the Creative Commons Attribution 4.0 License.
the Creative Commons Attribution 4.0 License.
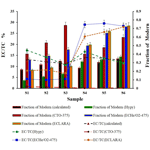
Technical note: Intercomparison study of the elemental carbon radiocarbon analysis methods using synthetic known samples
Xiangyun Zhang
Sanyuan Zhu
Junwen Liu
Ping Ding
Shutao Gao
Chongguo Tian
Yingjun Chen
Ping'an Peng
Gan Zhang
The accurate identification of elemental carbon (EC) sources in aerosol based on radiocarbon (14C) depends on the method of EC isolation. The lack of aerosol EC reference materials with “true” 14C values makes it impossible to evaluate the accuracy of various methods for the analysis of 14C-EC in aerosols. In this study, EC isolation methods were evaluated by using samples of mixed biomass burning, vehicle exhaust, and coal combustion. The results show that 14C-EC was not only related to the isolation method but also to the types and proportions of biomass sources in the sample. The hydropyrolysis (Hypy) method, which can be used to isolate a highly stable portion of ECHypy and avoid charring, is a more effective and stable approach for the matrix-independent 14C quantification of EC in aerosols. The 13C-ECHypy and non-fossil ECHypy values of Standard Reference Material (SRM) 1649b were −24.9 ‰ and 11 %, respectively.
- Article
(747 KB) -
Supplement
(823 KB) - BibTeX
- EndNote
The elemental carbon (EC) or black carbon (BC) fraction of carbonaceous aerosols (CAs) is derived from the incomplete combustion of fossil fuels or biomass and is responsible for an overall warming effect on the Earth by either absorbing incoming solar radiation in the atmosphere or reducing the albedo of surface materials (i.e., snow and ice; Fuzzi et al., 2006; Schwarz et al., 2015; Szidat, 2009; Szidat et al., 2004, 2009). The limited understanding of EC aerosol emissions results in poorly constrained estimates of their contribution to anthropogenic climate warming that may globally be second only to CO2 and may regionally, such as over East Asia, be the dominant driver of climate change (Chen et al., 2013). Therefore, detailed knowledge of the sources of EC is necessary for the implementation of mitigation strategies for EC reduction. Carbon isotope (14C and 13C) analysis is a powerful tool for unambiguously distinguishing the carbon sources of EC (Currie, 2000; Szidat, 2009; Szidat et al., 2009; Gustafsson et al., 2009; Kirillova et al., 2013; Liu et al., 2013; Zencak et al., 2007; Zhang et al., 2019b). Carbon isotope source apportionment requires the physical isolation of organic carbon (OC) and EC, which is complicated by the fact that there is no sharp boundary between OC and EC in carbonaceous aerosols (Elmquist et al., 2006). Therefore, one of the greatest challenges of this method is the isolation of EC for 14C and 13C analysis.
Based on the thermal stability of EC, several methods for isolating OC and EC from aerosols have been developed. An intercomparison of nine laboratories for 14C analysis of carbonaceous aerosol samples was conducted in a previous study, and 14C analysis of EC revealed a large deviation of 28 %–79 % between the approaches as a consequence of the different isolation techniques (Szidat et al., 2013). Due to the application of the same principle as aerosol OC and EC measurement, thermo-optical isolation, also named oxygen-based OC-EC isolation, has gradually become the main method for EC isolation in recent years. Although the 14C results of EC between three independent laboratory methods showed good agreement, the recovery of EC differed greatly (Zenker et al., 2017). Recently, hydropyrolysis (Hypy) has been introduced as an EC isolation method (Meredith et al., 2012; Zhang et al., 2019b). A comparison study of 14C-EC in aerosol samples, isolated using the two-step heating method (CTO-375), EC method, and Hypy method, was also conducted (Zhang et al., 2019b). However, the 14C intercomparisons of all studies were mainly restricted to The ambient filter samples or urban dust (SRM 1649a/b), for which the “true” 14C activity of EC is not known. As the literature emphasizes (Dasari and Widory, 2022), even when methods give similar results, it may still be unclear whether the methods give accurate results. In the worst case scenario, if the methods give different results, then it is impossible to determine which method (if any) gives an accurate value (Zenker et al., 2017). Therefore, the key to evaluating the accuracy of different isolation methods is to obtain suitable EC reference materials for the 14C analysis of aerosols.
