the Creative Commons Attribution 4.0 License.
the Creative Commons Attribution 4.0 License.
Technical note: A method for calculating offsets to ozone depletion and climate impacts of ozone-depleting substances
Stephen A. Montzka
Stephen O. Andersen
Richard Ferris
By phasing out production and consumption of most ozone-depleting substances (ODSs), the Montreal Protocol on Substances that Deplete the Ozone Layer (Montreal Protocol) has avoided consequences of increased ultraviolet (UV) radiation and will restore stratospheric ozone to pre-1980 conditions by mid-century, assuming compliance with the phaseout. However, several studies have documented an unexpected increase in emissions and suggested unreported production of trichlorofluoromethane (CFC-11) and potentially other ODSs after 2012 despite production phaseouts under the Montreal Protocol. Furthermore, because most ODSs are powerful greenhouse gases (GHGs), there are significant climate protection benefits in collecting and destroying the substantial quantities of historically allowed production of chemicals under the Montreal Protocol that are contained in existing equipment and products and referred to as ODS “banks”. This technical note presents a framework for considering offsets to ozone depletion, climate forcing, and other environmental impacts arising from occurrences of unexpected emissions and unreported production of Montreal Protocol controlled substances, as recently experienced and likely to be experienced again. We also show how this methodology could be applied to the destruction of banks of controlled ODSs and GHGs or to halon or other production allowed under a Montreal Protocol Essential Use Exemption or Critical Use Exemption. Further, we roughly estimate the magnitude of offset each type of action could provide for ozone depletion, climate, and other environmental impacts that Montreal Protocol Parties agree warrant remedial action.
- Article
(386 KB) - Full-text XML
-
Supplement
(388 KB) - BibTeX
- EndNote
The stratospheric ozone layer shields Earth against ultraviolet (UV) radiation that causes skin cancer and cataracts, suppresses the human immune system, damages agricultural and natural ecosystems including terrestrial carbon sinks, and deteriorates the built environment (UNEP/EEAP, 2019; Bais et al., 2018; Young et al., 2021). Ozone-depleting substances (ODSs) deplete stratospheric ozone, thus increasing the amount of UV radiation reaching Earth's surface. Some ODSs, primarily chlorofluorocarbons (CFCs), hydrochlorofluorocarbons (HCFCs), carbon tetrachloride (CTC or CCl4), and halons, are also potent greenhouse gases (GHGs) (Ramanathan, 1975) (WMO, 2022), as are long-lived hydrofluorocarbons (HFCs) used as ODS substitutes.
The 1987 Montreal Protocol is an international treaty that has already phased out more than 99 % of the production and consumption of about 100 ozone-depleting GHGs and will soon phase down about a dozen HFCs that do not contain ozone-depleting chlorine or bromine. The United States Environmental Protection Agency (US EPA) has estimated that ODS phaseout under the fully revised and amended Montreal Protocol compared with a scenario of no controls will prevent approximately 443 million cases of skin cancer, 2.3 million skin cancer deaths, and 63 million cataract cases for people in the United States born in the years 1890–2100 (US EPA, 2020; Madronich et al., 2021). Global impacts are significantly higher considering that these estimates are for the US alone, representing about 4.25 % of the global population, and do not include the economic consequences of the full spectrum of health, agricultural productivity, and product deterioration. Even a seemingly small increase in UV radiation from unexpected emissions of unreported CFC-11 production has been estimated to contribute to an additional 31 600 to 59 800 cases of skin cancer as well as 170 to 340 deaths and 4100 to 9300 cases of cataracts that would otherwise have been avoided in the US alone (US EPA, 2020). Consider also that every ecosystem would suffer adverse effects owing to any increase in damaging UV radiation (Young et al., 2021). Furthermore, the family and community consequences are far worse in societies without adequate health and where food is already in short supply (Andersen and Sarma, 2002).
In May 2018, scientists warned that emissions of CFC-11 had unexpectedly increased despite a production phaseout under the Montreal Protocol (Montzka et al., 2018). In May 2019, scientists pinpointed ∼ 60 ± 40 % of unexpected emission increase to an area in China's northeastern provinces of Shandong and Hebei and found no evidence of a significant increase in CFC-11 emissions from any other locations where monitoring stations are sensitive to emissions on a regional scale (Rigby et al., 2019; Adcock et al., 2020). Over the course of 2018 and 2019, the unexpected emissions and unreported production of CFC-11 globally and from eastern China dropped substantially (Montzka et al., 2021; Park et al., 2021). Enhanced emissions of dichlorodifluoromethane (CFC-12) from eastern China, perhaps associated with CFC-11 production, have also been suggested (Park et al., 2021). A separate study, which analyzed 27 whole-air samples collected in 2016 over Hebei Province, implied new production and emissions of CFC-11, CFC-12, and 1,2-dichlorotetrafluoroethane (CFC-114) in various locations in China during spring 2016 (Benish et al., 2021). Another analysis of global atmospheric concentrations of CFC-11, CFC-12, and 1,1,2-trichloro-1,2,2-trifluoroethane (CFC-113) confirmed unexpected emissions of CFC-11 but suggested the possibility of unexpected emissions of these other gases during 2014–2016 and called for further investigation of potential sources of these emissions (Lickley et al., 2021). Increases in global emission and atmospheric concentrations of several CFCs with production allowed under the Montreal Protocol for use as feedstocks in the production of hydrofluorocarbons, e.g., 1,1,1-trichloro-2,2,2-trifluoroethane (CFC-113a), CFC-114a, and chloropentafluoroethane (CFC-115), are also being observed, together with emission increases in CFC-13 and CFC-112a, although the driver of the increase for these latter two CFCs is unclear (Western et al., 2023).
