the Creative Commons Attribution 4.0 License.
the Creative Commons Attribution 4.0 License.
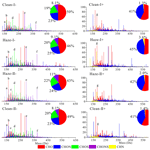
Measurement report: Changes in light absorption and molecular composition of water-soluble humic-like substances during a winter haze bloom-decay process in Guangzhou, China
Chunlin Zou
Tao Cao
Meiju Li
Jianzhong Song
Bin Jiang
Wanglu Jia
Xiang Ding
Zhiqiang Yu
Gan Zhang
Ping'an Peng
Water-soluble humic-like substances (HULIS) absorb light in near-UV and visible wavelengths and exert significant influence on the atmospheric environment and climate. However, knowledge on HULIS evolution during haze bloom-decay process is limited. Herein, PM2.5 samples were obtained during a winter haze event in Guangzhou, China, and the light absorption and molecular composition of HULIS were investigated by UV–Vis spectrophotometry and ultrahigh-resolution mass spectrometry. Compared with HULIS on clean days, the absorption coefficients (Abs365) of HULIS on haze days were significantly higher but the mass absorption efficiencies (MAE365) were relatively low, suggesting diverse and dynamic absorption properties of HULIS during haze episodes. The CHO and CHON compounds were the most abundant components in HULIS, followed by CHOS, CHONS, and CHN. Haze HULIS presented comparatively high molecular weight; a lower aromaticity index (AImod); and higher , , and ratios, indicating that HULIS fractions undergo relatively high oxidation during haze days compared to clean days. Moreover, CHON and CHO compounds with high AImod were the major potential chromophores in HULIS and significantly contributed to HULIS light absorption. It is worth noting that the proportions of these chromophores decreased during haze events, mainly owing to their higher oxidation during haze episodes. Besides, accumulated contribution of organic compounds emitted from vehicles and formed from reactions of biogenic volatile organic compounds (bio-VOCs) also diluted light-absorbing compounds in haze HULIS. These findings help us to understand HULIS evolution during haze bloom-decay processes in the subtropic region of China.
- Article
(5103 KB) -
Supplement
(1026 KB) - BibTeX
- EndNote
Water-soluble humic-like substances (HULIS), belonging to a class of highly complex organic compounds with physical and chemical properties similar to humic substances in natural aquatic/soil environments, constitute 30 %–70 % of water-soluble organic compounds in ambient aerosols and are responsible for >70 % of light absorption (at 365 nm) in water-soluble brown carbon (BrC) (Graber and Rudich, 2006; Laskin et al., 2015; Huang et al., 2018). They are thought to be comprised of aromatic structures containing aliphatic side chains and oxygenated functional groups such as hydroxyl, carboxyl, nitrate, and organosulfate groups (Lin et al., 2012; Song et al., 2018; Zeng et al., 2020). HULIS are ubiquitously identified in atmospheric aerosols, fog, cloud, and rainwater and have been demonstrated to have significant effects on both the atmospheric environment and climate (Bianco et al., 2018; Wu et al., 2018; Zhan et al., 2022). In addition, HULIS exert adverse health effects because they can enhance the oxidative potential of organic aerosols (Ma et al., 2019; Cao et al., 2021).
In recent years, severe particulate pollution (i.e., haze events) has frequently occurred in some developing countries such as China, which has drawn extensive public and scientific concerns (An et al., 2019; Zhang et al., 2020). According to An et al. (2019), contributions of organic aerosols, including primary organic aerosols and secondary organic aerosols (SOA), are significant for severe haze events; in particular, the contribution of SOA in China is expected to continuously increase because of stronger chemical reactions in the atmosphere. HULIS are an important component in organic aerosols, which originate from a variety of primary emissions (e.g., biomass burning (BB), coal combustion, off-road engine emissions) (Fan et al., 2016; Cui et al., 2019; Tang et al., 2020) and secondary chemical oxidation of biogenic and anthropogenic volatile organic compounds (VOCs) (Yu et al., 2016; Tomaz et al., 2018) and soot (Fan et al., 2020). During haze episodes, a number of chemical processes occur in the aqueous phase (Wong et al., 2017; Wu et al., 2018) and gas phase (Sumlin et al., 2017), which lead to significant changes in the chemical composition and light absorption properties of HULIS. For instance, recent studies on oxidation of BB-derived BrC have indicated that although both enhancement and bleaching of BrC occur during aging, bleaching of BrC becomes dominant over a long period (Fan et al., 2020; Wong et al., 2017; Ni et al., 2021). However, multiphase reaction between carbonyl and amine has demonstrated rapid formation of light-absorbing organic compounds (Kampf et al., 2016). Nevertheless, it should be noted that these results were mainly obtained from laboratory experiments and may not reflect the complex evolution behavior of BrC in atmospheric environments.
High concentrations of HULIS have been determined during typical haze episodes in northern, eastern, and southern China (Fan et al., 2016; Zhang et al., 2020; Wang et al., 2020) and have been demonstrated to significantly influence atmospheric visibility, the environment, and photochemical processes. Guangzhou is the biggest city in the Pearl River Delta (PRD), one of the most developed regions in China, and is located in the subtropical zone with a population of over 18 million people (Yu et al., 2017). Although a remarkable decline in atmospheric particulate matter (PM2.5) pollution has been observed in recent years owing to strict regulatory controls, O3 and VOCs still remain at higher levels and severe haze pollution caused by fine particulate matter frequently occurs in winter (An et al., 2019; K. Li et al., 2019; Yang et al., 2022). Several studies have investigated the optical, chemical, and molecular properties of HULIS in the PRD region (Lin et al., 2010, 2012; Fan et al., 2016; Liu et al., 2018; Jiang et al., 2020, 2021). For example, studies on the temporal variations in water-soluble HULIS in Guangzhou have indicated that HULIS had higher concentrations and mass absorption efficiencies (MAE365) in the winter, which were attributed to the increasing contribution of BB and secondary nitrate formation in the winter monsoon period (Fan et al., 2016; Jiang et al., 2020, 2021). In addition, the molecular composition of HULIS (and BrC) in the PRD region was also investigated, and it was demonstrated that the levels of unsaturated and aromatic structures comprise the important factor influencing their light absorption properties (Jiang et al., 2020). However, detailed information regarding the evolution of light absorption and molecular composition of HULIS during haze events is still scarce.
Recently, ultrahigh-resolution Fourier transform ion cyclotron resonance mass spectrometry (FT-ICR MS) coupled with electrospray ionization (ESI) sources has been frequently employed to investigate the molecular characteristics of HULIS in ambient aerosols (Song et al., 2018, 2022; Tang et al., 2020; Zeng et al., 2020). Owing to its extremely high mass resolution and accuracy, this technique allows further exploration of the evolution of HULIS during haze events. The present study performed comprehensive characterization of HULIS in PM2.5 collected during a haze event in Guangzhou, China. The abundances and light absorption properties of HULIS were first measured, and carbonaceous fractions, water-soluble ions, and levoglucosan (Lev) were determined. Subsequently, four HULIS samples collected during different haze stages were analyzed using FT-ICR MS operated in both ESI− and ESI+ modes. To the best of our knowledge, the present study is the first to apply a combination of optical properties and molecular characterization by FT-ICR MS to investigate HULIS in a haze event in the subtropical zone of China. The results obtained provide novel insights into the evolution of HULIS during haze events and are important for predicting the environmental and climatic effects of HULIS in southern China.
2.1 Aerosol sampling
The PM2.5 samples were collected on the campus of Guangzhou Institute of Geochemistry, Chinese Academy of Sciences, Guangzhou, China (23.14∘ N, 113.35∘ E), which is an academic and residential region. Traffic emissions and residential activities are the potential pollution sources in the sampling area. The 24 h PM2.5 sampling was conducted using a high-volume sampler (Tianhong Intelligent Instrument Plant, Wuhan, China, with a flow rate of 1.0 m3 min−1) during 7 to 30 January 2018, and a total of 24 samples were collected on the prebaked quartz filters (20.3 cm×25.4 cm, Whatman, Maidstone, UK). Field blank samples were collected by keeping a blank filter in the sampler without pumping air. Before sampling, the filters were wrapped in aluminum foil and prebaked at 450 ∘C for 6 h to remove carbonaceous impurities. Before and after sampling, the filters were weighed at 25 ∘C and 50 % RH on a microbalance (Sartorius Model BP210D). The PM2.5 concentrations were determined by weighing the filters before and after collection. Finally, all filter samples were stored in a refrigerator at −20 ∘C until analysis. The mass accuracy achieved was <2 % based on triplicate analyses of filter samples. Meteorological data (http://www.wunderground.com/history/airport/ZGGG, last access: 10 March 2020), including wind speed; temperature; relative humidity; and concentrations of SO2, O3, and NO2, for the sampling days are presented in Fig. 1 and Table S1 in the Supplement.
2.2 Isolation of HULIS
HULIS were isolated using a water extraction and solid-phase extraction (SPE) procedure as described previously (Zou et al., 2020). This method has been used in most previous studies because of its easy operation and high reliability and reproducibility and low limit of detection (Fan et al., 2012); therefore, it was also used in this study. Briefly, portions of the PM2.5 samples (100 cm2) were ultrasonically extracted with 50 mL of ultrapure water for 30 min. The extracts were filtered through a 0.22 µm PTFE syringe filter to remove the suspended insoluble particles. About 50 mL of water extracts was obtained from each sample, of which 20 mL was used for the isolation and analysis of HULIS, 20 mL for analysis of water-soluble organic carbon (WSOC), and the remaining extracts for the analysis of inorganic ions. Then, the 20 mL water extracts were adjusted to a pH of 2 with HCl and loaded on a preconditioned SPE cartridge (Oasis HLB, 200 mg, 6 mL, Waters, USA). The hydrophilic fraction (i.e., inorganic ions, high-polarity organic acids, etc.) was removed with ultrapure water, whereas the relatively hydrophobic HULIS fraction was retained and eluted with 2 % () ammonia/methanol. Finally, HULIS solution was evaporated to dryness with a gentle N2 stream and redissolved with ultrapure water for the analysis.