Carbonaceous aerosols are mainly composed of primary emissions from fossil fuel and biomass combustion and secondary organic compounds (Huang et al., 2014; Zhang et al., 2015). In general, secondary organic aerosols are relatively easy to isolate from EC, using methods such as water or organic solvent extraction. However, it is difficult to isolate insoluble OC from EC in primary combustion products. Biomass burning, coal combustion, and traffic emissions are the main primary sources of EC in aerosols (Bond et al., 2013). In this study, six samples were synthesized artificially by using biomass combustion (corn straw or pine wood), coal combustion, and motor vehicle exhaust samples, according to the relative content of fossil carbon and modern carbon in actual aerosols in this study. The theoretically calculated values of the EC contents and EC carbon isotopes in six synthetic samples were determined based on the measured isotopes of each source sample and the elemental carbon and/or total carbon () measured by using the thermo-optical transmittance (TOT) method. And the calculated 14C value of EC can be taken as the true 14C-EC value. Consequently, four EC isolation methods, including Hypy (Zhang et al., 2019b), CTO-375 (Liu et al., 2013), EC (Liu et al., 2017), and ECLARA (Zenker et al., 2017), were selected for EC isolation, and then the EC contents and EC carbon isotopes were compared to the corresponding theoretically calculated value of each synthetic sample. The accuracy of each isolation method was evaluated based on the recovery of the EC contents and carbon isotopes. Finally, the EC contents and EC carbon isotopes (14C and 13C) of urban dust (SRM 1649b) were determined by the isolation method with the best accuracy.
2.1 Sample collection
Corn straw (Zea mays; C4 plant, with a carbon isotope composition that differs significantly from fossil fuels), pine wood (Pinus tabulaeformis Carr.; woody plant), one type of raw coal in chunks sourced from Yanzhou (YZ) in Shandong province, and one type of gasoline truck exhaust were selected to be the representative sources for biomass burning, coal combustion, and vehicle exhaust. Corn straw and pine wood combustion products were collected through a sampling system. Coal was combusted in a high-efficiency stove, and PM2.5 emissions were collected using a dilution sampling system. Vehicle exhaust particles were collected using the on-board emission measurement system. A description of the detailed sampling information was provided in a previous report (Zhang et al., 2019b).
2.2 EC isolation method
-
CTO-375 method. To achieve the complete removal of the OC from the ECCTO375 fraction, the samples were treated by vaporizing the OC at 375 ∘C in a muffle furnace in the presence of air, using a shorter isolation time of 4 h (Liu et al., 2013).
-
EC method. The EC fractions in the samples were purified in the commercial OC-EC analyzer as follows: 120 s at 200 ∘C, 150 s at 300 ∘C, and 180 s at 475 ∘C in an oxidative atmosphere (10 % oxygen and 90 % helium), followed by 180 s at 650 ∘C in helium. Details of the handling methods were described in a previous report (Liu et al., 2017).
-
ECLARA method. A punch of the water-extracted filter was treated with a thermo-optical OC-EC analyzer, using the first three steps of the Swiss 4S protocol to remove all remaining water-insoluble OC, giving a residue that constituted the ECLARA sample (Zenker et al., 2017; Zhang et al., 2015).
-
Hydropyrolysis. Each sample was mixed fully with ammonium dioxydithiomolybdate [(NH4)2MoO2S2] as a catalyst to reach a nominal molybdenum loading of more than 20 % of the sample carbon weight. The samples were first heated in the reactor tube, from ambient temperature to 250 ∘C, at a rate of 300 ∘C min−1 and then from 300 ∘C to the final temperature (550 ∘C) at 8 ∘C min−1; samples were then held for 5 min under a hydrogen pressure of 15 MPa and a flow rate of 5.0 L min−1. The resulting residue was the ECHypy sample (Zhang et al., 2019b).
