure 2a illustrates the variation of OP levels of PM2.5 samples. The average DTTv of PM2.5 samples in this study was 164 ± 171 , much lower than that reported for Tianjin (6.8 ± 3.4 ) (Liu et al., 2018) but on the same order of magnitude as the other Chinese cities (Shanghai, 0.13 ; Xi'an, 0.51 ; Hangzhou, 0.62 ) (Lyu et al., 2018; Q. Chen et al., 2019; Wang et al., 2019).
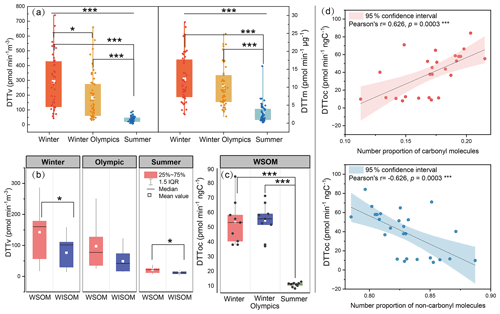
Figure 2DTT consumption rates of different fractions in PM2.5 and its correlation with organic molecules over Beijing in the winter, Winter Olympics, and summer (Kruskal–Wallis tests; differences between groups were considered statistically significant when p<0.05, with marked by *, p<0.01 marked by **, and p<0.001 marked by ***). (a) Volume-normalized DTT consumption rate (DTTv) and mass-normalized DTT consumption rate (DTTm) of organic matter in PM2.5 of the sampling period. (b) DTTv of water-soluble organic matter (WSOM) and water-insoluble organic matter (WISOM) for representative samples. (c) DTT consumption rate of WSOM normalized by TOC (DTTOC). (d) Relationship between the number proportion of carbonyl and non-carbonyl molecules and oxidative potential of WSOM.
Samples collected in summer displayed the lowest DTTv (8–90 ), followed by the Winter Olympics (32–660 ) and winter (43–741 ). Both DTTv and DTTm were significantly higher in winter than in summer (p<0.001), indicating that both of the contributions of total organic aerosol and organic aerosol per unit mass to the OP are higher in winter, and therefore the overall OP risk is higher in winter. Although the PM2.5 pollution was significantly improved during the Winter Olympics, only a slight reduction in DTTv and DTTm values was observed in the Winter Olympics compared to other days in winter. During the Winter Olympics, transportation and industrial emissions have been successfully controlled (Y. Liu et al., 2022), but livelihood-related heating and household emissions remain unrestricted. In particular, this study observed a significantly lower DTTv level in 2022 compared to that reported in 2016 (1.9 ± 0.3 ) and 2017 (5.8 ± 7.1 ) for Beijing PM2.5 samples (Campbell et al., 2021; Yu et al., 2022). This may be attributed to China's implementation of the “Three-Year Action Plan (2018–2020)”, an energy policy that gradually shifted the use of coal to natural gas and electricity for cooking and heating in rural areas, leading to improved air quality for Beijing and its surroundings (Du et al., 2022; Y. Li et al., 2023). The results indicated that under the current air quality level in Beijing, livelihood-related emissions have an important contribution to the atmospheric OP; thus the potential of regulating industrial and transportation emissions to improve the atmospheric OP is less valid than energy upgrading. Hence, winter heating and meteorological conditions may be the main influences on the OP of PM2.5. As shown in Fig. S13 in the Supplement, no significant difference in DTTv was observed between regions. However, the DTTm of suburban sites is significantly higher than that of urban sites in winter, and the opposite is true in summer (p<0.05). This is likely due to the differences in aerosol sources between different seasons (Campbell et al., 2021; Steimer et al., 2020). In winter, fossil fuel and biomass burning constitute a major local source of PM2.5 in suburban sites, while this special combustion source aerosol in suburban sites was eliminated in summer. Detailed differences in carbonyl molecules in organic aerosol (OA) between winter and summer will be discussed later.
The DTT activities of WSOM and WISOM fractions for each sample were detected as well; results showed that the contribution of WSOM to DTTv (51 %–84 %) was much higher than that of WISOM (Fig. 2b), indicating that water-extractable compounds have a greater contribution to the OP of PM2.5. Meanwhile, we noticed that the seasonal variation of carbonyl molecules (Fig. 1b) was consistent with the DTTOC (Fig. 2c), which was higher in winter than in summer. Further, the correlation between the DTTOC and organic molecules was investigated (Fig. 2d). Although an insignificant correlation was observed between the normalized molecular intensities of all carbonyls and DTTOC, a significant positive correlation was observed when S-containing molecules were excluded (Fig. S14) because of unreasonably high intensities of sulfur-containing molecules in the samples due to their extremely high ionization efficiencies (Xie et al., 2022). In addition, both the number and the number proportion of carbonyl molecules were positively correlated with DTTOC (Pearson's r=0.55, p<0.01 and Pearson's r=0.63, p<0.001), whereas an insignificant or negative correlation was observed between those of non-carbonyls and DTTOC (Pearson's , p=0.185 and Pearson's , p<0.001) (Figs. 2d and S14). These results suggested carbonyl molecules played a more important role in OP generation than non-carbonyl molecules. Using simulated organic aerosol samples or model compounds, the contributions of carbonyl compounds with different structures to the oxidative potential of OA by a variety of cellular and acellular assays have been studied (Bates et al., 2019; J. Y. Chen et al., 2019; Han et al., 2020; Jiang and Jang, 2018; Tuet et al., 2017; Wang et al., 2018). Conjugated carbonyls, such as benzaldehyde, α,β-unsaturated carbonyl, aromatic aldehydes, and polycyclic aromatic hydrocarbon o-quinones, mainly belong to polycyclic aromatic-like carbonyl molecules and highly aromatic-like carbonyl molecules according to VK diagrams and are found to have high oxidative potential and may cause the toxicities of OA. Here, we observed the relationships between carbonyl molecules and DTT activities of real aerosol samples for the first time, providing further evidence for the health hazards of carbonyl molecules in atmospheric environments.