It is noted that the HULIS here is the hydrophobic portion of water-soluble organic matter, which can be isolated with different types of SPE columns (e.g., HLB, C-18, DEAE, XAD-8, and PPL) (Fan et al., 2012, 2013; Lin et al., 2012; Zou et al., 2020; Jiang et al., 2020). Although each resin type has its special chemical properties, the hydrophobic HULIS isolated with different sorbents were similar in chemical, molecular properties based on previous studies (Fan et al., 2012, 2013; Zou et al., 2020). Therefore, for better comparison with other studies, the hydrophobic fractions isolated by SPE methods were all termed as HULIS in the present paper.
2.3 Light absorption analysis
The absorption spectra of the WSOC and HULIS fractions were measured by a UV–Vis spectrophotometer (UV-2600, Shimadzu) between 200 and 700 nm. Each spectrum was corrected for the filter blanks. The light absorption coefficients, absorption Ångström exponent (AAE), and mass absorption efficiency (MAEλ) were calculated, and the detailed methods for this are presented in the Supplement.
2.4 Chemical analysis
For FT-ICR MS analysis, the HULIS samples were isolated from PM2.5 collected during four periods: before haze days (clean-I days, 7–12 January), haze bloom days (haze-I days, 13–18 January), haze decay days (haze-II days, 19–24 January), and after haze days (clean-II days, 25–30 January). A filter punch (18 cm in diameter) was taken from every sample, and all six samples in each period were combined for the isolation of HULIS fractions. The obtained HULIS samples were measured with an ESI FT-ICR MS instrument (Bruker Daltonics GmbH, Bremen, Germany) equipped with a 9.4 T refrigerated actively shielded superconducting magnet. The system was operated in both ESI− and ESI+ modes. The scan range was set to from 100 to 1000, with a typical mass-resolving power >450 000 at 319 with <0.2 ppm absolute mass error. The mass spectra were calibrated externally with arginine clusters and internally recalibrated with typical O5-class species peaks in DataAnalysis 4.4 (Bruker Daltonics). Due to the inherent differences in the ionization mechanisms between ESI− and ESI+ modes, the data detected by the two ionization modes can provide complementary information on the molecular composition of atmospheric HULIS (Lin et al., 2012, 2018). The details of data analysis are provided in the Supplement.
The amounts of organic carbon (OC) and elemental carbon (EC) were determined by an OC–EC analyzer (Sunset Laboratory Inc., USA) (Mo et al., 2018). The concentrations of WSOC and HULIS were determined by a total organic carbon (TOC) analyzer (Shimadzu TOC-VCPH, Kyoto, Japan). The water-soluble inorganic species (, , Cl−, , K+, Na+, Ca2+, Mg2+) were measured with a Dionex ICS-900 ion chromatography system (Thermo Fisher Scientific, USA) as described previously (Huang et al., 2018). The concentrations of Lev were analyzed with gas chromatography–MS after derivatization with N,O-bis(trimethylsilyl)trifluoroacetamide and pyridine at 70 ∘C for 3 h (Huang et al., 2018). Detailed information regarding these measurements is provided in the Supplement.
3.1 Abundance and chemical composition of PM2.5
Figure 1 shows the meteorological conditions, PM2.5 concentration, and concentrations of major chemical constituents, including carbon fractions and water-soluble inorganic ions in PM2.5 samples obtained during a haze bloom-decay process. Based on the variation in PM2.5 concentration, these samples were categorized into four groups: clean-I days (before haze, 14–24 µg m−3), haze-I days (haze bloom, 45–114 µg m−3), haze-II days (haze decay, 58–115 µg m−3), and clean-II days (after haze, 9–35 µg m−3). As indicated in Table S1 and Fig. 1, the PM2.5 concentrations increased from 18 ± 3.3 µg m−3 on clean-I days to 82 ± 26 and 84 ± 22 µg m−3 on haze-I and haze-II days, respectively, and then decreased to 21 ± 10 µg m−3 on clean-II days. This finding obviously indicated that the average PM2.5 concentrations during the examined haze episode are higher than the second-grade national ambient air quality standard in China (75 µg m−3, 24 h), whereas those during clean days are lower than the first-grade national ambient air quality standard in China (35 µg m−3, 24 h). However, the average PM2.5 concentrations during the haze event are lower than those in the cities in winter haze, including Shenyang (108 µg m−3) (Zhang et al., 2020) and Nanjing (123 ± 28.5 µg m−3) (Li et al., 2020), Beijing (158 µg m−3), and Xi'an (345 µg m−3) (Zhang et al., 2018).
As shown in Table S1, the average concentrations of OC and EC were 2.2–15 and 0.36–2.7 µg C m−3 in the four stages, respectively, implying that the distinct changes in OC and EC were higher during haze episodes than on clear days. During the entire study period, WSOC concentration ranged from 0.5 to 12.5 µg C m−3 (4.3 ± 1.2 µg C m−3), which contributed to 53 %–57 % of OC in PM2.5. The HULIS concentration noted in the present study ranged from 0.15 to 6.1 µg C m−3 (2.2 ± 1.9 µg C m−3), which was comparable to those concentrations observed in the PRD region, such as Hong Kong (2.38 ± 1.62 µg C m−3) (Ma et al., 2019), Guangzhou (2.4 ± 1.6 µg C m−3) (Fan et al., 2016), and Heshan (2.08 ± 1.16 µg C m−3) (Jiang et al., 2020), but lower than those in northern cities of China, such as Xi'an (12.4 ± 6.5 µg C m−3) (Huang et al., 2020), Beijing (3.79 ± 3.03 µg C m−3) (Mo et al., 2018), and Lanzhou (4.7 µg C m−3) (Tan et al., 2016). As shown in Fig. 1, HULIS also exhibited obvious variations during the entire sampling period. The average HULIS concentration was 0.46 ± 0.22 µg C m−3 on clean-I days, which sharply increased to 4.5 ± 1.2 µg C m−3 on haze-I days, then decreased to 3.1 ± 1.2 µg C m−3 on haze-II days, and rapidly declined to 0.75 ± 0.52 µg C m−3 on clean-II days. This result was consistent with the changing trend of WSOC, OC, and EC. In addition, the HULIS WSOC ratios were about 0.50 ± 0.13 in the PM2.5 samples, which are in broad agreement with other studies showing that HULIS comprise the major fraction of WSOC (Fan et al., 2016; Ma et al., 2019; Jiang et al., 2020).
As illustrated in Fig. 1, obvious variations in chemical compositions were also observed in these PM2.5 samples. Secondary inorganic aerosols (SIA) (i.e., , , and ), OC, and EC exhibited a similar variation during the entire study period, and their contents sharply increased from 10 January on clean-I days to 13–18 January on haze-I days, then slowly decreased on haze-II days, and finally reached lower levels on clean-II days. It must be noted that the increasing rate of EC was similar to that of SIA on haze-I days, indicating that direct emissions and atmospheric reactions may play similar roles in PM2.5 increase during this haze bloom period. As indicated in Fig. 1f, the highest values of were observed on haze-I days, implying the important influence of traffic exhaust emissions in the haze bloom period (Mo et al., 2018). In addition, the high NO2 and O3 concentrations and the stable meteorological conditions with high temperature also led to the outburst of fine particulate pollution in this period. During haze-II days, the SIA and organic matter (OM) contents in PM2.5 slowly decreased, whereas the concentrations of Na+, Cl−, and unidentified materials in PM2.5 increased (Fig. 1e and h), suggesting that local contribution weakened and regional contribution via sea salt became more important (Jiang et al., 2021). This phenomenon was also observed to be consistent with the changes in the pollutant sources transported by air masses. As indicated in Fig. S1, the PM2.5 samples on haze-II days included some contributors transported from the coastal area of eastern Guangdong Province and Fujian Province, and the PM2.5 is likely to be enriched with sea salt materials and mineral dusts.
3.2 Light absorption
The light absorption properties of WSOC and HULIS (Fig. 1d, i, and j and Table S2) exhibited obvious temporal variations during the sampling period. The AAE values of WSOC and HULIS ranged from 4.1 to 6.4 and 5.6 to 6.6, respectively. The AAE values for HULIS were obviously higher than those for WSOC in the same sample (Fig. 1i), indicating that light absorption of HULIS is more wavelength-dependent than that of WSOC. Similar results have also been observed in previous studies (Park et al., 2018; Jiang et al., 2020; Cao et al., 2021). These differences could be attributed to the differences in chemical composition of chromophores in WSOC and HULIS. As shown in Table S2, the EE365 values of HULIS (5.3–5.6) are all higher than those of WSOC (4.4–5.1), suggesting that the light-absorbing species in HULIS may have lower aromaticity and/or lower molecular weight than those in WSOC (Chen et al., 2016; Li and Hur, 2017). Therefore, the HULIS fractions exhibit relatively high absorption at UV and short visible wavelengths and relatively low absorption at long visible wavelengths, which results in relatively high AAE values. Moreover, the AAE values of HULIS did not present significant variation during the entire haze process, which could be related to the evolution of HULIS chromophores in different stages (Jiang et al., 2020; Huang et al., 2018; Deng et al., 2022). At first, the enhanced oxidation of aromatic species on haze days could lead to the bleaching or degradation of chromophores (a detailed explanation is provided in Sect. 3.3) and thus a lower wavelength dependence (Forrister et al., 2015; Zhan et al., 2022). In contrast, the outburst of secondary organic aerosols and the photolysis of organic aerosols on haze days both tended toward higher AAE values (Saleh et al., 2013; Dasari et al., 2019). Consequently, the different trends in AAE were counterbalanced during the haze days, which resulted in no significant AAE variations being observed for HULIS in the entire sampling process. This is also consistent with the trends of the EE365 ratios of HULIS in the four stages (Table S2).