2.3 and carbon isotopes analysis
The OC and EC were analyzed by a laboratory analyzer (Sunset Laboratory, Inc., USA) using the NIOSH2 thermal protocol (Maenhaut et al., 2005; Salma et al., 2004). The methods of the 13C and 14C analysis for all samples are described in the Supplement.
2.4 Nuclear magnetic resonance and field emission scanning electron microscopy analysis
NMR experiments were performed with an Avance III 400 MHz nuclear magnetic resonance (NMR) spectrometer (Bruker Scientific LLC, Billerica, MA, USA). Field emission scanning electron microscopy (FESEM) experiments were analyzed by the field emission scanning electron microscope (Hitachi su8010; Hitachi, Japan). Detailed experimental methods of NMR and FESEM analysis are described in the Supplement.
3.1 Comparison of EC purified by different methods
The six synthetic known samples were made by biomass combustion (corn straw or pine wood), coal combustion, and motor vehicle exhaust (Table S1 in the Supplement). The hybrid samples were produced according to the proportioning principle, based on the approximate proportions (Fig. S1 and Table S2). The average deviations of carbon content, 13CTC, and fM (TC) of the hybrid samples between the theoretical values and the test values were 0.30 %, −0.12 ‰, and 0.03, respectively (Table S3), and there was no significant difference (T test; P=0.77, 0.96, and 0.49, respectively). These results show that the samples were well mixed and were therefore suitable for the method comparison experiments.
Before comparing the EC recovery rates, it is necessary to obtain relatively accurate EC concentrations. The ratios of the four combustion source samples were analyzed by the TOT method (Table S4). The results of the four combustion source samples processed using the four isolation methods (CTO-375, EC, ECLARA, and Hypy) are listed in Table S4. The amount of EC obtained by the CTO-375 method is obviously lower than the results of the other three methods. For example, due to the high content of soot in the vehicle exhaust, the amount of EC can reach about 20 %, while the amount of EC in other source samples is less than 10 % (Hammes et al., 2007). It indicates that the CTO-375 method has obvious defects in the quantitative analysis of EC content in aerosols. Therefore, this method is not suitable for analysis involving the isolation of EC to isotopes. The ratios of the coal combustion and motor vehicle exhaust obtained by the other three methods are lower than those of the TOT method, and the ratios of the pine burning samples are higher than those of the TOT method. Among the above four methods, the result obtained by the Hypy method is the closest to the result of the TOT method. For the corn straw combustion samples, the Hypy and ECLARA methods are lower than the TOT method, but the results obtained by the EC are higher than the TOT method. On the one hand, this difference is the problem of the method itself. Each method only isolates a specific part of the EC continuum, rather than all of the components of the EC continuum (Currie et al., 2002; de la Rosa et al., 2011; Schmidt et al., 2001). On the other hand, the different isolation effect of the method is due to the difference in the organic carbon structure in the source sample.
NMR spectroscopy is an essential tool for acquiring the detailed structural characterization results of the complex natural organic matter. The four combustion source samples were characterized by using solid-state 13C NMR (Fig. S2 and Table S5). The 13C NMR results show that the average lower-limit estimate for organic oxygen (Kelemen et al., 2010) from biomass combustion is 37 % higher than that for fossil combustion. This finding indicates that there are more oxygen-containing organic carbon components in biomass combustion samples. The more organic oxygen in the sample, the greater the sample's polarity, which contributes to the increased fraction of water-soluble components in biomass combustion compared to the fossil combustion samples. In the absence of oxygen, using an aromatization process based on the cleavage of O-alkylated carbons might overestimate the EC content analyzed by thermo-optical methods (Li et al., 2013). The fraction of methyls in the aliphatics (FMA; Chen et al., 2020) and non-protonated aromatics (Kelemen et al., 2010) in pine wood combustion are 22 % and 12 % higher, respectively, than those in corn straw. These findings indicate that the water solubility of pine wood combustion products is worse than that of corn straw, in addition to the fact that the aromatic structure of the pine wood combustion materials is denser. The water-soluble components of the corn straw combustion products were high, such that OC charring has greatly influenced EC isolation when using the EC method, while minimally impacting EC isolation following the water extraction ECLARA method (Zhang et al., 2012).