Emissions of another Montreal Protocol-controlled substance, CTC, have also been substantially higher than expected after the phaseout of CFC production (SPARC, 2016). CTC is used as a feedstock in the production of CFC-11 and CFC-12 and as a solvent. While the ongoing CTC emissions have not yet been implicated in non-compliance with the Montreal Protocol, they add significantly to the ozone-depleting halogen burden of the atmosphere. Hence, stakeholders may benefit from understanding how to offset the impacts of such emissions on stratospheric ozone and its recovery and to offset the impacts on climate forcing.
Here we propose an approach for calculating the quantity of ODSs for offsetting adverse environmental impacts arising from occurrences of unreported and unauthorized production ODSs and chemical substitutes such as HFCs. These offsets could take the form of preventing the emissions of ODSs and HFCs that were legally produced and would otherwise be emitted, such as through collecting and destroying banks of these chemicals. Other options are highlighted that could also be considered to offset the ozone depletion, climate, and other environmental impacts arising from instances of unexpected emissions or unreported production that Montreal Protocol Parties agree warrant remedial action. Note that an offset approach could also be applied to management and destruction of ODS banks or could be used to manage halon production allowed under a Montreal Protocol Essential Use Exemption in cases where entities are allowed to use and emit available halon banks.
An important aspect of offsetting impacts relates to the timing of the impact compared to the offset. Given the added uncertainties associated with estimating the year-to-year impacts of unexpected or illicit production and the associated emission that one might hope to offset, we focus here on offsetting cumulative impacts. We note that this approach is the only possible path to offset adverse impacts of uncertain emissions that occurred in the past. We also recognize that the approach of offsetting impacts with a cumulative time frame and not year by year will lead to a different time history for an impact compared to the offset, especially when the chemical being considered for supplying an offset has a substantially different lifetime than the chemical causing the adverse impact. This latter point will likely always be true when devising an offset to an impact that has already occurred. Consider, however, that environmental justice usually requires judgment of both the environmental impact and which victims deserve and qualify for compensation. The approach to calculating offsets presented here offsets cumulative impacts but does not compensate those harmed by increased UV radiation.
Atmospheric observations of long-lived ODS and HFC substances can provide an estimate of an unexpected emission magnitude that is to be offset. For many halocarbon substances, however, anomalous emissions will represent only a fraction of the total amount of chemical produced and the total cumulative impact, owing to the retention of chemicals in cooling appliances, fire protection equipment, closed-cell foams, and emission from the banks well after original production and use. Relating changes in atmospheric concentrations to production and, therefore, a more complete picture of the cumulative impact into the future as the banked chemical slowly escapes into the atmosphere requires an understanding of how substances are produced and used. We summarize in the Supplement typical historical production and uses for several ODSs for which unexpected emissions have been observed.
The damages from unexpected emissions and unreported production of substances controlled under the Montreal Protocol can be quantified for the impacts related to ozone depletion for ODSs, UV radiation exposure to estimate health and environmental effects, damage to the terrestrial carbon sink, deterioration of the built environment, and climate forcing for GHGs. In an ideal scenario, an offset would match the impacts year by year. However, this is likely to be impractical due to differences in the time-dependent impacts of different chemicals due to differences in potency and lifetimes. In addition, unreported production and emissions of the controlled substances to be offset would likely precede any offset actions. For these reasons, we focus here on estimating cumulative impacts and offsets, although we realize that there are limitations of this approach, e.g., in cases where the impact is nonlinearly related to the atmospheric abundance, as in the case of biological effects that depend on behavioral and other factors (Slaper et al., 1996). Specifically, we propose using the established metrics of ozone depletion potential (ODP) and global warming potential (GWP) when calculating offsets associated with an emission. While the ODP is defined as the ratio at steady state of calculated ozone column change for each mass unit of a gas emitted into the atmosphere relative to the calculated depletion for the reference gas CFC-11 (Fisher et al., 1990), it is also true that the ODP can reliably be used to estimate the cumulative impacts arising from a pulsed emission (Prather, 2002). In this way, ODP integrates the cumulative impact on the ozone column of a chemical relative to CFC-11 over the lifetime of the chemical and the timescale of secondary impacts. The ODP differs from the GWP in one important respect, however, in that the GWP reflects the ratio of a change in radiative forcing from an emission of gas relative to that same mass emission of carbon dioxide integrated over a specific time horizon (usually 100 or 20 years) and not over the lifetime of the chemical and its impacts. Choosing an appropriate integration period for estimating the impact and deriving an appropriate offset will therefore require a choice to be made, and this choice hinges on the relative importance of near-term vs. long-term impacts. We discuss additional considerations of impacts and offset metrics in this section by type of impact and conclude with an illustrative example.
3.1 Ozone depletion for ODSs
The approach of offsetting, through a reduction in emission or production of an ODS, the cumulative ozone depletion arising from unexpected or illicit emissions after weighting those emissions by the ODP, is supported by the near-linear relationships between cumulative emissions of a particular long-lived ODS and stratospheric ozone impacts from that ODS, both globally and over the Antarctic (Keeble et al., 2020; Fleming et al., 2020; Dhomse et al., 2019) as summarized in the WMO et al. (2021). This is because the impacts on stratospheric ozone of an emission roughly scale by the amount of chlorine (Cl) released into the stratosphere, all other factors (aerosol loading, etc.) being equal, and so can be applied to CFC-12, CTC, and other ODS species (Dhomse et al., 2019; Keeble et al., 2020; WMO et al., 2021). Other metrics such as the integrated ozone depletion (IOD) could be used to quantify the impact on stratospheric ozone of an emission to be offset, and use of this metric would provide results very similar to the use of ODP unless the chemical being used to offset an impact had a substantially different loss frequency in the troposphere and stratosphere (Pyle et al., 2022).