Light absorption at 365 nm (Abs365) for WSOC and HULIS was 2.5 ± 2.0 and 1.8 ± 1.6 M m−1, respectively (Table S2). HULIS contributed to about 72 % of light absorption coefficients by WSOC, implying that they enriched the major light-absorbing components in WSOC. As shown in Fig. 1d, the Abs365 values for HULIS presented obvious temporal variations. The Abs365,HULIS value was 0.55 ± 0.06 M m−1 on clean-I days, which first increased to 3.4 ± 1.5 M m−1 on haze-I days, then slowly decreased to 2.6 ± 0.85 M m−1 on haze-II days, and finally rapidly declined to 0.64 ± 0.32 M m−1 on clean-II days. This result was similar to the variations in the mass concentration of HULIS. Furthermore, the Abs365 values for HULIS in Guangzhou were found to be higher than those observed in the southeastern Tibetan Plateau (0.38–1.0 M m−1) (Zhu et al., 2018) but obviously lower than those in Xi'an (7.6–36 M m−1) (Shen et al., 2017) and Beijing (3.7–10.1 M m−1) (Du et al., 2014).
In general, MAE365 values can be used to assess the light absorption capacity of target organic compounds (M. Li et al., 2019). As shown in Fig. 1j and Table S2, the average MAE365 value for WSOC was 1.0 ± 0.21 m2 g−1 C (0.68–1.3 m2 g−1 C), nearly the same as 1.1 ± 0.27 m2 g−1 C (0.77–1.8 m2 g−1 C) for HULIS, during the entire sampling period. Moreover, the MAE365 values for HULIS measured in the present study were noted to have dropped to the ranges of those determined in Beijing (1.43 ± 0.33 m2 g−1 C) (Mo et al., 2018), Xi'an (0.91–1.85 m2 g−1 C) (Yuan et al., 2021), and Hong Kong (1.84 ± 0.77 m2 g−1 C) (Ma et al., 2019). The average MAE365 values for HULIS exhibited some temporal variations. The MAE365 values for HULIS were 0.91 ± 0.03 and 0.95 ± 0.11 m2 g−1 C on haze-I and haze-II days, respectively, which were lower than those (1.3 ± 0.22 and 1.3 ± 0.27 m2 g−1 C, respectively) observed on clean-I and clean-II days, suggesting that HULIS have a relatively weaker light absorption capability on haze days. This finding is consistent with the results reported by Zhang et al. (2017), who found that the MAE365 values in the heating or non-heating seasons during hazy days were lower than those on clean days. These differences in MAE365 values may potentially contribute to the enhanced oxidation reaction that was derived by the increased O3 levels and high temperature and relative humidity (RH) during haze days (Fig. 1). This oxidation process would lead the chromophores containing C=C unsaturated bonds to be severely degraded (J. Wang et al., 2017; Zhang et al., 2017). Besides, an increase in additional sources for HULIS in the study area, such as weaker or non-light-absorbing compounds formed by atmospheric oxidation, could also result in weaker light absorption of HULIS during the haze episode (Liu et al., 2018).
3.3 Molecular evolution of HULIS during the haze process
For an in-depth understanding of the variation in HULIS at the molecular level during the haze process, the four HULIS samples collected in different stages of the haze process were analyzed by ESI FT-ICR MS in both negative and positive modes. As shown in Fig. 2, thousands of peaks were detected in the mass range between 100 and 700, with the high-intensity ions noted within 150–400. It is obvious that some organic compounds with stronger arbitrary abundance were labeled, and their formulas, double-bond equivalent (DBE), modified aromaticity index (AImod), and potential sources are listed in Table S3. Compounds a (C7H7NO3) and b (C8H6O4) both have high DBE values, which might be assigned to aromatics such as methylnitrophenol and phthalic acid, whereas compound d (C8H18O4S) with a low DBE value and high ratio was probably aliphatic organosulfate. According to previous studies, these organic molecules might be derived from BB and diesel fuel, and thereby these results suggest that both BB and vehicular emissions are important sources of BrC in ambient aerosols (Mohr et al., 2013; Riva et al., 2015; Blair et al., 2017). Furthermore, compound e (C10H17NO7S) and compound f (C10H18N2O11S) in Table S3 were found to be identical to the oxidation products of monoterpenes, suggesting that biogenic sources could contribute to the formation of HULIS (Surratt et al., 2008; Wang et al., 2019). Thus, HULIS could be affected by multiple sources during the haze process, possibly including BB, biogenic sources, and anthropogenic emissions.
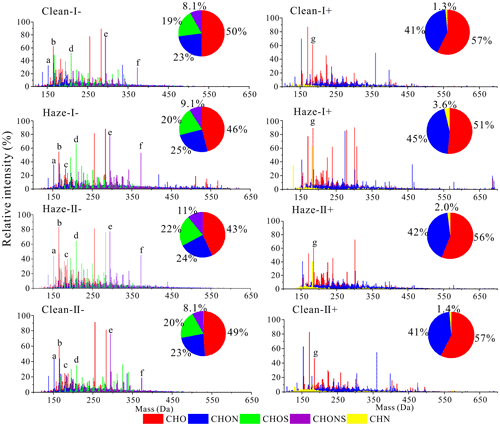
Figure 2Mass spectra of HULIS detected in ESI− and ESI+ modes during the haze process. The pie charts represent the intensity percent of different compound groups.
The identified formulas could be divided into seven compound categories, namely, CHO−, CHON−, CHOS−, and CHONS− detected in ESI− mode and CHO+, CHN+, and CHON+ detected in ESI+ mode. As illustrated in Fig. 2, the CHO compounds were the most abundant group in all the HULIS, accounting for 43 %–50 % and 51 %–57 % of the overall compounds detected in the ESI− and ESI+ modes, respectively. It must be noted that relatively low contents of CHO− were detected during the haze episode (haze-I and haze-II days) and of CHO+ molecules in haze-I HULIS. The CHON compounds were the second most abundant group in all the HULIS. As shown in Fig. 2, the relative content of CHON− was 23 % on clean-I days, which slightly increased to 24 %–25 % in the haze episode and then decreased to 23 % on clean-II days. In contrast, the relative content of CHON+ compounds was 41 % on clean-I days, which increased to 45 % on haze-I days and then fell to 42 % on haze-II days and 41 % on clean-II days. Both CHOS− and CHONS− compounds were identified in all the four HULIS, accounting for 19 %–22 % and 8 %–11 % of the total identified compounds, respectively. The CHN+ compounds were the least abundant (1.3 %–3.6 %) in the four HULIS samples and were relatively high during the haze episode, especially on haze-I days.
Tables S4 and S5 show the relative-abundance-weighted elemental ratios, molecular weight (MW), DBE, AImod, and carbon oxidation state (OSC) for the identified compounds in HULIS. The MWw values for HULIS determined in the ESI− mode on haze-I and haze-II days were 302 and 283, respectively, which were higher than those on clean-I and clean-II days (266 and 264, respectively). Similar variation was also observed for MWw for HULIS detected in ESI+ mode (Table S5). These results clearly indicated that more higher-MW compounds constituted HULIS obtained during the haze episode. Furthermore, the molecular properties of HULIS in different stages of the haze process also exhibited some observable differences. As shown in Table S4, the HULIS samples in the haze episode detected by the ESI− mode presented relatively low AImod,w values and relatively high , , and ratios compared to those on clean days, indicating that haze HULIS exhibited relatively low aromaticity and a higher oxidation degree than clean HULIS. These differences can be attributed to the enhanced oxidation degradation of aromatic compounds (e.g., phenols, nitroaromatic compounds, and polycyclic aromatic hydrocarbons (PAHs)) during the haze process. In addition, an increased contribution from traffic emissions and secondary reactions of biogenic VOCs (bio-VOCs) also decreased the aromaticity and increased the oxidation degree of HULIS (Liu et al., 2016; Tang et al., 2020). These changes in HULIS compounds led to the decrease in their MAE365 values during the haze episode, as described above (Zhong and Jang, 2014; Song et al., 2019).
3.3.1 CHO compounds
The CHO compounds bear O-containing functional groups and have been frequently detected in ambient aerosols. As shown in Fig. 2, the CHO compounds were the predominant component in the four HULIS samples, and the MWw values for CHO− and CHO+ compounds were 247–288 and 236–272, respectively, with relatively high MWw values observed for the CHO group (CHO− and CHO+) in haze HULIS, especially in haze-I samples. This finding may be related to the stronger oxidation of HULIS during haze days because the aqueous oxidation of biomass burning aerosols was found to yield high MW of organic products (Tomaz et al., 2018; Yu et al., 2016).
The OSC is often used to describe the degree of oxidation of organic species in the atmosphere (Kroll et al., 2011; X. K. Wang et al., 2017). Figure 3 shows plots of OSC versus the carbon number for the CHO compounds. As indicated in the figure, CHO compounds exhibited OSC from −2 to +1 with up to 40 carbon atoms. Kroll et al. (2011) proposed that compounds with OSC between −0.5 and +1 and <18 carbon atoms can be attributed to semi-volatile and low-volatility oxidized organic aerosols (SV-OOA and LV-OOA), which are mainly formed by complex oxidation reactions in atmosphere. Compounds with OSC between −0.5 and −1.5 and 6–23 carbon atoms are related to primary biomass burning organic aerosols (BBOA). In addition, compounds with OSC between −1 and −2 and ≥18 carbon atoms have been suggested to be hydrocarbon-like organic aerosols (HOA), which are regarded as a primary combustion surrogate (Zhang et al., 2005; Kroll et al., 2011; X. K. Wang et al., 2017).