By using FESEM in this study (Fig. S3), it was observed that the pine wood samples contained a coke structure that was more condensed than the structure of the corn straw samples. In addition, a large number of soot structures were observed in the fossil source samples, indicating that the fossil source samples are more condensed than biomass samples.
In general, due to the differences between the isolation technologies, the ratios of the six synthetic known samples (Table S4) revealed a deviation of approximately −99 %–+125 % between the calculated ratios and the ratios isolated by the four methods. The deviations isolated by the Hypy, ECLARA, EC, and CTO-375 methods were approximately −8 %–+31 %, −39 %–+121 %, −62 %–+125 %, and −93 %–−99 %, respectively. The result obtained by the Hypy method is closest to the TOT method, and the average value of the ratio is 1.1, which shows the advantages of the stability and reliability of purifying EC.
Table 1The δ13C and 14C results of EC from the six synthetic known samples by four isolation methods.

3.2 Comparison of carbon isotopes in EC
The different EC recoveries of the source samples may lead to different carbon isotope results in the EC from the synthetic known samples. The theoretical ratios and 13CEC values of each hybrid sample were calculated according to the (TOT) ratios and 13CTC values in the combustion source and the relative proportion of each source, respectively (Table S3). In the calculation, it is assumed that the 13C value in the EC is very close to that in the TC in each source sample. The 13C results of the EC from the six synthetic known samples processed by the four isolation methods are shown in Table 1. No significant difference in the 13C was observed between the results of the four isolation methods and the corresponding theoretical calculation values of all samples (T test; P>0.05), except for the samples (S1, S2, and S3) containing corn straw combustion material isolated using the EC method, which presented much higher 13C values. This finding indicated that the EC isolated using the EC method contained more biomass carbon. The reason for this result may be that the organic carbon of combusted corn straw is charring during the EC isolation process. However, this phenomenon was not observed in the samples containing pine wood combustion, which may be the reason that the 13C value of the pine wood combustion sample is close to that of coal combustion.
The 14C results of the EC are shown in Table 1 and Fig. 1. The Fraction of Modern (fM) is used to express the proportion of biomass burning. The theoretical values of fM (EC) in the hybrid samples were obtained according to the proportion of fossil carbon and modern carbon in each sample, in accordance with the (TOT) ratios (Fig. 1). The results show that the fM values obtained by different isolation methods are quite different and are generally affected by the ratios of the combustion source sample EC recovered by different methods. Due to the low recovery rate of EC by the CTO-375 method, the fM (ECCTO−375) value is irregular. Generally, the fM (ECCTO−375) value obtained by this method is more than twice the theoretical value. For the EC method, due to the influence of biomass burning OC charring, the fM (EC value obtained by this method deviates greatly from the theoretical value. On the whole, fM values obtained by the Hypy and ECLARA methods are relatively close to the theoretical values, but the two methods have their own advantages in the two different sets of samples. For the combustion of herbaceous plants, the Hypy method has a low EC recovery rate for such source samples, resulting in a small fM value. For the combustion of woody plants, due to the higher EC recovery rate by the Hypy method, the fM (ECHypy) value is slightly higher than that of samples containing herbaceous plants. For the ECLARA method, in the first group of samples with corn straw combustion, the EC recovery rate of each source sample is lower than the theoretical value, so that the fM (ECLARA) value obtained by this method is the closest to the theoretical value. However, in the second group of samples containing woody combustion, the fM (ECLARA) value obtained by this method was significantly higher than the theoretical value, due to the higher recovery rate of woody combustion EC by the ECLARA method. The results show that the fM value of EC was not only related to the isolation method but also to the types and proportions of the biomass sources in the sample.