3.3 Verification of the contribution of carbonyl molecules to the oxidative potential of organic aerosol
NaBH4 is a widely used reagent to irreversibly reduce ketone, aldehyde, and quinone to alcohols with high selectivity (Baluha et al., 2013; Ma et al., 2010; Phillips and Smith, 2015). To verify the contribution of carbonyl molecules to the OP of organic aerosol, NaBH4 was employed to reduce carbonyls in WSOM to alcohols, and the changes in DTT activities before and after NaBH4 treatment were investigated. Two OA model samples were chosen to perform NaBH4 reduction, PFBHA derivatization, FT-ICR MS analysis, and DTT activity assay. One is SRNOM obtained from IHSS because it has the similar chemical composition to HULIS (Graber and Rudich, 2006; Havers et al., 1998); the other is WSOM extracted from diesel soot (WSOMds), as it is an important source of carbonyls in urban OA (Grosjean et al., 2001; Wang et al., 2023). FT-ICR MS results showed that SRNOM and WSOMds contained 1367 and 1722 carbonyl molecules, respectively (Fig. 3a and b). Unlike SRNOM, the carbonyl molecules of WSOMds had a higher number of CHON (48.1 %), likely due to the reaction between the high amount of nitrogen oxide (NOx) and VOCs released from diesel vehicles. The original OP of SRNOM and WSOMds were 23.83 ± 1.07 and 57.28 ± 3.05 . The higher OP of WSOMds was attributed to its higher contents of carbonyl molecules. After NaBH4 treatment, 62.9 % (619) and 64.1 % (1103) of carbonyl molecules in SRNOM and WSOMds were removed, respectively, leaving 24.5 % (335) and 37.1 % (619) of carbonyl molecules that cannot be removed (Fig. 3c and d). This is the first time that the effect of NaBH4 on the reduction of carbonyls is evaluated in WSOM instead of model compounds because the new detection method was used. As predicted, DTT activities of the SRNOM and WSOMds reduced 41.3 % and 77.2 % after NaBH4 treatment (Fig. 3e and f), which was consistent with the reduction of carbonyl molecules in two samples by NaBH4 treatments. Moreover, an ambient-collected OA sample (PM2.5=52.55 µg m−3) was used to verify the results obtained using model sample. As shown in Fig. S15, after NaBH4 treatment, the DTT activity of the real OA sample reduced by about 60 %, which was consistent with the results using the model samples. These results further confirmed the important role of carbonyl molecules in determining the OP of organic aerosol.
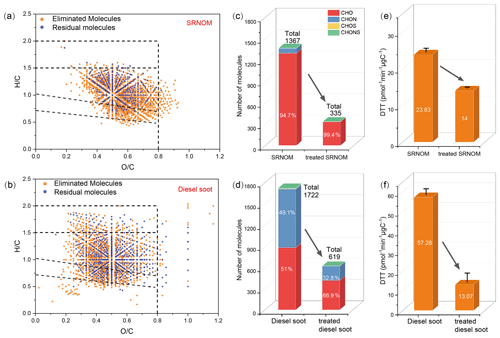
Figure 3Van Krevelen diagrams of carbonyl molecules in SRNOM (a) and WSOM extracted from diesel soot (b), with orange points representing carbonyl molecules eliminated by NaBH4 and blue points representing residual carbonyl molecules. Changes in carbonyl molecule composition of SRNOM (c) and WSOM extracted from diesel soot (d) before and after NaBH4 treatment. Changes in DTTOC before and after NaBH4 treatment of SRNOM (e) and WSOM extracted from diesel soot (f).
3.4 Molecular composition of carbonyls in PM2.5
Because of the importance of carbonyl molecules in determining the OP of PM2.5, molecular characteristics for carbonyls were studied further. In general, the DBEw and AImodw values of carbonyl molecules detected in winter were higher than those in summer, while the MWw, , and values of carbonyl molecules detected in winter were lower (Fig. S16). Using cluster analysis of the samples based on the composition of carbonyl molecules (Fig. 4a), two distinct clusters of summer and winter samples were successfully obtained. In one of the clusters, the samples collected during the strict control period of the Winter Olympics can be distinguished from the other winter samples. The proportions of carbonyl molecules in the five categories were estimated based on the molecular numbers. As shown in Fig. S17, highly unsaturated-like molecules were the most abundant components during winter (62.3 % ± 0.9 %) and the Winter Olympics (62.1 % ± 1.1 %), and their abundance was significantly higher than that in summer (58.2 % ± 2.3 %) (p<0.01). In summer, besides highly unsaturated-like molecules, unsaturated aliphatic-like molecules (33.2 % ± 2.2 %) were also one of the most dominant components. In addition, the abundance of highly aromatic-like molecules was significantly higher in winter (14.0 % ± 1.5 %) and Winter Olympics periods (14.0 % ± 0.6 %) than in summer (3.9 % ± 1.0 %) (p<0.05).
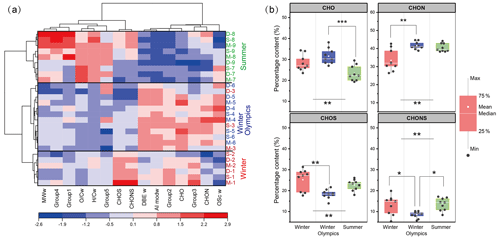
Figure 4Molecular composition and distribution characteristics of carbonyl molecules in WSOM of PM2.5 samples. (a) The heatmap shows the correlation between the carbonyl molecular characteristics and the samples collected from the winter (1–3), Winter Olympics (4–6), and summer (7–9) in the mountainous (M), suburban (S), and downtown site (D) (Group 1, polycyclic aromatic-like molecules; Group 2, highly aromatic-like molecules; Group 3, highly unsaturated-like molecules; Group 4, unsaturated aliphatic-like molecules; Group 5, saturated-like molecules). (b) The difference in the percentage distribution based on the molecular numbers of the elementary composition (CHO, CHON, CHOS, CHONS) of carbonyl molecules in winter, Winter Olympics, and summer samples.
The elementary composition of the carbonyl molecules was analyzed further. In general, the CHO-containing carbonyl molecules were distributed in regions with lower values dominated by highly aromatic-like and highly unsaturated-like molecules, while the sulfur-containing carbonyl molecules (CHOS, CHONS) were distributed in the region with higher and values dominated by unsaturated aliphatic-like and saturated-like molecules (Fig. S18). Furthermore, CHON-containing carbonyl molecules comprised the highest proportion among all sampling seasons (Fig. 4b). CHO-containing carbonyl molecules showed a higher proportion during the winter and Winter Olympics periods compared to summer. In particular, carbonyl molecules containing sulfur (CHOS and CHONS) exhibit a lower proportion during the Winter Olympics period compared to winter and summer. This discrepancy may be attributed to control measures implemented during the Winter Olympics, such as limiting the operations of high-emission and high-pollution enterprises, which potentially led to a reduction in sulfur-containing compounds. However, it is noteworthy that sulfur-containing carbonyls do not constitute the primary source of the aerosol OP, as the DTTOC during the Winter Olympics remained comparable to that observed on other days (Fig. 2d).