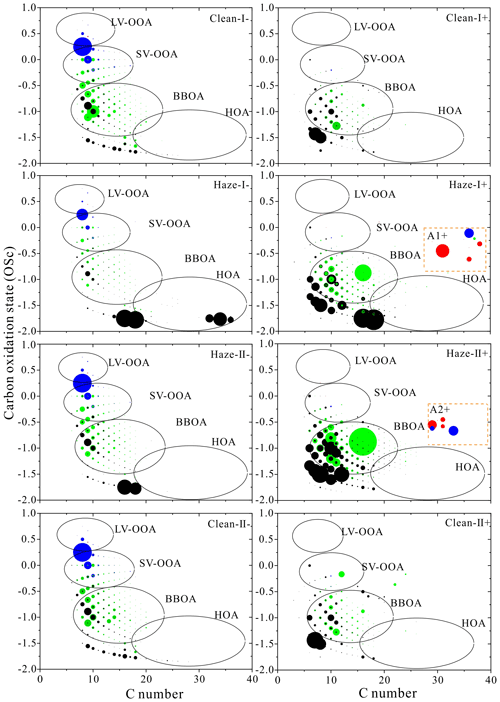
Figure 3Carbon oxidation state (OSC) plots for CHO− and CHO+. Formulas with black, green, blue, and red are assigned to aliphatic (AI=0), olefinic (), aromatic (), and condensed aromatic (AI≥0.67) species (Koch and Dittmar, 2006), respectively.
As illustrated in Fig. 3 and Table S6, most of the CHO− compounds clustered in the BBOA region, accounting for 40 %–46 % of the total CHO− compounds, thus suggesting that BB may be a major contributor to CHO compounds in HULIS. Figure 3 clearly indicates that the majority of aromatic and condensed aromatic compounds produced signals in the OSC region between −0.5 and 1.0 and with a carbon number of 3–18 (Fig. 3), which corresponded to SV-OOA and LV-OOA. The proportions of SV-OOA and LV-OOA accounted for 23 %–28 % and 1.9 %–2.4 % of the total CHO− compounds, respectively, and presented no significant variation. In contrast, the HOA components on haze-I days showed the highest abundance (18 %), which were much higher than those (3.5 %–4.5 %) on haze-II, clean-I, and clean-II days. This finding indicated that the increase in the primary source is associated with fossil fuel combustion such as vehicle emissions during the haze bloom period (Zhang et al., 2005).
As shown in Fig. 3, CHO+ compounds presented lower OSC (from −2.0 to 1.0) than CHO− compounds. Most of the CHO+ compounds occurred in the BBOA region in all four HULIS samples, forming up to 60 %–72 % of the total CHO+ compounds, which again suggests that BB is an important contributor to CHO compounds in HULIS. The HOA among CHO+ compounds showed the same changing trends as those among CHO− compounds, and higher HOA abundance was observed during haze-I days. In addition, some high AImod values of aromatics were found in the regions A1+ and A2+ (Fig. 3), which implied that the highest AImod values (AI≥0.67) with DBE≥22 were only detected during the haze days, possibly owing to soot-derived materials or oxidized PAHs (Decesari et al., 2002; Kuang and Shang, 2020). It must be noted that the sampling site in the present study is influenced by traffic sources; the enhanced oxidation of vehicle-exhausted soot also results in the accumulation of water-soluble high aromatic organic species (Decesari et al., 2002).
3.3.2 CHON compounds
In the present study, 1379–2217 and 2008–2943 formulas were assigned to CHON compounds identified in the ESI− and ESI+ spectra, respectively, which accounted for 23 %–25 % (ESI−) and 41 %–45 % (ESI+) of the total identified compounds, respectively. Relatively high contents of CHON− compounds were obviously detected in HULIS samples obtained during haze-I days, suggesting the occurrence of more N-containing components in HULIS during haze bloom days. As shown in Tables S4 and S5, the average MWw values for CHON− and CHON+ compounds were 328 and 317 on haze-I days, respectively, which were slightly higher than those determined on haze-II days, and all were higher than those observed on clean-I and clean-II days. Meanwhile, the AImod,w values for CHON− on haze days were 0.31–0.34, which were slightly lower than those on clean days (0.37 and 0.40). These findings indicated that more high-MW CHON compounds with lower aromatic structures were formed during the haze episode.
The ratios for CHON− and CHON+ during haze-I and haze-II days were 5.3–5.7 and 3.8, respectively, which were higher than those ratios determined during the two clean periods, confirming that these compounds were highly oxidized during the haze episode (Tables S4 and S5). In general, compounds with may indicate oxidized N groups such as nitro (−NO2) or nitrooxy (−ONO2), whereas compounds with may denote the reduced N compounds (i.e., amines) (Lin et al., 2012; Song et al., 2018). In the present study, most of the CHON compounds (79 %–91 % of CHON− compounds and 61 %–64 % of CHON+ compounds) exhibited , suggesting that high concentrations of nitro compounds or organonitrates were contained in the CHON compounds. Moreover, these compounds were more abundant in the CHON− group during the haze episode (87 %–91 %), when compared with those during clean-I and clean-II days (79 %–82 %), again implying that CHON− compounds undergo relatively high oxidization during the haze episode. As indicated in Fig. 1, the increase in NO2 was consistent with increased production of highly oxidized N-containing organic compounds (NOCs) during the haze episode, which suggested the significant contribution of NO3-related multigenerational chemistry to organonitrate aerosol formation (Berkemeier et al., 2016).
The majority of aromatics and condensed aromatics produced clear signals in regions associated with SV-OOA and LV-OOA (Fig. 4). BBOA also constituted a significant proportion (33 %–39 %) in the CHON− group, and a relatively low BBOA content was observed on haze-I days. The abundance of HOA was relatively low, accounting for 2.3 %–7.8 % of the total CHON compounds, and the relative abundance of HOA on haze-I days was much higher than that on haze-II, clean-I, and clean-II days, suggesting the accumulation of primary fossil fuel combustion during haze-I days.
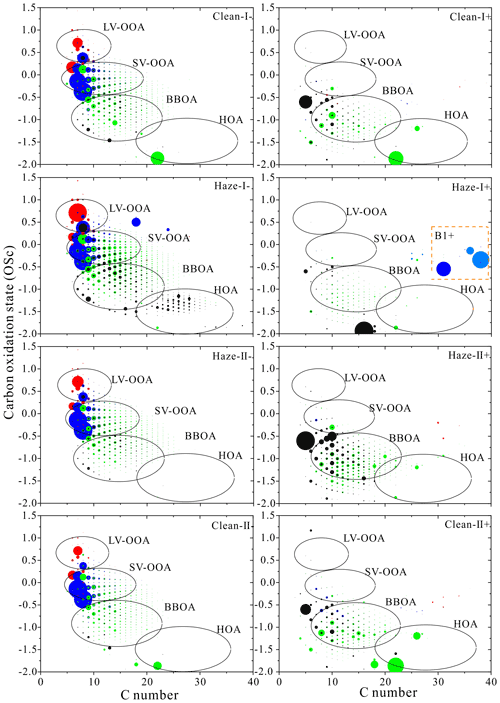
Figure 4Carbon oxidation state (OSC) plots for CHON− and CHON+. Formulas with black, green, blue, and red are assigned to aliphatic (AI=0), olefinic (), aromatic (), and condensed aromatic (AI≥0.67) species (Koch and Dittmar, 2006), respectively.
The CHON+ compounds mainly occurred at the range of , with average OSC values of around −1.0 for each sample, clearly indicating that CHON+ compounds were lower than CHON− compounds. Most of the CHON+ compounds were detected in the BBOA region, accounting for 60 %–76 % of the total CHON+ compounds. The relative contribution of BBOA on haze-I days was lower than that on haze-II and clean days. Moreover, a large number of aromatic species were observed at the region B1+ (Fig. 4), demonstrating that higher aromatic compounds were only detected on haze-I days, which may be related to soot or black carbon (BC). A similar trend was also exhibited by CHO+ compounds, indicating the contribution of local combustion sources (e.g., traffic emission) during haze-I days.
3.3.3 CHOS and CHONS compounds
In this study, 478–696 CHOS compounds and 306–589 CHONS compounds were identified in ESI− mode (Table S4). Among these S-containing compounds, >86 % of the CHOS compounds had ratios >4, whereas >89 % of the CHONS compounds presented ratios >7, suggesting that these S-containing compounds were possibly organosulfates and nitrooxyorganosulfates. As listed in Table S4, the AImod,w values for CHOS and CHONS were about 0.02 and 0.01 in the HULIS fraction, which were much lower than those for CHO and CHON. Almost 99 % of the CHOS and CHONS compounds in the HULIS fraction had AImod values <0.5, while >93 % of the CHONS compounds had AImod=0, indicating that they were mainly comprised of aliphatic and olefinic organosulfates. These results are consistent with the previous findings that the major S-containing compounds among organic aerosols in Guangzhou are organosulfates formed by secondary oxidation reaction of long-chain alkenes/fatty acids with SO2 (Jiang et al., 2020), which generally possessed long aliphatic carbon chains and a higher degree of oxidation. However, these compounds are different from the S-containing compounds detected during the hazy days in Beijing (Jiang et al., 2016; Mo et al., 2018), which were determined to be aliphatic organosulfates with a low degree of oxidation and higher amounts of aromatics and PAH-derived organosulfates, having a strong correlation with anthropogenic emissions.
As described earlier, CHOS− and CHONS− compounds might be related to organosulfates or nitrooxyorganosulfates, which have been observed to be derived from atmospheric reactions of bio-VOCs such as α-pinene, limonene, and isoprene (Huang et al., 2018; Surratt et al., 2008) and fossil fuel combustion including coal combustion and off-road engine emissions (Song et al., 2018, 2019; Cui et al., 2019). In the present study, the relative contents of S-containing compounds (CHOS+CHONS) in the HULIS fraction on haze days were all higher than those on clean days (Fig. 2). Moreover, the CHOS and CHONS compounds in haze HULIS always have relatively high ratios compared to those in clean HULIS. These findings suggested the relatively high contribution of SO2-related chemical oxidation during the haze event.