The above results show that the type of biomass combustion affects the efficiency of the isolation method when purifying black carbon and the accuracy of the radiocarbon test results. Charcoal, harvest residues, and woody materials are the most common biomass fuels used as energy sources (Anenberg et al., 2013). From a global perspective, the types of biomass fuels are complex but generally can be divided into two categories, namely herbaceous burning and woody plant burning, and the ratio of the two types of biomass is about 58:42 (Table S6). Different regions have different proportions. According to the literature (Bond et al., 2004; Stevens et al., 2017; Streets et al., 2003; Zhang et al., 2019a), developed countries, in regions such as Europe and North America, have a relatively high proportion of woody plants, while developing countries, in regions such as Africa and Asia, have a relatively high proportion of herbaceous plants (Table S6). According to the recovery rate of the different types of biomass combustion EC by different methods, the deviations that may be caused by the results of testing 14C in different regions are estimated. The results are listed in Table S6. It can be seen that, on a global scale, the fM value obtained by the Hypy method is the closest to the theoretical value. Therefore, the Hypy method is an effective and stable approach for the matrix-independent 14C quantification of EC avoiding charring in aerosols.
3.3 Carbon isotopes of EC in Standard Reference Material 1649b
Standard Reference Material (SRM) 1649a/b, urban dust, was used to check the quality of EC or EC isotope measurement method (Currie et al., 2002; Liu et al., 2013; Szidat et al., 2004; Reddy et al., 2002; Heal et al., 2011). SRM 1649b was prepared from the same particulate material that was issued in 1982 as SRM 1649 and re-issued in 1999 as SRM 1649a, and the only difference is that the bulk material was sieved to a smaller particle size fraction. Therefore, the fM and δ13C of the TC in SRM 1649b obtained in this study was consistent with SRM 1649a reported by Szidat et al. (2004). ratios, fM-EC, and δ13C-EC of SRM 1649b isolated using the Hypy method in this study and archived data from the literature using different isolation methods are listed in Table 2. The ratios varied from 7.5 % to 46 %, as determined by the different analyzing methods. However, the value of about ∼ 28 % obtained in this study is in good agreement with that obtained by the Hypy method (Meredith et al., 2012) and by the TOT method (Currie et al., 2002). The fM and δ13C of EC in SRM 1649a ranged from 0.038 to 0.153 and from −26.55‰ to −24.8‰, respectively, as reported by the previous research using the different isolation methods (except for Hypy; Currie et al., 2002). The corresponding values of 0.11 ‰ and −24.9 ‰ that are treated by Hypy method in this study are just in the range of the archived data. Therefore, the values of 13C and 14C of ECHypy in SRM 1649b provide a definite and comparable reference for future research methods.
Carbon isotope (14C and 13C) analysis is a powerful tool for distinguishing the carbon sources in carbonaceous aerosols. As addressed in this work, one main challenge of this method is the isolation of EC or BC for carbon isotope analysis. In this study, six synthetic known samples were collected, including biomass combustion (corn straw or pine wood), coal combustion products, and motor vehicle exhaust. These samples were then used to evaluate four EC isolation methods, which included the Hypy, CTO-375, EC, and ECLARA methods. The results demonstrated that the Hypy method was in good agreement with the thermo-optical (TOT) method for the quantification of EC. And the EC fM values depended not only on the isolation method but also on the types and proportions of the biomass sources in the samples. The Hypy method is the most appropriate EC isolation method of the four methods reported here, followed by the ECLARA method. The Hypy method, which can be used to isolate a highly stable portion of ECHypy and avoid charring, is a more effective and stable approach for the matrix-independent 14C quantification of EC in aerosols. The ECHypy of SRM 1649b sample was isolated by the Hypy method. The results indicated that the 13C-ECHypy and non-fossil ECHypy values of SRM 1649b were −24.9 ‰ and 11 %, respectively. These two isotope values was able to provide a valuable reference for other EC isolation methods.