The ODPs used in Table 1 are based on atmospheric model simulations and can be expressed as a semi-empirical relationship:
where nCl is the number of chlorine atoms in the molecule, fi is the fractional release factor for the molecule, τi is the total lifetime, and mi is the molecular mass of the molecule (Burkholder et al., 2022).
The IOD for comparison, which is not used here, is given by Eq. (2):
where K=100 ± 16 Dobson unit years per Tg Cl, and EEq is the total emission in Tg Cl, multiplied by the ratio of the whole atmosphere lifetime of the molecule to its stratospheric lifetime, (Pyle et al., 2022).
3.2 Environmental impacts from ozone depletion
UV radiation exposure can be estimated from ozone depletion to estimate health and environmental effects. For example, the US EPA used its Atmospheric and Health Effects Framework (AHEF) model to estimate that, in the USA alone, the unexpected CFC-11 emissions,1 absent offset, would result in nearly 60 000 cancer deaths through 2100 that compliance with the Montreal Protocol would have avoided (US EPA, 2020). This and other health effect models can be extrapolated by taking into account geographic location, genetic vulnerability, lifestyle differences, and access to preventative and therapeutic mitigation (Slaper et al., 1996; Longstreth et al., 1998; Struijs et al., 2010; van Dijk et al., 2013). A calculation of the health and environmental impacts from ozone depletion and global warming of emissions is beyond the scope of this paper, as the authors are unaware of simplified metrics for these impacts analogous to the metrics for estimating ozone column and climate impacts. In addition to human health impacts, UV exposure can harm aboveground plant biomass and diminish the uptake of carbon dioxide (CO2) by the terrestrial biosphere in its capacity as a carbon sink. Studies suggest a UV response strength of a 3 % reduction in biomass for a 10 % increase in plant-weighted surface UV fluxes which can be related to total column ozone (Young et al., 2021). These impacts could also be included in deriving appropriate offsets if desired in terms of ozone offsets for UV impacts and protection of carbon sinks or GHG mitigation to offset the CO2 impacts (Sect. 3.3).
3.3 Climate forcing for GHGs
Offsets in carbon emissions are measured in tons of carbon dioxide equivalent (CO2 eq.) using GWPs from the most recently published set, i.e., Burkholder et al. (2022). The issue of timescales is also important with this metric, as the GWP involves a comparison of the cumulative climate impact over a specified time interval of a pulse emission for chemicals with different lifetimes. While 100-year GWPs are most commonly used to capture the longer-term warming effects of long-lived greenhouse gases like CO2 and CFCs, the use of a 20-year GWP may be more relevant when considering near-term warming impacts of potent but short-lived GHGs like most HFCs, such as 1,1,1,2-tetrafluoroethane (HFC-134a) (13.5-year lifetime), difluoromethane (HFC-32) (5.27-year lifetime), or pentafluoroethane (HFC-125) (30.7-year lifetime) (Burkholder et al., 2022). Such near-term impacts are particularly relevant to temperature goals such as limiting warming to 1.5 ∘C with no or limited overshoot, noting the possibility of crossing the 1.5 ∘C warming target of the Paris Agreement as soon as the 2030s (Abernethy and Jackson, 2022; Xu et al., 2018; Arias et al., 2021).
The GWP for a molecule x is given by Eq. (3):
where a is the radiative efficiency per unit mass, τx is the global lifetime of molecule x, R(t) is the decay time of a pulse of the reference gas CO2, and t′ is the time horizon over which the integrated radiative forcing is calculated (Daniel et al., 2012).
Table 1CFC-11 cumulative production in ODP-weighted, GWP-weighted, and calculated masses of HCFC-22, HCFC-141b, and HCFC-142b to achieve equivalent offsets. ODP and GWP values are from Table A-5 in the 2022 Quadrennial Ozone Assessment (Burkholder et al., 2022).
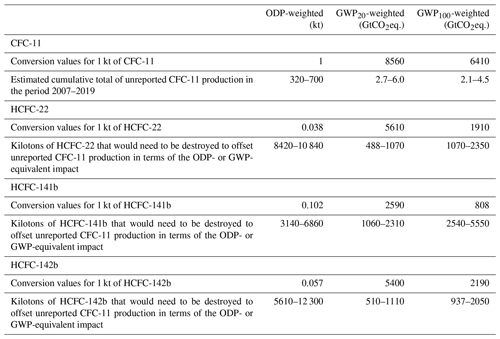
3.4 Illustrative offset calculation
When deriving offsets based on anomalies in emissions, it is important to remember to consider the potential for future emissions that have not yet escaped into the atmosphere (e.g., from banked chemicals that were produced illicitly but that have not yet reached the atmosphere; see the earlier text). In the case of the unexpected CFC-11 emissions, the TEAP Task Force found that “the estimated cumulative total of unreported CFC-11 production is 320–700 kt in the period 2007–2019. Assuming usage in closed-cell foam production, this cumulative unreported CFC-11 production would lead to an estimated increase in the magnitude of the CFC-11 bank of 300 (266–333) kilotons by the end of 2019” (UNEP/TEAP, 2022). Taking the cumulative total of unreported production of 320–700 kt CFC-11, we calculate an ODP-weighted emission of 320–700 kt, a GWP20 of 2.7–6.0 GtCO2eq., and a GWP100 of 2.1–4.5 GtCO2eq. (Table 1). To calculate equivalent offsets, the formula in Eq. (4) is considered below to derive offsets for three ODSs that are being phased out under the Montreal Protocol with the largest remaining eligible production and consumption (Table 1).