3.5 Potential carbonyl markers of atmospheric oxidative stress
Carbonyl compounds with different structures should have different DTT activities. The Spearman correlation matrix of the characteristic parameters of carbonyl molecules with DTTOC revealed that DBEw and AImodw were significantly positively correlated with DTTOC, while MWw, , and were significantly negatively correlated with DTTOC (Fig. S19). Thus, oligomers with high ratios and a range of ratios formed by atmospheric secondary oxidation (Kenseth et al., 2023; Nguyen et al., 2011), which are categorized as unsaturated aliphatic and saturated-like molecules, contribute weakly to oxidative potential. Conversely, the number of highly unsaturated and aromatic carbonyls (AImod>0.5, classified as polycyclic aromatic-like, highly aromatic-like, and highly unsaturated-like molecules in the VK diagram) in the samples showed a higher correlation with the DTTOC (Spearman's r=0.779, p<0.001), suggesting more attention should be paid to those carbonyl molecules.
In order to screen out potential carbonyl markers to indicate atmospheric oxidative stress (pro-oxidative carbonyls) from numerous carbonyl molecules, Spearman rank correlation and PLSR model analysis using the normalized intensities of carbonyl molecules with more than 50 % detection rate and DTTOC were performed. The results showed that 380 carbonyl molecules positively correlated with DTTOC had a value of variable importance in projection scores (VIP) greater than 1.0, indicating these carbonyl molecules had greater DTT activity and thus can be considered typical pro-oxidative carbonyls. As shown in Fig. 5a, these pro-oxidative carbonyls were mainly highly unsaturated-like (64 %) and highly aromatic-like molecules (33 %) and dominated by CHO (45.5 %) and CHON (42.6 %).
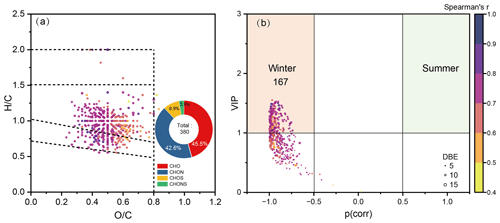
Figure 5(a) Van Krevelen diagram of pro-oxidative carbonyls. (b) From the OPLS-DA model, the contribution of each pro-oxidative carbonyls to winter versus summer in the volcano plot, showing seasonal variation markers with the highest model-correlation coefficients () and variable importance in projection scores (VIP>1.0). Features are colored by their Spearman correlation coefficients.
OPLS-DA model analysis was performed to further reveal the potential carbonyl markers in different seasons. Results showed that samples collected in winter and summer could be distinguished from each other by the pro-oxidative carbonyls (Fig. S20). Then, the molecules with VIP>1 and those that had the highest model-correlation coefficients () in the volcano plot were screened out as molecular markers in different seasons. Overall, 167 molecular markers in winter were found, which were mainly composed of CHO elements and had significantly higher aromaticity (DBE=11 ± 2, AImod=0.47 ± 0.16) and molecular weight (MW=368 ± 65) compared to other pro-oxidative carbonyls (DBE=9 ± 2, AImod=0.42 ± 0.19, MW=329 ± 65). However, no pro-oxidative carbonyls were found in summer (Fig. 5b) due to the low DTTOC of PM2.5 collected in summer. Previous study also found that oxygenated OA containing carbon–oxygen double bonds connected to aromatic structures contributes to cellular oxidative stress, with a higher contribution observed during winter compared to summer (Liu et al., 2023). These results suggested that carbonyl-containing highly unsaturated and aromatic products serve as potential molecular markers for atmospheric oxidative stress, especially during periods of increased combustion by-products such as the wintertime domestic heating season (Huang et al., 2018; Huo et al., 2021; Steimer et al., 2020).
PM2.5 contains a large number of organic compounds which can cause great potential harm to the ecological environment and human health. In this study, we investigated the abundance and molecular diversity of carbonyl and non-carbonyl molecules in WSOM from PM2.5 using FT-ICR MS analysis with chemical derivatization, taking Beijing as a research area. Carbonyl molecules in these samples displayed high molecular diversity, accounting for 11 %–22 % of the total number of identified organic molecules and 20 %–35 % of the total intensities of identified organic molecules. Compared with non-carbonyl molecules, more abundant carbonyl molecules are detected in winter than in summer, mainly due to the increasing fuel combustion emissions in winter. Through field sample analysis and simulation experiments, we found that carbonyl molecules played a pivotal role in determining the OP of organic aerosol. Among various carbonyl molecules, highly unsaturated and aromatic carbonyl molecules contributed more to atmospheric oxidative stress, suggesting more attention should be paid to the possible health hazards posed by atmospheric carbonyls. Highly unsaturated and aromatic carbonyl molecules are mainly sourced from fuel combustion and biomass burning processes such as wildfires, agricultural fires, and residential wood burning. In addition, although obvious improvement in PM2.5 pollution was achieved, the OP and carbonyl molecules of atmospheric aerosols did not change significantly during the 2022 Beijing Winter Olympics. Therefore, livelihood-related heating and household emissions contribute significantly to the abundance of atmospheric carbonyl molecules, leading to an elevated OP in atmospheric aerosols under the current air quality level in Beijing. As a result, the potential of regulating industrial and transportation emissions to improve the atmospheric OP in Beijing will be decreased in the future. The control of atmospheric carbonyl pollution is a more challenging task than that of PM2.5 pollution, and more scientific measures are needed to reduce the health hazards caused by atmospheric oxidation stress.
The data used in this study are available from the corresponding author upon request (jtlv@rcees.ac.cn).
The supplement related to this article is available online at: https://doi.org/10.5194/acp-24-8397-2024-supplement.