3.3.4 CHN compounds
The N bases (CHN) are usually identified in ambient aerosols and smoke from BB. In the present study, 110–165 CHN+ compounds were identified in ESI+ mode, with most of them (>86 %) presenting DBE≥2, suggesting that they might be nitrile and amine species (Lin et al., 2012). As shown in Fig. 2, the abundances of CHN+ compounds were 2.0 %–3.6 % on the haze days, which were much higher than those noted on clean days (1.3 %–1.4 %), indicating a higher contribution of CHN+ compounds to the HULIS fraction during the haze episode. The MWw values for CHN+ compounds were 204–223, which were lower than those for the other groups (i.e., CHO+, CHON+) (Table S5). However, the average AImod values for N bases (0.37–0.48) detected in the ESI+ mode were much higher than those for CHO+ (0.11–0.12) and CHON+ (0.20–0.22) compounds, implying that these reduced CHN+ compounds exhibited more unsaturated or aromatic structures.
To further understand the molecular distribution of CHN+ compounds during the haze process, van Krevelen (VK) diagrams were constructed by plotting the ratio versus the ratio (Fig. S2). It was obvious that this plot could separate the compound classes with different degrees of AI. As shown in Fig. S2, compounds (denoted in black color) in the upper region of the VK diagram had one N atom with DBE=0, indicating that they are aliphatic amines. It can be noted from Table S7 that the aliphatic group presented the lowest abundance in all the samples, suggesting that the CHN+ compounds possessed comparatively low aliphatic structures. Olefinic compounds showed the highest abundance in the four samples, which accounted for 37 %–51 % of the total CHN+ compounds. Importantly, a large proportion of the compounds (>39 %) exhibited high degree of AI (AI>0.5) (Fig. S2 and Table S7), suggesting a large extent of aromatic structure and N-heterocyclic rings in HULIS. Moreover, the CHN+ compounds on haze-I days presented obviously lower content of aromatic structures than those on haze-II, clean-I, and clean-II days, signifying the relatively high contribution of fossil fuel combustion (which generally emits more low aromatic CHN compounds) during the haze bloom episode (Song et al., 2022). In addition, the CHN+ group also constituted a large proportion of BBOA (Table S6), which indicated the significant contribution of BB. However, it must be noted that a relatively low content of BBOA was detected during haze-I days, which was consistent with the changing trends of CHON− or CHON+ compounds during the haze episode. These results suggested the relatively low contribution of BB during haze-I days because quiet and stable weather conditions can prevent regional transport of BB sources during this stage (Wu et al., 2018).
3.4 Factors influencing light absorption and molecular characteristics of HULIS during the haze bloom-decay process
As described earlier, the light absorption properties of HULIS exhibited obvious variation during the haze bloom-decay process. The average Abs365 value for HULIS was 0.55 ± 0.06 M m−1 on clean-I days, which first increased to 3.4 ± 1.5 M m−1 on haze-I days, then slowly decreased to 2.6 ± 0.85 M m−1 on haze-II days, and finally rapidly declined to 0.64 ± 0.32 M m−1 on clean-II days. In general, the light absorption of HULIS can be related to their chemical and molecular properties that are influenced by factors such as sources, secondary formation, and the aging process. The results of principal component analysis (PCA) obviously showed a positive loading for principal component 1 (PC1), and the Abs365 values for HULIS were clustered with EC, , Lev, , and (Fig. 5). These results suggested that BB and other sources such as new particle formation could contribute to light absorption of HULIS (An et al., 2019; Song et al., 2019). Similarly, the findings of Pearson correlation coefficient analysis revealed that the Abs365 values for HULIS exhibited significant positive correlations with (r=0.728, p<0.01) and Lev (r=0.800, p<0.01) (Table S8). As Lev and are generally considered tracers derived from BB, these results suggested the significant contribution of BB to light absorption of HULIS. This observation was also supported by the abundance of BBOA compounds detected in all the four HULIS samples (Table S6). The significant positive relationships between the Abs365 values for HULIS and secondary ions (i.e., (r=0.702, p<0.01), (r=0.554, p<0.05), and (r=0.899, p<0.01)) indicated the important impact of secondary formation on the light absorption of HULIS. Besides, the Abs365 values for HULIS were also strongly correlated with NO2, O3, and NO2, which confirmed the important impact of atmospheric oxidation reactions on the light absorption of HULIS.
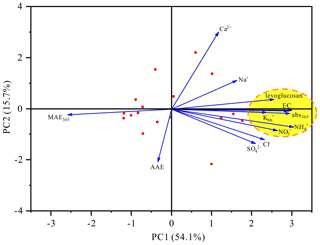
Figure 5Principal component analysis results for the optical properties of HULIS and chemical compositions of PM2.5.
Table 1Formula number of potential BrC chromophores and the intensity ratios of each group of potential BrC in total potential BrC and each group of total identified formulas, respectively.
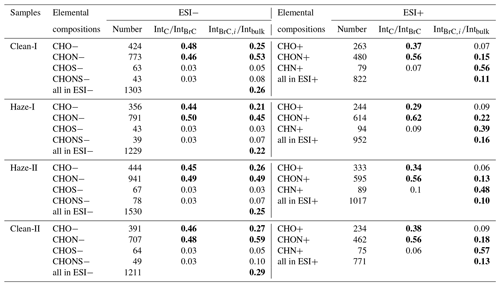
IntC: the intensity of each group of identified potential BrC; IntBrC: the sum intensity of identified potential BrC; Intbulk: the sum intensity of each group of total identified formulas. The bold formatting represents the relative high values in each group.
It must be noted that MAE365 is a key parameter signifying the light absorption ability of HULIS. As listed in Table S2, the MAE365 values for HULIS varied in different stages and were lower on haze days owing to the variation in the chemical and molecular composition of HULIS during the haze bloom-decay process. Furthermore, the AImod values for HULIS varied in different stages (Tables S4) and were relatively low on haze days, indicating that haze HULIS have a comparatively low degree of conjugation or aromaticity. This finding suggested that the HULIS compounds may undergo higher oxidation during the haze episode, causing a decline in chromophores and reduction in the light absorption capacity of HULIS (Lin et al., 2017). Besides, the accumulated contribution of organic compounds from vehicle emission and secondary chemical reactions of bio-VOCs may also dilute light-absorbing compounds in haze HULIS (Tang et al., 2020; Liu et al., 2016).
Lin et al. (2018) reported that potential light-absorbing chromophores can be determined in the region between (linear conjugated polyenes CxHyC2) and (fullerene-like hydrocarbons). In the present study, most of the high-intensity CHON, CHO, and CHN compounds with high AI values were clustered in a potential BrC chromophore region (Figs. S3 and S4), which mainly comprised CHON (46 %–50 % in ESI− mode and 56 %–62 % in ESI+ mode) and CHO (44 %–48 % in ESI− mode and 29 %–38 % in ESI+ mode) compounds (Table 1). Although the contribution of CHN+ compounds to BrC was relatively low, the content of potential chromophores among the total CHN+ compounds was higher than those in CHON+ and CHO+ compounds. Therefore, these three groups of light-absorbing compounds (i.e., CHON+, CHN+, and CHO+ compounds) were further examined. As shown in Table 1, the IntIntBrC values of CHO− (i.e., content of CHO− chromophores in the total chromophores) decreased from 48 % to 44 %, whereas the IntIntBrC values of CHON− increased from 46 % to 50 % during the haze bloom process. These findings indicated that more NOC chromophores were formed during this stage in which higher NO2 concentration may be preferred for the formation of N-containing chromophores such as nitrophenols. However, it must be noted that the proportions of both CHO− and CHON− chromophores among the total identified compounds decreased from clean-I to haze-I days, suggesting the occurrence of a stronger photo-bleaching process during the haze bloom stage (Zeng et al., 2020). Likewise, both CHO+ and CHON+ compounds presented similar variation during the entire study period. In addition, the CHN+ compounds also exhibited higher IntIntBrC values during the haze bloom process, suggesting the accumulated contribution from the local combustion process. Furthermore, the proportion of CHON+ chromophores in the total CHON+ compounds increased with the decreasing content of CHN+ chromophores, possibly implying that some aromatic CHN compounds were transformed to CHON+ compounds during the aging process.
This study investigated the evolution of light absorption and molecular properties of HULIS during a winter haze bloom-decay process and examined the key factors affecting the light absorption of HULIS in Guangzhou, China. The results showed that HULIS exhibited significant variation in light absorption during the haze bloom-decay process. First, higher Abs365 values were observed on haze days, indicating the presence of significant quantities of light-absorbing organic compounds during the haze episode. However, the MAE365 values for HULIS on haze days were lower than those on clean days, suggesting the light absorption capabilities of HULIS were weakened during the haze event. Furthermore, CHON and CHO compounds, exhibiting a relatively high degree of conjugated structure, were the most abundant groups in all the HULIS samples and were also the major contributors to the light absorption capacity of HULIS. Importantly, the molecular properties of HULIS dynamically varied during the entire haze episode. When compared with HULIS on clean days, those on haze days presented relatively low AImod values and higher , , and ratios, suggesting the predominance of compounds with low aromaticity and higher oxidation in HULIS during haze episodes. These results indicated that HULIS compounds undergo relatively strong oxidation during the haze days. Moreover, PCA and Pearson correlation analysis revealed that BB and secondary chemical formation both contributed to the variation in the light absorption properties of HULIS. Both primary sources (such as the accumulated contribution of organic compounds formed from local traffic emissions) and secondary sources (such as stronger chemical reactions) led to the rapid increase in HULIS during the haze bloom days. However, stronger oxidation of HULIS compounds were observed during the haze episode, and some potential BrC chromophores were degraded. In addition, the chemical reactions of bio-VOCs such as isoprene also diluted the light-absorbing compounds in HULIS.
Thus, the present study provides novel insights into the light and molecular evolution of HULIS during haze events, which are important for predicting the environmental and climatic effects of HULIS. However, as this study examined only one haze bloom-decay process in winter in Guangzhou, the results obtained may be not adequate for understanding all the haze episodes in southern China. Therefore, there is a need for a comprehensive investigation of haze episodes in different seasons and regions in the future.