Offsetting the ozone depletion from the cumulative total CFC-11 production would require one to prevent emissions of 8420 to 10 840 kt of HCFC-22, either through the destruction of that amount from existing banks or as reduced production allowances. In this case, the amount of HCFC required to offset the cumulative ozone impacts is greater than the amount that would be needed to offset the global warming impacts under both 20- and 100-year time horizons. For comparison, the estimated cumulative HCFC-22 production allowed under the Montreal Protocol phaseout schedule for controlled uses (excluding feedstocks) through 2040 is on the order of 1300 kt (Table 2). Even if recent HCFC-22 production from 2021 to 2023 was considered available for recovery and destruction, this would only amount to about 900 kt available for offset. Due to the low ozone-depleting potential of HFCF-22, it would take the additional step of recovery and destruction of CFC banks or some other actions to offset the ozone impacts of the unexpected CFC-11 production. Elimination or prevention of remaining eligible consumption for 1,1-dichloro-1-fluoroethane (HCFC-141b) and 1-chloro-1,1-difluoroethane (HCFC-142b) would also be insufficient, as those magnitudes are estimated to be 1.3 and 7.6 kt, respectively, based on reporting through the 92nd meeting of the Executive Committee of the Multilateral Fund, as reported in Table 4-1 of the TEAP Replenishment Task Force Supplementary Report (UNEP/TEAP, 2023b). However, narrowing exemptions for production of ODSs for feedstock uses could expand the number of ODSs available for offset (see Table 2).
While any given instance of unexpected and unreported emissions may seem small in terms of atmospheric impacts, such impacts are cumulative and in absolute terms significant compared to other environmental violations where compensation is sought – consider for example the settlement requiring Volkswagen (VW) to provide nearly USD 3 billion to the Environmental Mitigation Trust to “fully remediate the excess NOx emissions from the illegal vehicles” (Breyer, 2016). Stratospheric ozone depletion and climate-forcing offsets can compensate for unexpected and unreported production by reducing production or emissions of an ODS produced legally prior to phaseout under the Montreal Protocol or by preventing emissions or production of an ODS not yet subject to the Montreal Protocol's phaseout requirements, e.g., trifluoromethyl iodide (CF3I), methylene chloride (CH2Cl2), or nitrous oxide (N2O), and/or with respect to climate forcing, avoiding cumulative emissions or removing GHGs equivalent to the near-term (20-year GWP) forcing of the unexpected and unreported emission to help prevent triggering tipping points and longer-term climate change (Lenton et al., 2019). In the absence of chemical offsets being applied, an alternative approach might be to calculate cumulative health impacts and determine monetary compensation and/or punitive damages of loss of health, life, productivity, ecological impact, and material degradation.
Offsets in ozone depletion are measured in tons of CFC-11 emission-equivalent (as an ODP-weighted emission). In the case of ozone depletion, the size of the offset estimated to be needed would ensure that the cumulative adverse impact of the unreported or illicit activity would be offset. Such an approach would contribute to ozone recovery and offsetting the health and environmental damage done prior to mitigation.
In Table 2 we present a non-exhaustive list of potential actions that could be used to offset the ozone depletion, climate, and other environmental impacts arising from instances of unexpected and unreported production that Parties to the Montreal Protocol may agree warrant remedial action. Any of these measures could also be employed to offset the ozone and climate impacts of Essential Use Exemptions (EUEs) for ODSs other than HCFCs and methyl bromide (including emergency EUEs) together with Critical Use Exemptions (CUEs) for methyl bromide (including emergency CUEs) (UNEP/TEAP, 2005).2 We provide indicative numbers on the potential available offsets for each type of action.
Others (WMO et al., 2021) have implicitly suggested the usefulness of offsets: “The recovery and destruction of CFC-11 banks would not only accelerate the ozone layer recovery, but would also yield climate benefits. Based on the TEAP/UNEP (2019b) scenario, recovery and destruction of the active and inactive banks would reduce emissions by 1.6 Gt CO2 eq. (GWP100, 2.2 GtCO2 eq. GWP20) between 2020 and 2060 and 2.6 Gt CO2 eq. (GWP100, by 3.6 GtCO2 eq. GWP20) between 2020 and 2100 (see Table 5.2). Using their estimates of much larger banks, Lickley et al. (2020) estimated that recovery and destruction of the CFC-11 and CFC-12 banks would reduce emissions by 9 Gt CO2 eq. (GWP100, 13 Gt GtCO2 eq. GWP20) between 2020 and 2100.” Recent analysis by Lickley et al. (2022) suggests that production may have been underreported for nearly all the chemicals examined, implying larger banks, and they conclude that, “in terms of climate impacts, CFC-11, CFC-12, and HCFC-22 are the largest banked materials weighted by GWP100, accounting for 36 %, 14 %, and 36 % of current [ODS] banks, respectively. When banks are weighted by ODP, CFC-11 and CFC-12 represent 46 % and halons also represent 46 % of current banked chemicals … In terms of GWP100, CFC-11 banks largely reside in foams, whereas CFC-12 and HCFC-22 are largely in non-hermetic refrigeration. The latter may be more readily recoverable. In terms of ODP, CFC-11 foams and CFC-12 non-hermetic refrigeration remain important, along with halons which are all contained in fire extinguishers, a recoverable reservoir”.3
This technical note describes approaches for offsetting the ozone depletion and climate forcing from unexpected and unreported production and associated emissions of ozone-depleting substances (ODSs) (e.g., CFC-11, CFC-12, CFC-113, and CTC). Scientists can calculate the contribution of each option in offsetting potentially both the annual and cumulative ozone depletion and climate forcing over the atmospheric lifetime of the ODSs, but we argue here that typically the most practical approach (and in some instances the only approach) will be to consider offsetting cumulative impacts without consideration of timing. The Montreal Protocol Parties have shown creativity and flexibility in their non-compliance remedies (UNEP, 1992). Parties to the Montreal Protocol may wish to consider action on compliance to minimize ozone and climate consequences and to discourage future unexpected and unreported production.
All the data and methods are provided in the paper.
The supplement related to this article is available online at: https://doi.org/10.5194/acp-24-2023-2024-supplement.
SOA conceptualized the study. GBD, SAM, SOA, and RF wrote the paper.