FL: methodology, formal analysis, investigation, visualization, writing (original draft). ST: validation, investigation, methodology. JL: conceptualization, methodology, formal analysis, writing (review and editing), supervision, funding acquisition. SY: methodology. XS: investigation. DC: methodology, validation. YW: conceptualization, writing (review and editing), supervision, funding acquisition. GJ: supervision, validation.
The contact author has declared that none of the authors has any competing interests.
Publisher's note: Copernicus Publications remains neutral with regard to jurisdictional claims made in the text, published maps, institutional affiliations, or any other geographical representation in this paper. While Copernicus Publications makes every effort to include appropriate place names, the final responsibility lies with the authors.
We are thankful for the help of the Beijing Urban Ecosystem Research Station in sampling and data sharing.
This research was jointly supported by the Strategic Priority Research Program of the Chinese Academy of Sciences (grant no. XDB0750000), the National Natural Science Foundation of China (grant nos. 22021003 and 22106031), the K.C. Wong 394 Education Foundation of China (grant no. GJTD-2020-03), and the Youth Innovation Promotion Association of the Chinese Academy of Sciences (grant no. 2020044).
This paper was edited by Drew Gentner and reviewed by two anonymous referees.
Baluha, D. R., Blough, N. V., and Del Vecchio, R.: Selective Mass Labeling for Linking the Optical Properties of Chromophoric Dissolved Organic Matter to Structure and Composition via Ultrahigh Resolution Electrospray Ionization Mass Spectrometry, Environ. Sci. Technol., 47, 9891–9897, https://doi.org/10.1021/es402400j, 2013.
Bates, J. T., Fang, T., Verma, V., Zeng, L., Weber, R. J., Tolbert, P. E., Abrams, J. Y., Sarnat, S. E., Klein, M., Mulholland, J. A., and Russell, A. G.: Review of Acellular Assays of Ambient Particulate Matter Oxidative Potential: Methods and Relationships with Composition, Sources, and Health Effects, Environ. Sci. Technol., 53, 4003–4019, https://doi.org/10.1021/acs.est.8b03430, 2019.
Bianco, A., Deguillaume, L., Vaïtilingom, M., Nicol, E., Baray, J.-L., Chaumerliac, N., and Bridoux, M.: Molecular Characterization of Cloud Water Samples Collected at the Puy de Dôme (France) by Fourier Transform Ion Cyclotron Resonance Mass Spectrometry, Environ. Sci. Technol., 52, 10275–10285, https://doi.org/10.1021/acs.est.8b01964, 2018.
Bora, S. and Shankarrao Adole, P.: Carbonyl stress in diabetics with acute coronary syndrome, Clin. Chim. Acta, 520, 78–86, https://doi.org/10.1016/j.cca.2021.06.002, 2021.
Campbell, S. J., Wolfer, K., Utinger, B., Westwood, J., Zhang, Z.-H., Bukowiecki, N., Steimer, S. S., Vu, T. V., Xu, J., Straw, N., Thomson, S., Elzein, A., Sun, Y., Liu, D., Li, L., Fu, P., Lewis, A. C., Harrison, R. M., Bloss, W. J., Loh, M., Miller, M. R., Shi, Z., and Kalberer, M.: Atmospheric conditions and composition that influence PM2.5 oxidative potential in Beijing, China, Atmos. Chem. Phys., 21, 5549–5573, https://doi.org/10.5194/acp-21-5549-2021, 2021.
Chen, J. Y., Jiang, H., Chen, S. J., Cullen, C., Ahmed, C. M. S., and Lin, Y.-H.: Characterization of electrophilicity and oxidative potential of atmospheric carbonyls, Environ. Sci.-Proc. Imp., 21, 856–866, https://doi.org/10.1039/C9EM00033J, 2019.
Chen, K., Raeofy, N., Lum, M., Mayorga, R., Woods, M., Bahreini, R., Zhang, H., and Lin, Y.-H.: Solvent effects on chemical composition and optical properties of extracted secondary brown carbon constituents, Aerosol Sci. Tech., 56, 917–930, https://doi.org/10.1080/02786826.2022.2100734, 2022.
Chen, K., Mayorga, R., Hamilton, C., Bahreini, R., Zhang, H., and Lin, Y.-H.: Contribution of Carbonyl Chromophores in Secondary Brown Carbon from Nighttime Oxidation of Unsaturated Heterocyclic Volatile Organic Compounds, Environ. Sci. Technol., 57, 20085–20096, https://doi.org/10.1021/acs.est.3c08872, 2023.
Chen, Q., Wang, M., Wang, Y., Zhang, L., Li, Y., and Han, Y.: Oxidative Potential of Water-Soluble Matter Associated with Chromophoric Substances in PM2.5 over Xi'an, China, Environ. Sci. Technol., 53, 8574–8584, https://doi.org/10.1021/acs.est.9b01976, 2019.
Claeys, M., Graham, B., Vas, G., Wang, W., Vermeylen, R., Pashynska, V., Cafmeyer, J., Guyon, P., Andreae, M. O., Artaxo, P., and Maenhaut, W.: Formation of Secondary Organic Aerosols Through Photooxidation of Isoprene, Science, 303, 1173–1176, https://doi.org/10.1126/science.1092805, 2004.
Daellenbach, K. R., Uzu, G., Jiang, J., Cassagnes, L.-E., Leni, Z., Vlachou, A., Stefenelli, G., Canonaco, F., Weber, S., Segers, A., Kuenen, J. J. P., Schaap, M., Favez, O., Albinet, A., Aksoyoglu, S., Dommen, J., Baltensperger, U., Geiser, M., El Haddad, I., Jaffrezo, J.-L., and Prévôt, A. S. H.: Sources of particulate-matter air pollution and its oxidative potential in Europe, Nature, 587, 414–419, https://doi.org/10.1038/s41586-020-2902-8, 2020.
Dou, J., Lin, P., Kuang, B.-Y., and Yu, J. Z.: Reactive Oxygen Species Production Mediated by Humic-like Substances in Atmospheric Aerosols: Enhancement Effects by Pyridine, Imidazole, and Their Derivatives, Environ. Sci. Technol., 49, 6457–6465, https://doi.org/10.1021/es5059378, 2015.
Du, H., Li, J., Wang, Z., Chen, X., Yang, W., Sun, Y., Xin, J., Pan, X., Wang, W., Ye, Q., and Dao, X.: Assessment of the effect of meteorological and emission variations on winter PM2.5 over the North China Plain in the three-year action plan against air pollution in 2018–2020, Atmos. Res., 280, 106395, https://doi.org/10.1016/j.atmosres.2022.106395, 2022.