The research data are available in the Harvard Dataverse (https://doi.org/10.7910/DVN/DYGYQT, Song, 2022).
The supplement related to this article is available online at: https://doi.org/10.5194/acp-23-963-2023-supplement.
JS and PP designed the research together. CZ, TC, and ML carried out the PM2.5 sampling experiments. CZ and TC extracted and analyzed the WSOC and HULIS samples. BJ analyzed the HULIS samples by FT-ICR MS. CZ and JS wrote the paper. JL, XD, ZY, and GZ commented on and revised the paper.
The contact author has declared that none of the authors has any competing interests.
Publisher's note: Copernicus Publications remains neutral with regard to jurisdictional claims in published maps and institutional affiliations.
This is contribution no. IS-3298 from GIGCAS.
This research has been supported by the National Natural Science Foundation of China (grant nos. 42192514 and 41977188) and Guangdong Foundation for Program of Science and Technology Research (grant nos. 2020B1212060053 and 2019B121205006).
This paper was edited by Roya Bahreini and reviewed by two anonymous referees.
An, Z., Huang, R. J., Zhang, R., Tie, X., Li, G., Cao, J., Zhou, W., Shi, Z., Han, Y., Gu, Z., and Ji, Y.: Severe haze in northern China: A synergy of anthropogenic emissions and atmospheric processes, P. Natl. Acad. Sci. USA, 116, 8657–8666, https://doi.org/10.1073/pnas.1900125116, 2019.
Berkemeier, T., Ammann, M., Mentel, T. F., Poschl, U., and Shiraiwa, M.: Organic nitrate contribution to new particle formation and growth in secondary organic aerosols from alpha-pinene ozonolysis, Environ. Sci. Technol., 50, 6334–6342, https://doi.org/10.1021/acs.est.6b00961, 2016.
Bianco, A., Deguillaume, L., Vaitilingom, M., Nicol, E., Baray, J. L., Chaumerliac, N., and Bridoux, M.: Molecular characterization of cloud water samples collected at the Puy de Dome (France) by Fourier transform ion cyclotron resonance mass spectrometry, Environ. Sci. Technol., 52, 10275–10285, https://doi.org/10.1021/acs.est.8b01964, 2018.
Blair, S. L., MacMillan, A. C., Drozd, G. T., Goldstein, A. H., Chu, R. K., Paša-Tolić, L., Shaw J. B., Tolić, N., Lin, P., Laskin, J., Laskin, A., and Nizkorodov, S. A.: Molecular characterization of organosulfur compounds in biodiesel and diesel fuel secondary organic aerosol. American Chemical Society, Environ. Sci. Technol., 51, 119–127, https://doi.org/10.1021/acs.est.6b03304, 2017.
Cao, T., Li, M., Zou, C., Fan, X., Song, J., Jia, W., Yu, C., Yu, Z., and Peng, P.: Chemical composition, optical properties, and oxidative potential of water- and methanol-soluble organic compounds emitted from the combustion of biomass materials and coal, Atmos. Chem. Phys., 21, 13187–13205, https://doi.org/10.5194/acp-21-13187-2021, 2021.
Chen, Q., Ikemori, F., and Mochida, M.: Light absorption and excitation-emission fluorescence of urban organic aerosol components and their relationship to chemical structure, Environ. Sci. Technol., 50, 10859–10868, https://doi.org/10.1021/acs.est.6b02541, 2016.
Cui, M., Li, C., Chen, Y., Zhang, F., Li, J., Jiang, B., Mo, Y., Li, J., Yan, C., Zheng, M., Xie, Z., Zhang, G., and Zheng, J.: Molecular characterization of polar organic aerosol constituents in off-road engine emissions using Fourier transform ion cyclotron resonance mass spectrometry (FT-ICR MS): implications for source apportionment, Atmos. Chem. Phys., 19, 13945–13956, https://doi.org/10.5194/acp-19-13945-2019, 2019.
Dasari, S., Andersson, A., Bikkina, S., Holmstrand, H., Budhavant, K., Satheesh, S., Asmi, E., Kesti, J., Backman, J., Salam, A., Bisht, D. S., Tiwari, S., Hameed, Z., and Gustafsson, Ö: Photochemical degradation affects the light absorption of water-soluble brown carbon in the South Asian outflow, Sci. Adv., 5, eaau8066, https://doi.org/10.1126/sciadv.aau8066, 2019.
Decesari, S., Facchini, M. C., Matta, E., Mircea, M., Fuzzi, S., Chughtai, A. R., and Smith, D. M.: Water soluble organic compounds formed by oxidation of soot, Atmos. Environ., 36, 1827–1832, https://doi.org/10.1016/s1352-2310(02)00141-3, 2002.
Deng, J., Ma, H., Wang, X., Zhong, S., Zhang, Z., Zhu, J., Fan, Y., Hu, W., Wu, L., Li, X., Ren, L., Pavuluri, C. M., Pan, X., Sun, Y., Wang, Z., Kawamura, K., and Fu, P.: Measurement report: Optical properties and sources of water-soluble brown carbon in Tianjin, North China – insights from organic molecular compositions, Atmos. Chem. Phys., 22, 6449–6470, https://doi.org/10.5194/acp-22-6449-2022, 2022.
Du, Z., He, K., Cheng, Y., Duan, F., Ma, Y., Liu, J., Zhang, X., Zheng, M., and Weber, R.: A yearlong study of water-soluble organic carbon in Beijing II: Light absorption properties, Atmos. Environ., 89, 235–241, https://doi.org/10.1016/j.atmosenv.2014.02.022, 2014.
Fan, X., Song, J., and Peng, P.: Comparative study for separation of atmospheric humiclike substance (HULIS) by ENVI-18, HLB, XAD-8 and DEAE sorbents: elemental composition, FT-IR, 1H-NMR and off-line thermochemolysis with tetramethylammonium hydroxide (TMAH), Chemosphere, 93, 1710–1719, https://doi.org/10.1016/j.chemosphere.2013.05.045, 2013.
Fan, X., Song, J., and Peng, P.: Temporal variations of the abundance and optical properties of water soluble Humic-Like Substances (HULIS) in PM2.5 at Guangzhou, China, Atmos. Res., 172–173, 8–15, https://doi.org/10.1016/j.atmosres.2015.12.024, 2016.
Fan, X., Cao, T., Yu, X., Wang, Y., Xiao, X., Li, F., Xie, Y., Ji, W., Song, J., and Peng, P.: The evolutionary behavior of chromophoric brown carbon during ozone aging of fine particles from biomass burning, Atmos. Chem. Phys., 20, 4593–4605, https://doi.org/10.5194/acp-20-4593-2020, 2020.
Fan, X. J., Song, J. Z., and Peng, P. A.: Comparison of isolation and quantification methods to measure humic-like substances (HULIS) in atmospheric particles, Atmos. Environ., 60, 366–374, https://doi.org/10.1016/j.atmosenv.2012.06.063, 2012.
Forrister, H., Liu, J., Scheuer, E., Dibb, J., Ziemba, L., Thornhill, K. L., Anderson, B., Diskin, G., Perring, A. E., Schwarz, P., Campuzano-Jost, P., Day, D. A., Palm, B. B., Jimenez, J. L., Nenes, A., and Weber, R. J.: Evolution of brown carbon in wildfire plumes, Geophys. Res. Lett., 42, 4623–4630, https://doi.org/10.1002/2015gl063897, 2015.
Graber, E. R. and Rudich, Y.: Atmospheric HULIS: How humic-like are they? A comprehensive and critical review, Atmos. Chem. Phys., 6, 729–753, https://doi.org/10.5194/acp-6-729-2006, 2006.
Huang, R. J., Yang, L., Cao, J., Chen, Y., Chen, Q., Chen, Q., Li, Y. J., Duan, J., Zhu, C. C., Dai, W. T., Wang, K., Lin, C. S., Ni, H. Y., Corbin, J. C., Wu, Y. F., Zhang, R. J., Tie, X. X., Hoffmann, T., O'Dowd, C., and Dusek, U.: Brown carbon aerosol in urban Xi'an, Northwest China: The composition and light absorption properties, Environ. Sci. Technol., 52, 6825–6833, https://doi.org/10.1021/acs.est.8b02386, 2018.
Huang, R. J., Yang, L., Shen, J. C., Yuan, W., Gong, Y. Q., Guo, J., Cao, W., Duan, J., Ni, H., Zhu, C., Dai, W., Li, Y., Chen, Y., Chen, Q., Wu, Y., Zhang, R., Dusek, U., O'Dowd, C., and Hoffmann, T.: Water-insoluble organics dominate brown carbon in wintertime urban aerosol of China: chemical characteristics and optical properties, Environ. Sci. Technol., 54, 7836–7847, https://doi.org/10.1021/acs.est.0c01149, 2020.
Jiang, B., Kuang, B. Y., Liang, Y., Zhang, J., Huang, X. H. H., Xu, C., Yu, J. Z., and Shi, Q.: Molecular composition of urban organic aerosols on clear and hazy days in Beijing: a comparative study using FT-ICR MS, Environ. Chem., 13, 888–901, https://doi.org/10.1071/en15230, 2016.
Jiang, H., Li, J., Chen, D., Tang, J., Cheng, Z., Mo, Y., Su, T., Tian, C., Jiang, B., Liao, Y., and Zhang, G.: Biomass burning organic aerosols significantly influence the light absorption properties of polarity-dependent organic compounds in the Pearl River Delta Region, China, Environ. Int., 144, 106079, https://doi.org/10.1016/j.envint.2020.106079, 2020.
Jiang, H., Li, J., Sun, R., Liu, G., Tian, C., Tang, J., Cheng, Z., Zhu, S., Zhong, G., Ding, X., and Zhang, G.: Determining the sources and transport of brown carbon using radionuclide tracers and modeling, J. Geophys. Res.-Atmos., 126, e2021JD034616, https://doi.org/10.1029/2021JD034616, 2021.