The contact author has declared that none of the authors has any competing interests.
Publisher’s note: Copernicus Publications remains neutral with regard to jurisdictional claims made in the text, published maps, institutional affiliations, or any other geographical representation in this paper. While Copernicus Publications makes every effort to include appropriate place names, the final responsibility lies with the authors.
This article is part of the special issue “Atmospheric ozone and related species in the early 2020s: latest results and trends (ACP/AMT inter-journal SI)”. It is a result of the 2021 Quadrennial Ozone Symposium (QOS) held online on 3–9 October 2021.
The authors thank the colleagues who helped identify the policy-relevant science and the opportunities to offset ODS and HFC emissions. We are particularly grateful to John S. Daniel, David W. Fahey, Korey G. Silverman-Roati, and Durwood Zaelke. Gabrielle B. Dreyfus, Stephen O. Andersen, and Richard Ferris acknowledge support from the Children's Investment Fund Foundation.
This research has been supported by the Children's Investment Fund Foundation.
This paper was edited by Tanja Schuck and reviewed by two anonymous referees.
Abernethy, S. and Jackson, R. B.: Global temperature goals should determine the time horizons for greenhouse gas emission metrics, Environ. Res. Lett., 17, 024019, https://doi.org/10.1088/1748-9326/ac4940, 2022.
Adcock, K. E., Ashfold, M. J., Chou, C. C.-K., Gooch, L. J., Mohd Hanif, N., Laube, J. C., Oram, D. E., Ou-Yang, C.-F., Panagi, M., Sturges, W. T., and Reeves, C. E.: Investigation of East Asian Emissions of CFC-11 Using Atmospheric Observations in Taiwan, Environ. Sci. Technol., 54, 3814–3822, https://doi.org/10.1021/acs.est.9b06433, 2020.
Andersen, S. O. and Sarma, K. M.: Protecting the Ozone Layer: The United Nations History, Earthscan Press (official publication of the United Nations Environment Programme), London, England, 513 pp., https://doi.org/10.4324/9781849772266, 2002.
Andersen, S. O., Gao, S., Carvalho, S., Ferris, T., Gonzalez, M., Sherman, N. J., Wei, Y., and Zaelke, D.: Narrowing feedstock exemptions under the Montreal Protocol has multiple environmental benefits, P. Natl. Acad. Sci. USA, 118, e2022668118, https://doi.org/10.1073/pnas.2022668118, 2021.
Arias, P. A., Bellouin, N., Coppola, E. et al.: Technical Summary. in: Climate Change 2021: The Physical Science Basis. Contribution of Working Group I to the Sixth Assessment Report of the Intergovernmental Panel on Climate Change, edited by: Masson-Delmotte, V., Zhai, P., Pirani, A. et al., Cambridge University Press, Cambridge, United Kingdom and New York, NY, USA, 33−-144, https://doi.org/10.1017/9781009157896.002, 2021.
Bais, A. F., Lucas, R. M., Bornman, J. F., Williamson, C. E., Sulzberger, B., Austin, A. T., Wilson, S. R., Andrady, A. L., Bernhard, G., McKenzie, R. L., Aucamp, P. J., Madronich, S., Neale, R. E., Yazar, S., Young, A. R., de Gruijl, F. R., Norval, M., Takizawa, Y., Barnes, P. W., Robson, T. M., Robinson, S. A., Bailaré, C. L., Flint, S. D., Neale, P. J., Hylander, S., Rose, K. C., Wängberg, S.-Å., Hader, D.-P., Worrest, R. C., Zepp, R. G., Paul, N. D., Cory, R. M., Solomon, K. R., Longstreth, J., Pandey, K. K., Redhwi, H. H., Torikai, A., and Heikkilä, A. M.: Environmental effects of ozone depletion, UV radiation and interactions with climate change: UNEP Environmental Effects Assessment Panel, update 2017, Photochem. Photobiol. Sci., 17, 127–179, https://doi.org/10.1039/c7pp90043k, 2018.
Benish, S. E., Salawitch, R. J., Ren, X., He, H., and Dickerson, R. R.: Airborne Observations of CFCs Over Hebei Province, China in Spring 2016, J. Geophys. Res.-Atmos., 126, e2021JD035152, https://doi.org/10.1029/2021JD035152, 2021.
Breyer, C. R.: Volkswagen “Clean Diesel” Marketing, Sales Practices, and Products Liability Litigation, https://www.cand.uscourts.gov/judges/breyer-charles-r-crb/in-re-volkswagen-clean-diesel-mdl/ (last access: 1 February 2024), 2016.
Burkholder, J. B., Hodnebrog, Ø., McDonald, B. C., Orkin, V., Papadimitriou, V. C., and Van Hoomissen, D.: Annex: Summary of Abundances, in: Scientific Assessment of Ozone Depletion: 2022, World Meteorological Organization, Geneva, https://ozone.unep.org/sites/default/files/2023-02/Scientific-Assessment-of-Ozone-Depletion-2022.pdf (last access: 8 February 2023), 2022.
Daniel, J. S., Solomon, S., Sanford, T. J., McFarland, M., Fuglestvedt, J. S., and Friedlingstein, P.: Limitations of single-basket trading: lessons from the Montreal Protocol for climate policy, Climatic Change, 111, 241–248, https://doi.org/10.1007/s10584-011-0136-3, 2012.
Daniel, J. S., Reimann, S., Ashford, P., Fleming, E. L., Hossaini, R., Lickley, M. J., Schofield, R., Walter-Terrinoni, H., McBride, L., Park, S., Ross, M. N., Salawitch, R. J., Sherry, D., Tegtmeier, S., and Velders, G. J. M.: Chapter 7: Scenarios and Information for Policymakers, in: Scientific Assessment of Ozone Depletion: 2022, World Meteorological Organization, Geneva, https://ozone.unep.org/sites/default/files/2023-02/Scientific-Assessment-of-Ozone-Depletion-2022.pdf (last access: 8 February 2023), 2022.