Fu, T.-M., Jacob, D. J., Wittrock, F., Burrows, J. P., Vrekoussis, M., and Henze, D. K.: Global budgets of atmospheric glyoxal and methylglyoxal, and implications for formation of secondary organic aerosols, J. Geophys. Res.-Atmos., 113, D15303, https://doi.org/10.1029/2007JD009505, 2008.
Graber, E. R. and Rudich, Y.: Atmospheric HULIS: How humic-like are they? A comprehensive and critical review, Atmos. Chem. Phys., 6, 729–753, https://doi.org/10.5194/acp-6-729-2006, 2006.
Grosjean, D., Grosjean, E., and Gertler, A. W.: On-Road Emissions of Carbonyls from Light-Duty and Heavy-Duty Vehicles, Environ. Sci. Technol., 35, 45–53, https://doi.org/10.1021/es001326a, 2001.
Han, J., Wang, S., Yeung, K., Yang, D., Gu, W., Ma, Z., Sun, J., Wang, X., Chow, C.-W., Chan, A. W. H., and Peng, H.: Proteome-wide effects of naphthalene-derived secondary organic aerosol in BEAS-2B cells are caused by short-lived unsaturated carbonyls, P. Natl. Acad. Sci. USA, 117, 25386–25395, https://doi.org/10.1073/pnas.2001378117, 2020.
Havers, N., Burba, P., Lambert, J., and Klockow, D.: Spectroscopic Characterization of Humic-Like Substances in Airborne Particulate Matter, J. Atmos. Chem., 29, 45–54, https://doi.org/10.1023/A:1005875225800, 1998.
He, L. and Zhang, J. (Jim): Particulate matter (PM) oxidative potential: Measurement methods and links to PM physicochemical characteristics and health effects, Critical Reviews in Environmental Science and Technology, 9, 822–828, https://doi.org/10.1080/10643389.2022.2050148, 2022.
Ho, S. S. H. and Yu, J. Z.: Determination of Airborne Carbonyls: Comparison of a Thermal Desorption/GC Method with the Standard DNPH/HPLC Method, Environ. Sci. Technol., 38, 862–870, https://doi.org/10.1021/es034795w, 2004.
Huang, R.-J., Yang, L., Cao, J., Chen, Y., Chen, Q., Li, Y., Duan, J., Zhu, C., Dai, W., Wang, K., Lin, C., Ni, H., Corbin, J. C., Wu, Y., Zhang, R., Tie, X., Hoffmann, T., O'Dowd, C., and Dusek, U.: Brown Carbon Aerosol in Urban Xi'an, Northwest China: The Composition and Light Absorption Properties, Environ. Sci. Technol., 52, 6825–6833, https://doi.org/10.1021/acs.est.8b02386, 2018.
Huang, R.-J., Yang, L., Shen, J., Yuan, W., Gong, Y., Guo, J., Cao, W., Duan, J., Ni, H., Zhu, C., Dai, W., Li, Y., Chen, Y., Chen, Q., Wu, Y., Zhang, R., Dusek, U., O'Dowd, C., and Hoffmann, T.: Water-Insoluble Organics Dominate Brown Carbon in Wintertime Urban Aerosol of China: Chemical Characteristics and Optical Properties, Environ. Sci. Technol., 54, 7836–7847, https://doi.org/10.1021/acs.est.0c01149, 2020.
Huo, Y., Guo, Z., Li, Q., Wu, D., Ding, X., Liu, A., Huang, D., Qiu, G., Wu, M., Zhao, Z., Sun, H., Song, W., Li, X., Chen, Y., Wu, T., and Chen, J.: Chemical Fingerprinting of HULIS in Particulate Matters Emitted from Residential Coal and Biomass Combustion, Environ. Sci. Technol., 55, 3593–3603, https://doi.org/10.1021/acs.est.0c08518, 2021.
Ji, Y., Shi, Q., Li, Y., An, T., Zheng, J., Peng, J., Gao, Y., Chen, J., Li, G., Wang, Y., Zhang, F., Zhang, A. L., Zhao, J., Molina, M. J., and Zhang, R.: Carbenium ion-mediated oligomerization of methylglyoxal for secondary organic aerosol formation, P. Natl. Acad. Sci. USA, 117, 13294–13299, https://doi.org/10.1073/pnas.1912235117, 2020.
Jiang, H. and Jang, M.: Dynamic Oxidative Potential of Atmospheric Organic Aerosol under Ambient Sunlight, Environ. Sci. Technol., 52, 7496–7504, https://doi.org/10.1021/acs.est.8b00148, 2018.
Jiang, H., Li, J., Sun, R., Tian, C., Tang, J., Jiang, B., Liao, Y., Chen, C.-E., and Zhang, G.: Molecular Dynamics and Light Absorption Properties of Atmospheric Dissolved Organic Matter, Environ. Sci. Technol., 55, 10268–10279, https://doi.org/10.1021/acs.est.1c01770, 2021.
Jiang, H., Li, J., Tang, J., Cui, M., Zhao, S., Mo, Y., Tian, C., Zhang, X., Jiang, B., Liao, Y., Chen, Y., and Zhang, G.: Molecular characteristics, sources, and formation pathways of organosulfur compounds in ambient aerosol in Guangzhou, South China, Atmos. Chem. Phys., 22, 6919–6935, https://doi.org/10.5194/acp-22-6919-2022, 2022.
Kalberer, M., Paulsen, D., Sax, M., Steinbacher, M., Dommen, J., Prevot, A. S. H., Fisseha, R., Weingartner, E., Frankevich, V., Zenobi, R., and Baltensperger, U.: Identification of Polymers as Major Components of Atmospheric Organic Aerosols, Science, 303, 1659–1662, https://doi.org/10.1126/science.1092185, 2004.
Kenseth, C. M., Hafeman, N. J., Rezgui, S. P., Chen, J., Huang, Y., Dalleska, N. F., Kjaergaard, H. G., Stoltz, B. M., Seinfeld, J. H., and Wennberg, P. O.: Particle-phase accretion forms dimer esters in pinene secondary organic aerosol, Science, 382, 787–792, https://doi.org/10.1126/science.adi0857, 2023.