Kampf, C. J., Filippi, A., Zuth, C., Hoffmann, T., and Opatz, T.: Secondary brown carbon formation via the dicarbonyl imine pathway: nitrogen heterocycle formation and synergistic effects, Phys. Chem. Chem. Phys., 18, 18353–18364, https://doi.org/10.1039/c6cp03029g, 2016.
Koch, B. P. and Dittmar, T.: From mass to structure: an aromaticity index for high-resolution mass data of natural organic matter, Rapid Commun. Mass Sp., 20, 926–932, https://doi.org/10.1002/rcm.2386, 2006.
Kroll, J. H., Donahue, N. M., Jimenez, J. L., Kessler, S. H., Canagaratna, M. R., Wilson, K. R., Altieri, K. E., Mazzoleni, L. R., Wozniak, A. S., Bluhm, H., Mysak, E. R., Smith, J. D., Kolb, C. E., and Worsnop, D. R.: Carbon oxidation state as a metric for describing the chemistry of atmospheric organic aerosol, Nat. Chem., 3, 133–139, https://doi.org/10.1038/nchem.948, 2011.
Kuang, K. and Shang, J.: Changes in light absorption by brown carbon in soot particles due to heterogeneous ozone aging in a smog chamber, Environ. Pollut., 266, 115273, https://doi.org/10.1016/j.envpol.2020.115273, 2020.
Laskin, A., Laskin, J., and Nizkorodov, S. A.: Chemistry of atmospheric brown carbon, Chem. Rev., 115, 4335–4382, https://doi.org/10.1021/cr5006167, 2015.
Li, K., Jacob, D. J., Liao, H., Shen, L., Zhang, Q., and Bates, K. H.: Anthropogenic drivers of 2013–2017 trends in summer surface ozone in China, P. Natl. Acad. Sci. USA, 116, 422–427, https://doi.org/10.1073/pnas.1812168116, 2019.
Li, M., Fan, X., Zhu, M., Zou, C., Song, J., Wei, S., Jia, W., and Peng, P.: Abundance and light absorption properties of brown carbon emitted from residential coal combustion in China, Environ. Sci. Technol., 53, 595–603, https://doi.org/10.1021/acs.est.8b05630, 2019.
Li, P. and Hur, J.: Utilization of UV-Vis spectroscopy and related data analyses for dissolved organic matter (DOM) studies: A review, Crit. Rev. Env. Sci. Tec., 47, 131–154, https://doi.org/10.1080/10643389.2017.1309186, 2017.
Li, S. W., Chang, M., Li, H., Cui, X. Y., and Ma, L. Q.: Chemical compositions and source apportionment of PM2.5 during clear and hazy days: seasonal changes and impacts of Youth Olympic Games, Chemosphere, 256, 127163, https://doi.org/10.1016/j.chemosphere.2020.127163, 2020.
Lin, P., Engling, G., and Yu, J. Z.: Humic-like substances in fresh emissions of rice straw burning and in ambient aerosols in the Pearl River Delta Region, China, Atmos. Chem. Phys., 10, 6487–6500, https://doi.org/10.5194/acp-10-6487-2010, 2010.
Lin, P., Rincon, A. G., Kalberer, M., and Yu, J. Z.: Elemental composition of HULIS in the Pearl River Delta Region, China: results inferred from positive and negative electrospray high resolution mass spectrometric data, Environ. Sci. Technol., 46, 7454–7462, https://doi.org/10.1021/es300285d, 2012.
Lin, P., Bluvshtein, N., Rudich, Y., Nizkorodov, S. A., Laskin, J., and Laskin, A.: Molecular chemistry of atmospheric brown carbon inferred from a nationwide biomass burning event, Environ. Sci. Technol., 51, 11561–11570, https://doi.org/10.1021/acs.est.7b02276, 2017.
Lin, P., Fleming, L. T., Nizkorodov, S. A., Laskin, J., and Laskin, A.: Comprehensive molecular characterization of atmospheric brown carbon by high resolution mass spectrometry with electrospray and atmospheric pressure photoionization, Anal. Chem., 90, 12493–12502, https://doi.org/10.1021/acs.analchem.8b02177, 2018.
Liu, J., Lin, P., Laskin, A., Laskin, J., Kathmann, S. M., Wise, M., Caylor, R., Imholt, F., Selimovic, V., and Shilling, J. E.: Optical properties and aging of light-absorbing secondary organic aerosol, Atmos. Chem. Phys., 16, 12815–12827, https://doi.org/10.5194/acp-16-12815-2016, 2016.
Liu, J., Mo, Y., Ding, P., Li, J., Shen, C., and Zhang, G.: Dual carbon isotopes (14C and 13C) and optical properties of WSOC and HULIS-C during winter in Guangzhou, China, Sci. Total Environ., 633, 1571–1578, https://doi.org/10.1016/j.scitotenv.2018.03.293, 2018.
Ma, Y., Cheng, Y., Qiu, X., Cao, G., Kuang, B., Yu, J. Z., and Hu, D.: Optical properties, source apportionment and redox activity of humic-like substances (HULIS) in airborne fine particulates in Hong Kong, Environ. Pollut., 255, 113087, https://doi.org/10.1016/j.envpol.2019.113087, 2019.
Mo, Y., Li, J., Jiang, B., Su, T., Geng, X., Liu, J., Jiang, H., Shen, C., Ding, P., Zhong, G., Cheng, Z., Liao, Y., Tian, C., Chen, Y., and Zhang, G.: Sources, compositions, and optical properties of humic-like substances in Beijing during the 2014 APEC summit: Results from dual carbon isotope and Fourier-transform ion cyclotron resonance mass spectrometry analyses, Environ. Pollut., 239, 322–331, https://doi.org/10.1016/j.envpol.2018.04.041, 2018.
Mohr, C., Lopez-Hilfiker, F. D., Zotter, P., Prevot, A. S., Xu, L., Ng, N. L., Herndon, S. C., Williams, L. R., Franklin, J. P., Zahniser, M. S., Worsnop, D. R., Knighton, W. B., Aiken, A. C., Gorkowski, K. J., Dubey, M. K., Allan, J. D., and Thornton, J. A.: Contribution of nitrated phenols to wood burning brown carbon light absorption in Detling, United Kingdom during winter time, Environ. Sci. Technol., 47, 6316–6324, https://doi.org/10.1021/es400683v, 2013.
Ni, H., Huang, R. J., Pieber, S. M., Corbin, J. C., Stefenelli, G., Pospisilova, V., Klein, F., Gysel-Beer, M., Yang, L., Baltensperger, U., Haddad, I. E., Slowik, J. G., Cao, J., Prevot, A. S. H., and Dusek, U.: Brown carbon in primary and aged coa lcombustion emission, Environ. Sci. Technol., 55, 5701–5710, https://doi.org/10.1021/acs.est.0c08084, 2021.
Park, S., Yua, G.-H., and Lee, S.: Optical absorption characteristics of brown carbon aerosols during the KORUS-AQ campaign at an urban site, Atmos. Res., 203, 16–27, https://doi.org/10.1016/j.atmosres.2017.12.002, 2018.
Riva, M., Robinson, E. S., Perraudin, E., Donahue, N. M., and Villenave, E.: Photochemical Aging of Secondary Organic Aerosols Generated from the Photooxidation of Polycyclic Aromatic Hydrocarbons in the Gas-Phase, Environ. Sci. Technol., 49, 5407–5416, https://doi.org/10.1021/acs.est.5b00442, 2015.
Saleh, R., Hennigan, C. J., McMeeking, G. R., Chuang, W. K., Robinson, E. S., Coe, H., Donahue, N. M., and Robinson, A. L.: Absorptivity of brown carbon in fresh and photo-chemically aged biomass-burning emissions, Atmos. Chem. Phys., 13, 7683–7693, https://doi.org/10.5194/acp-13-7683-2013, 2013.
Shen, Z., Zhang, Q., Cao, J., Zhang, L., Lei, Y., Huang, Y., Huang, R. J., Gao, J., Zhao, Z., Zhu, C., Yin, X., Zheng, C., Xu, H., and Liu, S.: Optical properties and possible sources of brown carbon in PM2.5 over Xi'an, China, Atmos. Environ., 150, 322–330, https://doi.org/10.1016/j.atmosenv.2016.11.024, 2017.
Song, J.: Data for SJ, Harvard Dataverse [data set], https://doi.org/10.7910/DVN/DYGYQT, 2022.
Song, J. Z., Li, M. J., Jiang, B., Wei, S. Y., Fan, X. J., and Peng, P. A.: Molecular Characterization of Water-Soluble Humic like substances in smoke particles emitted from combustion of biomass materials and coal using ultrahigh-resolution electrospray ionization Fourier transform ion cyclotron resonance mass spectrometry, Environ. Sci. Technol., 52, 2575–2585, https://doi.org/10.1021/acs.est.7b06126, 2018.
Song, J. Z., Li, M. J., Fan, X. J., Zou, C. L., Zhu, M. B., Jiang, B., Yu, Z. Q., Jia, W. L., Liao, Y. H., and Peng, P. A.: Molecular characterization of water- and methanol-soluble organic compounds emitted from residential coal combustion using ultrahigh-resolution electrospray ionization Fourier transform ion cyclotron resonance mass spectrometry, Environ. Sci. Technol., 53, 13607–13617, https://doi.org/10.1021/acs.est.9b04331, 2019.
Song, J. Z., Li, M. J., Zou, C. L., Cao, T., Fan, X. J., Jiang, B., Yu, Z. Q., Jia, W. L., and Peng, P. A.: Molecular characterization of nitrogen-containing compounds in humic-like substances emitted from biomass burning and coal combustion, Environ. Sci. Technol., 56, 119–130, https://doi.org/10.1021/acs.est.1c04451, 2022.