Davidson, E. A. and Winiwarter, W.: Urgent abatement of industrial sources of nitrous oxide, Nat. Clim. Change, 13, 599–601, https://doi.org/10.1038/s41558-023-01723-3, 2023.
Dhomse, S. S., Feng, W., Montzka, S. A., Hossaini, R., Keeble, J., Pyle, J. A., Daniel, J. S., and Chipperfield, M. P.: Delay in recovery of the Antarctic ozone hole from unexpected CFC-11 emissions, Nat. Commun., 10, 5781, https://doi.org/10.1038/s41467-019-13717-x, 2019.
Fisher, D. A., Hales, C. H., Filkin, D. L., Ko, M. K. W., Sze, N. D., Connell, P. S., Wuebbles, D. J., Isaksen, I. S. A., and Stordal, F.: VIII. Ozone Depletion Potentials, Relative Effects on Stratospheric Ozone of Halogenated Methanes and Ethanes of Social and Industrial Interest, in: Scientific Assessment of Stratospheric Ozone, 1989, vol. 2, World Meteorological Organization, Global Ozone Research and Monitoring Project], Geneva, Switzerland, 303–377, https://csl.noaa.gov/assessments/ozone/1989/vol2/section8.pdf (last access: 7 August 2023), 1990.
Fleming, E. L., Newman, P. A., Liang, Q., and Daniel, J. S.: The Impact of Continuing CFC-11 Emissions on Stratospheric Ozone, J. Geophys. Res.-Atmos., 125, e2019JD031849, https://doi.org/10.1029/2019JD031849, 2020.
Keeble, J., Abraham, N. L., Archibald, A. T., Chipperfield, M. P., Dhomse, S., Griffiths, P. T., and Pyle, J. A.: Modelling the potential impacts of the recent, unexpected increase in CFC-11 emissions on total column ozone recovery, Atmos. Chem. Phys., 20, 7153–7166, https://doi.org/10.5194/acp-20-7153-2020, 2020.
Lenton, T. M., Rockström, J., Gaffney, O., Rahmstorf, S., Richardson, K., Steffen, W., and Schellnhuber, H. J.: Climate tipping points – too risky to bet against, Nature, 575, 592–595, https://doi.org/10.1038/d41586-019-03595-0, 2019.
Liang, Q., Rigby, M., Fang, X., Godwin, D., Mühle, J., Saito, T., Stanley, K. M., Velders, G. J. M., Bernath, P., Derek, N., Reimann, S., Simpson, I. J., and Western, L.: Chapter 2: Hydrofluorocarbons (HFCs), in: Scientific Assessment of Ozone Depletion: 2022, World Meteorological Organization, Geneva, https://ozone.unep.org/sites/default/files/2023-02/Scientific-Assessment-of-Ozone-Depletion-2022.pdf (last access: 8 February 2023), 2022.
Lickley, M., Solomon, S., Fletcher, S., Velders, G. J. M., Daniel, J., Rigby, M., Montzka, S. A., Kuijpers, L. J. M., and Stone, K.: Quantifying contributions of chlorofluorocarbon banks to emissions and impacts on the ozone layer and climate, Nat. Commun., 11, 1380, https://doi.org/10.1038/s41467-020-15162-7, 2020.
Lickley, M., Fletcher, S., Rigby, M., and Solomon, S.: Joint inference of CFC lifetimes and banks suggests previously unidentified emissions, Nat. Commun., 12, 2920, https://doi.org/10.1038/s41467-021-23229-2, 2021.
Lickley, M. J., Daniel, J. S., Fleming, E. L., Reimann, S., and Solomon, S.: Bayesian assessment of chlorofluorocarbon (CFC), hydrochlorofluorocarbon (HCFC) and halon banks suggest large reservoirs still present in old equipment, Atmos. Chem. Phys., 22, 11125–11136, https://doi.org/10.5194/acp-22-11125-2022, 2022.
Longstreth, J., de Gruijl, F. R., Kripke, M. L., Abseck, S., Arnold, F., Slaper, H. I., Velders, G., Takizawa, Y., and van der Leun, J. C.: Health risks, J. Photochem. Photobiol. B, 46, 20–39, https://doi.org/10.1016/S1011-1344(98)00183-3, 1998.
Madronich, S., Lee-Taylor, J. M., Wagner, M., Kyle, J., Hu, Z., and Landolfi, R.: Estimation of Skin and Ocular Damage Avoided in the United States through Implementation of the Montreal Protocol on Substances that Deplete the Ozone Layer, ACS Earth Space Chem., 5, 1876–1888, https://doi.org/10.1021/acsearthspacechem.1c00183, 2021.
Montzka, S. A., Dutton, G. S., Yu, P., Ray, E., Portmann, R. W., Daniel, J. S., Kuijpers, L., Hall, B. D., Mondeel, D., Siso, C., Nance, J. D., Rigby, M., Manning, A. J., Hu, L., Moore, F., Miller, B. R., and Elkins, J. W.: An unexpected and persistent increase in global emissions of ozone-depleting CFC-11, Nature, 557, 413–417, https://doi.org/10.1038/s41586-018-0106-2, 2018.
Montzka, S. A., Dutton, G. S., Portmann, R. W., Chipperfield, M. P., Davis, S., Feng, W., Manning, A. J., Ray, E., Rigby, M., Hall, B. D., Siso, C., Nance, J. D., Krummel, P. B., Mühle, J., Young, D., O'Doherty, S., Salameh, P. K., Harth, C. M., Prinn, R. G., Weiss, R. F., Elkins, J. W., Walter-Terrinoni, H., and Theodoridi, C.: A decline in global CFC-11 emissions during 2018–2019, Nature, 590, 428–432, https://doi.org/10.1038/s41586-021-03260-5, 2021.