Kroll, J. H., Donahue, N. M., Jimenez, J. L., Kessler, S. H., Canagaratna, M. R., Wilson, K. R., Altieri, K. E., Mazzoleni, L. R., Wozniak, A. S., Bluhm, H., Mysak, E. R., Smith, J. D., Kolb, C. E., and Worsnop, D. R.: Carbon oxidation state as a metric for describing the chemistry of atmospheric organic aerosol, Nat. Chem., 3, 133–139, https://doi.org/10.1038/nchem.948, 2011.
Kumagai, Y., Koide, S., Taguchi, K., Endo, A., Nakai, Y., Yoshikawa, T., and Shimojo, N.: Oxidation of Proximal Protein Sulfhydryls by Phenanthraquinone, a Component of Diesel Exhaust Particles, Chem. Res. Toxicol., 15, 483–489, https://doi.org/10.1021/tx0100993, 2002.
Lary, D. J. and Shallcross, D. E.: Central role of carbonyl compounds in atmospheric chemistry, J. Geophys. Res.-Atmos., 105, 19771–19778, https://doi.org/10.1029/1999JD901184, 2000.
Li, F., Tang, S., Lv, J., He, A., Wang, Y., Liu, S., Cao, H., Zhao, L., Wang, Y., and Jiang, G.: Molecular-Scale Investigation on the Formation of Brown Carbon Aerosol via Iron-Phenolic Compound Reactions in the Dark, Environ. Sci. Technol., 57, 11173–11184, https://doi.org/10.1021/acs.est.3c04263, 2023.
Li, M., Yang, D., Brock, G., Knipp, R. J., Bousamra, M., Nantz, M. H., and Fu, X.-A.: Breath carbonyl compounds as biomarkers of lung cancer, Lung Cancer, 90, 92–97, https://doi.org/10.1016/j.lungcan.2015.07.005, 2015.
Li, Y., Lei, L., Sun, J., Gao, Y., Wang, P., Wang, S., Zhang, Z., Du, A., Li, Z., Wang, Z., Kim, J. Y., Kim, H., Zhang, H., and Sun, Y.: Significant Reductions in Secondary Aerosols after the Three-Year Action Plan in Beijing Summer, Environ. Sci. Technol., 57, 15945–15955, https://doi.org/10.1021/acs.est.3c02417, 2023.
Lin, M. and Yu, J. Z.: Assessment of Interactions between Transition Metals and Atmospheric Organics: Ascorbic Acid Depletion and Hydroxyl Radical Formation in Organic-Metal Mixtures, Environ. Sci. Technol., 54, 1431–1442, https://doi.org/10.1021/acs.est.9b07478, 2020.
Lin, P. and Yu, J. Z.: Generation of Reactive Oxygen Species Mediated by Humic-like Substances in Atmospheric Aerosols, Environ. Sci. Technol., 45, 10362–10368, https://doi.org/10.1021/es2028229, 2011.
Liu, F., Joo, T., Ditto, J. C., Saavedra, M. G., Takeuchi, M., Boris, A. J., Yang, Y., Weber, R. J., Dillner, A. M., Gentner, D. R., and Ng, N. L.: Oxidized and Unsaturated: Key Organic Aerosol Traits Associated with Cellular Reactive Oxygen Species Production in the Southeastern United States, Environ. Sci. Technol., 57, 14150–14161, https://doi.org/10.1021/acs.est.3c03641, 2023.
Liu, Q., Gao, Y., Huang, W., Ling, Z., Wang, Z., and Wang, X.: Carbonyl compounds in the atmosphere: A review of abundance, source and their contributions to O3 and SOA formation, Atmos. Res., 274, 106184, https://doi.org/10.1016/j.atmosres.2022.106184, 2022.
Liu, W., Xu, Y., Liu, W., Liu, Q., Yu, S., Liu, Y., Wang, X., and Tao, S.: Oxidative potential of ambient PM2.5 in the coastal cities of the Bohai Sea, northern China: Seasonal variation and source apportionment, Environ. Pollut., 236, 514–528, https://doi.org/10.1016/j.envpol.2018.01.116, 2018.
Liu, X., Liu, R., Zhu, B., Ruan, T., and Jiang, G.: Characterization of Carbonyl Disinfection By-Products During Ozonation, Chlorination, and Chloramination of Dissolved Organic Matters, Environ. Sci. Technol., 54, 2218–2227, https://doi.org/10.1021/acs.est.9b04875, 2020.
Liu, Y., Xu, X., Yang, X., He, J., Ji, D., and Wang, Y.: Significant Reduction in Fine Particulate Matter in Beijing during 2022 Beijing Winter Olympics, Environ. Sci. Tech. Let., 53, 177–197, https://doi.org/10.1021/acs.estlett.2c00532, 2022.
Lv, J., Zhang, S., Wang, S., Luo, L., Cao, D., and Christie, P.: Molecular-Scale Investigation with ESI-FT-ICR-MS on Fractionation of Dissolved Organic Matter Induced by Adsorption on Iron Oxyhydroxides, Environ. Sci. Technol., 50, 2328–2336, https://doi.org/10.1021/acs.est.5b04996, 2016.
Lyu, Y., Guo, H., Cheng, T., and Li, X.: Particle Size Distributions of Oxidative Potential of Lung-Deposited Particles: Assessing Contributions from Quinones and Water-Soluble Metals, Environ. Sci. Technol., 52, 6592–6600, https://doi.org/10.1021/acs.est.7b06686, 2018.
Ma, J., Del Vecchio, R., Golanoski, K. S., Boyle, E. S., and Blough, N. V.: Optical Properties of Humic Substances and CDOM: Effects of Borohydride Reduction, Environ. Sci. Technol., 44, 5395–5402, https://doi.org/10.1021/es100880q, 2010.
Ma, J., Ungeheuer, F., Zheng, F., Du, W., Wang, Y., Cai, J., Zhou, Y., Yan, C., Liu, Y., Kulmala, M., Daellenbach, K. R., and Vogel, A. L.: Nontarget Screening Exhibits a Seasonal Cycle of PM2.5 Organic Aerosol Composition in Beijing, Environ. Sci. Technol., 56, 7017–7028, https://doi.org/10.1021/acs.est.1c06905, 2022.