Sumlin, B. J., Pandey, A., Walker, M. J., Pattison, R. S., Williams, B. J., and Chakrabarty, R. K.: Atmospheric photooxidation diminishes light absorption by primary brown carbon aerosol from biomass burning, Environ. Sci. Tech. Let., 4, 540–545, https://doi.org/10.1021/acs.estlett.7b00393, 2017.
Surratt, J. D., Gomez-Gonzalez, Y., Chan, A. W. H., Vermeylen, R., Shahgholi, M., Kleindienst, T. E., Edney, E. O., Offenberg, J. H., Lewandowski, M., Jaoui, M., Maenhaut, W., Claeys, M., Flagan, R. C., and Seinfeld, J. H.: Organosulfate formation in biogenic secondary organic aerosol, J. Phys. Chem. A, 112, 8345–8378, https://doi.org/10.1021/jp802310p, 2008.
Tan, J., Xiang, P., Zhou, X., Duan, J., Ma, Y., He, K., Cheng, Y., Yu, J., and Querol, X.: Chemical characterization of humic-like substances (HULIS) in PM2.5 in Lanzhou, China, Sci. Total Environ., 573, 1481–1490, https://doi.org/10.1016/j.scitotenv.2016.08.025, 2016.
Tang, J., Li, J., Su, T., Han, Y., Mo, Y., Jiang, H., Cui, M., Jiang, B., Chen, Y., Tang, J., Song, J., Peng, P., and Zhang, G.: Molecular compositions and optical properties of dissolved brown carbon in biomass burning, coal combustion, and vehicle emission aerosols illuminated by excitation–emission matrix spectroscopy and Fourier transform ion cyclotron resonance mass spectrometry analysis, Atmos. Chem. Phys., 20, 2513–2532, https://doi.org/10.5194/acp-20-2513-2020, 2020.
Tomaz, S., Cui, T., Chen, Y., Sexton, K. G., Roberts, J. M., Warneke, C., Yokelson, R. J., Surratt, J. D., and Turpin, B. J.: Photochemical Cloud Processing of primary wildfire emissions as a ptential source of secondary organic Aerosol, Environ. Sci. Technol., 52, 11027–11037, https://doi.org/10.1021/acs.est.8b03293, 2018.
Wang, J., Wang, G., Gao, J., Wang, H., Ren, Y., Li, J., Zhou, B., Wu, C., Zhang, L., Wang, S., and Chai, F.: Concentrations and stable carbon isotope compositions of oxalic acid and related SOA in Beijing before, during, and after the 2014 APEC, Atmos. Chem. Phys., 17, 981–992, https://doi.org/10.5194/acp-17-981-2017, 2017.
Wang, X., Hayeck, N., Brüggemann, M., Abis, L., Riva, M., Lu, Y., Wang, B., Chen, J., George, C., and Wang, L.: Chemical characteristics and brown carbon chromophores of atmospheric organic aerosols over the Yangtze River channel: a cruise campaign, J. Geophys. Res.-Atmos., 125, e2020JD032497, https://doi.org/10.1029/2020jd032497, 2020.
Wang, X. K., Hayeck, N., Bruggemann, M., Yao, L., Chen, H. F., Zhang, C., Emmelin, C., Chen, J. M., George, C., and Wang, L.: Chemical characteristics of organic aerosols in shanghai: a study by ultrahigh-performance liquid chromatography coupled with orbitrap mass spectrometry, J. Geophys. Res.-Atmos., 122, 11703–11722, https://doi.org/10.1002/2017jd026930, 2017.
Wang, Y., Hu, M., Lin, P., Tan, T., Li, M., Xu, N., Zheng, J., Du, Z., Qin, Y., Wu, Y., Lu, S., Song, Y., Wu, Z., Guo, S., Zeng, L., Huang, X., and He, L.: Enhancement in particulate organic nitrogen and light absorption of humic-like substances over Tibetan Plateau due to long-range transported biomass burning emissions, Environ. Sci. Technol., 53, 14222–14232, https://doi.org/10.1021/acs.est.9b06152, 2019.
Wong, J. P. S., Nenes, A., and Weber, R. J.: Changes in Light Absorptivity of Molecular Weight Separated Brown Carbon Due to Photolytic Aging, Environ. Sci. Technol., 51, 8414–8421, https://doi.org/10.1021/acs.est.7b01739, 2017.
Wu, Z., Wang, Y., Tan, T., Zhu, Y., Li, M., Shang, D., Wang, H., Lu, K., Guo, S., Zeng, L., and Zhang, Y.: Aerosol liquid water driven by anthropogenic inorganic salts: implying its key role in haze formation over the North China Plain, Environ. Sci. Tech. Let., 5, 160–166, https://doi.org/10.1021/acs.estlett.8b00021, 2018.
Yang, X., Lu, K., Ma, X., Gao, Y., Tan, Z., Wang, H., Chen, X., Li, X., Huang, X., He, L., Tang, M., Zhu, B., Chen, S., Dong, H., Zeng, L., and Zhang, Y.: Radical chemistry in the Pearl River Delta: observations and modeling of OH and HO2 radicals in Shenzhen in 2018, Atmos. Chem. Phys., 22, 12525–12542, https://doi.org/10.5194/acp-22-12525-2022, 2022.
Yu, L., Smith, J., Laskin, A., George, K. M., Anastasio, C., Laskin, J., Dillner, A. M., and Zhang, Q.: Molecular transformations of phenolic SOA during photochemical aging in the aqueous phase: competition among oligomerization, functionalization, and fragmentation, Atmos. Chem. Phys., 16, 4511–4527, https://doi.org/10.5194/acp-16-4511-2016, 2016.
Yu, X., Yu, Q., Zhu, M., Tang, M., Li, S., Yang, W., Zhang, Y., Deng, W., Li, G., Yu, Y., Huang, Z., Song, W., Ding, X., Hu, Q., Li, J., Bi, X., and Wang, X.: Water soluble organic nitrogen (WSON) in ambient fine particles over a megacity in South China: spatiotemporal variations and source apportionment, J. Geophys. Res.-Atmos., 122, 13045–13060, https://doi.org/10.1002/2017jd027327, 2017.
Yuan, W., Huang, R. J., Yang, L., Ni, H., Wang, T., Cao, W., Duan, J., Guo, J., Huang, H., and Hoffmann, T.: Concentrations, optical properties and sources of humic-like substances (HULIS) in fine particulate matter in Xi'an, Northwest China, Sci. Total Environ., 789, 147902, https://doi.org/10.1016/j.scitotenv.2021.147902, 2021.
Zeng, Y., Shen, Z., Takahama, S., Zhang, L., Zhang, T., Lei, Y., Zhang, Q., Xu, H., Ning, Y., Huang, Y., Cao, J., and Rudolf, H.: Molecular Absorption and Evolution Mechanisms of PM2.5 Brown carbon revealed by electrospray ionization Fourier transform–ion cyclotron resonance mass spectrometry during a severe winter pollution episode in Xi'an, China, Geophys. Res. Lett., 47, e2020GL087977, https://doi.org/10.1029/2020gl087977, 2020.
Zhan, Y., Li, J., Tsona, N. T., Chen, B., Yan, C., George, C., and Du, L.: Seasonal variation of water-soluble brown carbon in Qingdao, China: Impacts from marine and terrestrial emissions, Environ. Res., 212, 113144, https://doi.org/10.1016/j.envres.2022.113144, 2022.
Zhang, J., Liu, L., Xu, L., Lin, Q., Zhao, H., Wang, Z., Guo, S., Hu, M., Liu, D., Shi, Z., Huang, D., and Li, W.: Exploring wintertime regional haze in northeast China: role of coal and biomass burning, Atmos. Chem. Phys., 20, 5355–5372, https://doi.org/10.5194/acp-20-5355-2020, 2020.
Zhang, L., Wang, G., Wang, J., Wu, C., Cao, C., and Li, J.: Chemical composition of fine particulate matter and optical properties of brown carbon before and during heating season in Xi'an, Journal of Earth Environment, 8, 451–458, 2017.
Zhang, Q., Worsnop, D. R., Canagaratna, M. R., and Jimenez, J. L.: Hydrocarbon-like and oxygenated organic aerosols in Pittsburgh: insights into sources and processes of organic aerosols, Atmos. Chem. Phys., 5, 3289–3311, https://doi.org/10.5194/acp-5-3289-2005, 2005.
Zhang, Y.-L., El-Haddad, I., Huang, R.-J., Ho, K.-F., Cao, J.-J., Han, Y., Zotter, P., Bozzetti, C., Daellenbach, K. R., Slowik, J. G., Salazar, G., Prévôt, A. S. H., and Szidat, S.: Large contribution of fossil fuel derived secondary organic carbon to water soluble organic aerosols in winter haze in China, Atmos. Chem. Phys., 18, 4005–4017, https://doi.org/10.5194/acp-18-4005-2018, 2018.
Zhong, M. and Jang, M.: Dynamic light absorption of biomass-burning organic carbon photochemically aged under natural sunlight, Atmos. Chem. Phys., 14, 1517–1525, https://doi.org/10.5194/acp-14-1517-2014, 2014.
Zhu, C. S., Cao, J. J., Huang, R. J., Shen, Z. X., Wang, Q. Y., and Zhang, N. N.: Light absorption properties of brown carbon over the southeastern Tibetan Plateau, Sci. Total Environ., 625, 246–251, https://doi.org/10.1016/j.scitotenv.2017.12.183, 2018.
Zou, C., Li, M., Cao, T., Zhu, M., Fan, X., Peng, S., Song, J., Jiang, B., Jia, W., Yu, C., Song, H., Yu, Z., Li, J., Zhang, G., and Peng, P.: Comparison of solid phase extraction methods for the measurement of humic-like substances (HULIS) in atmospheric particles, Atmos. Environ., 225, 117370, https://doi.org/10.1016/j.atmosenv.2020.117370, 2020.