Park, S., Western, L. M., Saito, T., Redington, A. L., Henne, S., Fang, X., Prinn, R. G., Manning, A. J., Montzka, S. A., Fraser, P. J., Ganesan, A. L., Harth, C. M., Kim, J., Krummel, P. B., Liang, Q., Mühle, J., O'Doherty, S., Park, H., Park, M.-K., Reimann, S., Salameh, P. K., Weiss, R. F., and Rigby, M.: A decline in emissions of CFC-11 and related chemicals from eastern China, Nature, 590, 433–437, https://doi.org/10.1038/s41586-021-03277-w, 2021.
Prather, M. J.: Lifetimes of atmospheric species: Integrating environmental impacts: Atmospheric Lifetimes & Integrated Effects, Geophys. Res. Lett., 29, 20-1–20-3, https://doi.org/10.1029/2002GL016299, 2002.
Pyle, J. A., Keeble, J., Abraham, N. L., Chipperfield, M. P., and Griffiths, P. T.: Integrated ozone depletion as a metric for ozone recovery, Nature, 608, 719–723, https://doi.org/10.1038/s41586-022-04968-8, 2022.
Ramanathan, V.: Greenhouse Effect Due to Chlorofluorocarbons: Climatic Implications, Science, 190, 50–52, https://doi.org/10.1126/science.190.4209.50, 1975.
Rigby, M., Park, S., Saito, T., Western, L. M., Redington, A. L., Fang, X., Henne, S., Manning, A. J., Prinn, R. G., Dutton, G. S., Fraser, P. J., Ganesan, A. L., Hall, B. D., Harth, C. M., Kim, J., Kim, K.-R., Krummel, P. B., Lee, T., Li, S., Liang, Q., Lunt, M. F., Montzka, S. A., Mühle, J., O'Doherty, S., Park, M.-K., Reimann, S., Salameh, P. K., Simmonds, P., Tunnicliffe, R. L., Weiss, R. F., Yokouchi, Y., and Young, D.: Increase in CFC-11 emissions from eastern China based on atmospheric observations, Nature, 569, 546–550, https://doi.org/10.1038/s41586-019-1193-4, 2019.
Slaper, H., Velders, G. J. M., Daniel, J. S., de Gruijl, F. R., and van der Leun, J. C.: Estimates of ozone depletion and skin cancer incidence to examine the Vienna Convention achievements, Nature, 384, 256–258, https://doi.org/10.1038/384256a0, 1996.
SPARC: SPARC Report on the Mystery of Carbon Tetrachloride, edited by: Liang, Q., Newman, P. A., and Reimann, S., SPARC Report No. 7, WCRP-13/2016, https://doi.org/10.3929/ethz-a-010690647, 2016.
Struijs, J., van Dijk, A., Slaper, H., van Wijnen, H. J., Velders, G. J. M., Chaplin, G., and Huijbregts, M. A. J.: Spatial- and Time-Explicit Human Damage Modeling of Ozone Depleting Substances in Life Cycle Impact Assessment, Environ. Sci. Technol., 44, 204–209, https://doi.org/10.1021/es9017865, 2010.
UNEP: Annex V: Indicative list of measures that might be taken by a Meeting of the Parties in respect of non-compliance with the Protocol, https://ozone.unep.org/node/2080 (last access: 12 December 2022), 1992.
UNEP: Agenda item 5: Country programme data and prospects for compliance, UNEP [data set], http://www.multilateralfund.org/92/pages/English.aspx (last access: 9 August 2023), 2023a.
UNEP: Consumption of controlled substances: Country Data for European Union, UNEP [data set], https://ozone.unep.org/countries/data?report_type=0&party[0]=65&group[0]=10&period_start=1986&period_end=2022&output_type=odp-CO2e-tonnes, last access: 28 November 2023b.
UNEP/EEAP: 2018 Assessment Report of the Environment Effects Assessment Panel (EEAP): Environmental Effects and Interactions of Stratospheric Ozone Depletion, UV Radiation, and Climate Change, Nairobi, Kenya, https://ozone.unep.org/science/assessment/eeap (last access: 1 February 2024), 2019.
UNEP/TEAP: Handbook on Essential Use Nominations, Nairobi, Kenya, https://ozone.unep.org/sites/default/files/2019-05/EUN-Handbook2005 (1).pdf (last access: 1 February 2024), 2005.
UNEP/TEAP: Volume 1: Decision XXX/3 TEAP Task Force Report on Unexpected Emissions of Trichlorofluoromethane (CFC-11), September 2019, Nairobi, Kenya, https://ozone.unep.org/system/files/documents/TEAP-TF-DecXXX-3-unexpected_CFC11_emissions-september2019.pdf (last access: 10 August 2023), 2019.
UNEP/TEAP: Volume 3 Decision XXXIII/5 – Continued Provision of Information on Energy-Efficient and Low-Global-Warming-Potential Technologies, May 2022, Nairobi, Kenya, https://ozone.unep.org/system/files/documents/TEAP-EETF-report-may-2022.pdf (last access: 14 March 2023), 2022.
UNEP/TEAP: Volume 1: Progress Report, Supplementary Report, Decision XXXIV/3 Energy Efficiency Working Group Report, May 2023, Nairobi, Kenya, https://ozone.unep.org/system/files/documents/TEAP-May2023-Progress-Report-Supplementary.pdf, (last access: 10 August 2023), 2023a.
UNEP/TEAP: Volume 7: Supplement to the May 2023 TEAP Replenishment Task Force Report “Assessment of the Funding Requirement for the Replenishment of the Multilateral Fund for the Period 2024–2026”, September 2023, Nairobi, Kenya, https://ozone.unep.org/system/files/documents/TEAP-Decision-XXXIV-2 RTF-supplementary-report-september2023.pdf, last access: 28 November 2023b.