Ma, Y., Cheng, Y., Qiu, X., Cao, G., Fang, Y., Wang, J., Zhu, T., Yu, J., and Hu, D.: Sources and oxidative potential of water-soluble humic-like substances (HULISWS) in fine particulate matter (PM2.5) in Beijing, Atmos. Chem. Phys., 18, 5607–5617, https://doi.org/10.5194/acp-18-5607-2018, 2018.
Nguyen, T. B., Roach, P. J., Laskin, J., Laskin, A., and Nizkorodov, S. A.: Effect of humidity on the composition of isoprene photooxidation secondary organic aerosol, Atmos. Chem. Phys., 11, 6931–6944, https://doi.org/10.5194/acp-11-6931-2011, 2011.
Papazian, S., D'Agostino, L. A., Sadiktsis, I., Froment, J., Bonnefille, B., Sdougkou, K., Xie, H., Athanassiadis, I., Budhavant, K., Dasari, S., Andersson, A., Gustafsson, Ö., and Martin, J. W.: Nontarget mass spectrometry and in silico molecular characterization of air pollution from the Indian subcontinent, Commun. Earth Environ., 3, 35, https://doi.org/10.1038/s43247-022-00365-1, 2022.
Phillips, S. M. and Smith, G. D.: Light Absorption by Charge Transfer Complexes in Brown Carbon Aerosols, Environ. Sci. Tech. Let., 1, 382–386, https://doi.org/10.1021/ez500263j, 2014.
Phillips, S. M. and Smith, G. D.: Further Evidence for Charge Transfer Complexes in Brown Carbon Aerosols from Excitation–Emission Matrix Fluorescence Spectroscopy, J. Phys. Chem. A, 119, 4545–4551, https://doi.org/10.1021/jp510709e, 2015.
Powelson, M. H., Espelien, B. M., Hawkins, L. N., Galloway, M. M., and De Haan, D. O.: Brown Carbon Formation by Aqueous-Phase Carbonyl Compound Reactions with Amines and Ammonium Sulfate, Environ. Sci. Technol., 48, 985–993, https://doi.org/10.1021/es4038325, 2014.
Qian, X., Shen, H., and Chen, Z.: Characterizing summer and winter carbonyl compounds in Beijing atmosphere, Atmos. Environ., 214, 116845, https://doi.org/10.1016/j.atmosenv.2019.116845, 2019.
Rappaport, S. M., Li, H., Grigoryan, H., Funk, W. E., and Williams, E. R.: Adductomics: Characterizing exposures to reactive electrophiles, Toxicol. Lett., 213, 83–90, https://doi.org/10.1016/j.toxlet.2011.04.002, 2012.
Shen, H., Chen, Z., Li, H., Qian, X., Qin, X., and Shi, W.: Gas-Particle Partitioning of Carbonyl Compounds in the Ambient Atmosphere, Environ. Sci. Technol., 52, 10997–11006, https://doi.org/10.1021/acs.est.8b01882, 2018.
Song, J., Li, M., Fan, X., Zou, C., Zhu, M., Jiang, B., Yu, Z., Jia, W., Liao, Y., and Peng, P.: Molecular Characterization of Water- and Methanol-Soluble Organic Compounds Emitted from Residential Coal Combustion Using Ultrahigh-Resolution Electrospray Ionization Fourier Transform Ion Cyclotron Resonance Mass Spectrometry, Environ. Sci. Technol., 53, 13607–13617, https://doi.org/10.1021/acs.est.9b04331, 2019.
Song, J., Li, M., Zou, C., Cao, T., Fan, X., Jiang, B., Yu, Z., Jia, W., and Peng, P.: Molecular Characterization of Nitrogen-Containing Compounds in Humic-like Substances Emitted from Biomass Burning and Coal Combustion, Environ. Sci. Technol., 56, 119–130, https://doi.org/10.1021/acs.est.1c04451, 2022.
Steimer, S. S., Patton, D. J., Vu, T. V., Panagi, M., Monks, P. S., Harrison, R. M., Fleming, Z. L., Shi, Z., and Kalberer, M.: Differences in the composition of organic aerosols between winter and summer in Beijing: a study by direct-infusion ultrahigh-resolution mass spectrometry, Atmos. Chem. Phys., 20, 13303–13318, https://doi.org/10.5194/acp-20-13303-2020, 2020.
Su, S., Xie, Q., Lang, Y., Cao, D., Xu, Y., Chen, J., Chen, S., Hu, W., Qi, Y., Pan, X., Sun, Y., Wang, Z., Liu, C.-Q., Jiang, G., and Fu, P.: High Molecular Diversity of Organic Nitrogen in Urban Snow in North China, Environ. Sci. Technol., 55, 4344–4356, https://doi.org/10.1021/acs.est.0c06851, 2021.
Tang, S., Li, F., Lv, J., Liu, L., Wu, G., Wang, Y., Yu, W., Wang, Y., and Jiang, G.: Unexpected molecular diversity of brown carbon formed by Maillard-like reactions in aqueous aerosols, Chem. Sci., 13, 8401–8411, https://doi.org/10.1039/D2SC02857C, 2022.
Tuet, W. Y., Chen, Y., Xu, L., Fok, S., Gao, D., Weber, R. J., and Ng, N. L.: Chemical oxidative potential of secondary organic aerosol (SOA) generated from the photooxidation of biogenic and anthropogenic volatile organic compounds, Atmos. Chem. Phys., 17, 839–853, https://doi.org/10.5194/acp-17-839-2017, 2017.
Verma, V., Rico-Martinez, R., Kotra, N., King, L., Liu, J., Snell, T. W., and Weber, R. J.: Contribution of Water-Soluble and Insoluble Components and Their Hydrophobic/Hydrophilic Subfractions to the Reactive Oxygen Species-Generating Potential of Fine Ambient Aerosols, Environ. Sci. Technol., 46, 11384–11392, https://doi.org/10.1021/es302484r, 2012.
Wang, J., Lin, X., Lu, L., Wu, Y., Zhang, H., Lv, Q., Liu, W., Zhang, Y., and Zhuang, S.: Temporal variation of oxidative potential of water soluble components of ambient PM2.5 measured by dithiothreitol (DTT) assay, Sci. Total Environ., 649, 969–978, https://doi.org/10.1016/j.scitotenv.2018.08.375, 2019.