UNEP/TEAP: Volume 3: Assessment of the funding requirement for the replenishment of the multilateral fund for the period 2024–2026, May 2023, Nairobi, Kenya, https://ozone.unep.org/system/files/documents/TEAP-DecisionXXXIV2-replenishment-TF-report-May2023-RTF-report.pdf (last access: 1 February 2024), 2023c.
US EPA: Phasedown of Hydrofluorocarbons: Adjustment to the Hydrofluorocarbon Production Baseline, https://www.federalregister.gov/documents/2023/07/12/2023-14189/phasedown-of-hydrofluorocarbons-adjustment-to-the-hydrofluorocarbon-production-baseline, last access: 7 August 2023.
US EPA: Updating the Atmospheric and Health Effects Framework Model: Stratospheric Ozone Protection and Human Health Benefits, Stratospheric Protection Division, Office of Air and Radiation, Washington, D.C., https://www.epa.gov/sites/default/files/2020-04/documents/2020_ahef_report.pdf (last access: 22 December 2022), 2020.
van Dijk, A., Slaper, H., den Outer, P. N., Morgenstern, O., Braesicke, P., Pyle, J. A., Garny, H., Stenke, A., Dameris, M., Kazantzidis, A., Tourpali, K., and Bais, A. F.: Skin cancer risks avoided by the Montreal Protocol–worldwide modeling integrating coupled climate-chemistry models with a risk model for UV, Photochem. Photobiol., 89, 234–246, https://doi.org/10.1111/j.1751-1097.2012.01223.x, 2013.
Western, L. M., Vollmer, M. K., Krummel, P. B., Adcock, K. E., Fraser, P. J., Harth, C. M., Langenfelds, R. L., Montzka, S. A., Mühle, J., O'Doherty, S., Oram, D. E., Reimann, S., Rigby, M., Vimont, I., Weiss, R. F., Young, D., and Laube, J. C.: Global increase of ozone-depleting chlorofluorocarbons from 2010 to 2020, Nat. Geosci., 16, 309–313, https://doi.org/10.1038/s41561-023-01147-w, 2023.
World Meteorological Organization (WMO): Scientific Assessment of Ozone Depletion: 2022, WMO, Geneva, 509 pp., https://ozone.unep.org/sites/default/files/2023-02/Scientific-Assessment-of-Ozone-Depletion-2022.pdf (last access: 1 February 2024), 2022.
Xu, Y., Ramanathan, V., and Victor, D. G.: Global warming will happen faster than we think, Nature, 564, 30–32, https://doi.org/10.1038/d41586-018-07586-5, 2018.
World Meteorological Organization (WMO), United Nations Environment Programme (UNEP), National Aeronautics and Space Administration (NASA), and National Oceanic and Atmospheric Administration (NOAA): Report on the Unexpected Emissions of CFC-11: A Report of the Scientific Assessment Panel of the Montreal Protocol on Substances that Deplete the Ozone Layer, Geneva, Switzerland, https://ozone.unep.org/system/files/documents/SAP-2021-report-on-the-unexpected-emissions-of-CFC-11-1268_en.pdf (last access: 1 February 2024), 2021.
Young, P. J., Harper, A. B., Huntingford, C., Paul, N. D., Morgenstern, O., Newman, P. A., Oman, L. D., Madronich, S., and Garcia, R. R.: The Montreal Protocol protects the terrestrial carbon sink, Nature, 596, 384–388, https://doi.org/10.1038/s41586-021-03737-3, 2021.
This estimate is based on the “bank scenario”, which assumes that CFC-11 emissions began increasing in 2012 above those expected under the reference WMO A1 scenario, peak around 77 Gg yr−1 in 2015–2017, and then decline sharply through 2100. While the end date of unreported production is unclear, the report states that “In the fourth scenario, CFC-11 emissions were estimated based on Dhomse et al. (2019), which constructs an emissions scenario curve based on initial rapid increase in CFC-11 emissions and slower release from accumulated CFC-11 banks. This scenario first takes the estimate of 13 Gg yr−1 in new emissions due to unreported production and assumes an immediate production release rate of 15 % followed by 3.5 % yr−1. This creates a gradually decreasing emissions curve where CFC-11 emissions continue past 2100 due to releases from the accumulated bank even after production goes to zero” (US EPA, 2020).
Numerous decisions of the Parties to the Montreal Protocol establish and interpret criteria and procedures for EUEs and CUEs, together with related emergency exemption applications. Examples of key decisions concerning EUEs and CUEs are provided in the references.
If an alternative is identified for those essential uses preserving health and safety, halons could be a recoverable reservoir.
- Abstract
- The stratospheric ozone layer and the Montreal Protocol
- Usage implications for estimating the magnitude of impacts to be offset
- Quantifying ozone depletion, environmental, and climate impacts
- Offsets are one option to maintain the integrity of ozone and climate protection under the Montreal Protocol
- Potential actions that could offset the ozone depletion and climate impacts of unexpected and unreported production
- Conclusion
- Data availability
- Author contributions
- Competing interests
- Disclaimer
- Special issue statement
- Acknowledgements
- Financial support
- Review statement
- References
- Supplement
- Abstract
- The stratospheric ozone layer and the Montreal Protocol
- Usage implications for estimating the magnitude of impacts to be offset
- Quantifying ozone depletion, environmental, and climate impacts
- Offsets are one option to maintain the integrity of ozone and climate protection under the Montreal Protocol
- Potential actions that could offset the ozone depletion and climate impacts of unexpected and unreported production
- Conclusion
- Data availability
- Author contributions
- Competing interests
- Disclaimer
- Special issue statement
- Acknowledgements
- Financial support
- Review statement
- References
- Supplement