Wang, L., Lin, Y., Ye, L., Qian, Y., Shi, Y., Xu, K., Ren, H., and Geng, J.: Microbial Roles in Dissolved Organic Matter Transformation in Full-Scale Wastewater Treatment Processes Revealed by Reactomics and Comparative Genomics, Environ. Sci. Technol., 55, 11294–11307, https://doi.org/10.1021/acs.est.1c02584, 2021.
Wang, L., Wen, W., Yan, J., Zhang, R., Li, C., Jiang, H., Chen, S., Pardo, M., Zhu, K., Jia, B., Zhang, W., Bai, Z., Shi, L., Cheng, Y., Rudich, Y., Morawska, L., and Chen, J.: Influence of Polycyclic Aromatic Compounds and Oxidation States of Soot Organics on the Metabolome of Human-Lung Cells (A549): Implications for Vehicle Fuel Selection, Environ. Sci. Technol., 57, 21593–21604, https://doi.org/10.1021/acs.est.3c05228, 2023.
Wang, S., Ye, J., Soong, R., Wu, B., Yu, L., Simpson, A. J., and Chan, A. W. H.: Relationship between chemical composition and oxidative potential of secondary organic aerosol from polycyclic aromatic hydrocarbons, Atmos. Chem. Phys., 18, 3987–4003, https://doi.org/10.5194/acp-18-3987-2018, 2018.
Wang, Z., Chen, X., Liang, Y., and Shi, Q.: Molecular characterization of carbonyl compounds in atmospheric fine particulate matters (PM2.5) in Beijing by derivatization with Girard's reagent T combined with positive-ion ESI Orbitrap MS, Atmos. Res., 273, 106176, https://doi.org/10.1016/j.atmosres.2022.106176, 2022.
Xie, Q., Su, S., Dai, Y., Hu, W., Yue, S., Cao, D., Jiang, G., and Fu, P.: Deciphering 13C and 34S Isotopes of Organosulfates in Urban Aerosols by FT-ICR Mass Spectrometry, Environ. Sci. Tech. Let., 9, 526–532, https://doi.org/10.1021/acs.estlett.2c00255, 2022.
Xu, Y., Feng, X., Chen, Y., Zheng, P., Hui, L., Chen, Y., Yu, J. Z., and Wang, Z.: Development of an enhanced method for atmospheric carbonyls and characterizing their roles in photochemistry in subtropical Hong Kong, Sci. Total Environ., 896, 165135, https://doi.org/10.1016/j.scitotenv.2023.165135, 2023.
Yang, L., Huang, R.-J., Shen, J., Wang, T., Gong, Y., Yuan, W., Liu, Y., Huang, H., You, Q., Huang, D. D., and Huang, C.: New Insights into the Brown Carbon Chromophores and Formation Pathways for Aqueous Reactions of α-Dicarbonyls with Amines and Ammonium, Environ. Sci. Technol., 57, 12351–12361, https://doi.org/10.1021/acs.est.3c04133, 2023.
Ye, C., Liu, Y., Yuan, B., Wang, Z., Lin, Y., Hu, W., Chen, W., Li, T., Song, W., Wang, X., Lv, D., Gu, D., and Shao, M.: Low-NO-like Oxidation Pathway Makes a Significant Contribution to Secondary Organic Aerosol in Polluted Urban Air, Environ. Sci. Technol., 57, 13912–13924, https://doi.org/10.1021/acs.est.3c01055, 2023.
Yu, Q., Chen, J., Qin, W., Ahmad, M., Zhang, Y., Sun, Y., Xin, K., and Ai, J.: Oxidative potential associated with water-soluble components of PM2.5 in Beijing: The important role of anthropogenic organic aerosols, J. Hazard. Mater., 433, 128839, https://doi.org/10.1016/j.jhazmat.2022.128839, 2022.
Yu, S., Tang, S., Lv, J., Li, F., Huang, Z., Zhao, L., Cao, D., and Wang, Y.: High throughput identification of carbonyl compounds in natural organic matter by directional derivatization combined with ultra-high resolution mass spectrometry, Water Res., 258, 121769, https://doi.org/10.1016/j.watres.2024.121769, 2024.
Yu, W., Shen, X., Wu, B., Kong, L., Xuan, K., Zhao, C., Cao, X., Hao, X., Li, X., Zhang, H., and Yao, Z.: Real-world emission characteristics of carbonyl compounds from agricultural machines based on a portable emission measurement system, J. Environ. Sci., 124, 846–859, https://doi.org/10.1016/j.jes.2022.02.031, 2023.
Yue, S., Zhu, J., Chen, S., Xie, Q., Li, W., Li, L., Ren, H., Su, S., Li, P., Ma, H., Fan, Y., Cheng, B., Wu, L., Deng, J., Hu, W., Ren, L., Wei, L., Zhao, W., Tian, Y., Pan, X., Sun, Y., Wang, Z., Wu, F., Liu, C.-Q., Su, H., Penner, J. E., Pöschl, U., Andreae, M. O., Cheng, Y., and Fu, P.: Brown carbon from biomass burning imposes strong circum-Arctic warming, One Earth, 5, 293–304, https://doi.org/10.1016/j.oneear.2022.02.006, 2022.
Zeng, Y., Shen, Z., Takahama, S., Zhang, L., Zhang, T., Lei, Y., Zhang, Q., Xu, H., Ning, Y., Huang, Y., Cao, J., and Rudolf, H.: Molecular Absorption and Evolution Mechanisms of PM2.5 Brown Carbon Revealed by Electrospray Ionization Fourier Transform–Ion Cyclotron Resonance Mass Spectrometry During a Severe Winter Pollution Episode in Xi'an, China, Geophys. Res. Lett., 47, e2020GL087977, https://doi.org/10.1029/2020GL087977, 2020.
Zhang, Q., Ma, H., Li, J., Jiang, H., Chen, W., Wan, C., Jiang, B., Dong, G., Zeng, X., Chen, D., Lu, S., You, J., Yu, Z., Wang, X., and Zhang, G.: Nitroaromatic Compounds from Secondary Nitrate Formation and Biomass Burning Are Major Proinflammatory Components in Organic Aerosols in Guangzhou: A Bioassay Combining High-Resolution Mass Spectrometry Analysis, Environ. Sci. Technol., 57, 21570–21580, https://doi.org/10.1021/acs.est.3c04983, 2023.