The data described in this study are available in this paper (Tables 1, 2) and its Supplement (Tables S1, S2, S3, S4, S5, S6).
The supplement related to this article is available online at: https://doi.org/10.5194/acp-23-7495-2023-supplement.
GZ led the study. JL and XZ designed the study, developed the analysis protocols, and wrote the draft. XZ, SZ, JL, PD, SG, CT, YC, and PP provided data, provided comments on the analysis, and contributed to and reviewed the final paper.
The contact author has declared that none of the authors has any competing interests.
Publisher’s note: Copernicus Publications remains neutral with regard to jurisdictional claims in published maps and institutional affiliations.
This work was supported by the National Natural Science Foundation of China (grant nos. 42192511, 42030715 and 41977177), Guangdong Foundation for Program of Science and Technology Research (grant nos. 2017BT01Z134 and 2020B1212060053) and Guangzhou Foundation for Program of Science and Technology Research (grant no. 202102080251).
This paper was edited by Katye Altieri and reviewed by Will Meredith and one anonymous referee.
Anenberg, S. C., Balakrishnan, K., Jetter, J., Masera, O., Mehta, S., Moss, J., and Ramanathan, V.: Cleaner Cooking Solutions to Achieve Health, Climate, and Economic Cobenefits, Environ. Sci. Technol., 47, 3944–3952, https://doi.org/10.1021/es304942e, 2013.
Bond, T. C., Streets, D. G., Yarber, K. F., Nelson, S. M., Woo, J. H., and Klimont, Z.: A technology-based global inventory of black and organic carbon emissions from combustion, J. Geophys. Res.-Atmos., 109, 43, https://doi.org/10.1029/2003jd003697, 2004.
Bond, T. C., Doherty, S. J., Fahey, D. W., Forster, P. M., Berntsen, T., DeAngelo, B. J., Flanner, M. G., Ghan, S., Kaercher, B., Koch, D., Kinne, S., Kondo, Y., Quinn, P. K., Sarofim, M. C., Schultz, M. G., Schulz, M., Venkataraman, C., Zhang, H., Zhang, S., Bellouin, N., Guttikunda, S. K., Hopke, P. K., Jacobson, M. Z., Kaiser, J. W., Klimont, Z., Lohmann, U., Schwarz, J. P., Shindell, D., Storelvmo, T., Warren, S. G., and Zender, C. S.: Bounding the role of black carbon in the climate system: A scientific assessment, J. Geophys. Res.-Atmos., 118, 5380–5552, https://doi.org/10.1002/jgrd.50171, 2013.
Chen, B., Andersson, A., Lee, M., Kirillova, E. N., Xiao, Q. F., Krusa, M., Shi, M. N., Hu, K., Lu, Z. F., Streets, D. G., Du, K., and Gustafsson, O.: Source Forensics of Black Carbon Aerosols from China, Environ. Sci. Technol., 47, 9102–9108, https://doi.org/10.1021/es401599r, 2013.
Chen, J., Jia, W. L., Yu, C. L., Zhang, X. Y., and Peng, P. A.: Bound hydrocarbons and structure of pyrobitumen rapidly formed by asphaltene cracking: Implications for oil-source correlation, Org. Geochem., 146, 16, https://doi.org/10.1016/j.orggeochem.2020.104053, 2020.
Currie, L. A.: Evolution and multidisciplinary frontiers of C-14 aerosol science, Radiocarbon, 42, 115–126, https://doi.org/10.1017/S003382220005308X, 2000.
Currie, L. A., Benner, B. A., Kessler, J. D., Klinedinst, D. B., Klouda, G. A., Marolf, J. V., Slater, J. F., Wise, S. A., Cachier, H., Cary, R., Chow, J. C., Watson, J., Druffel, E. R. M., Masiello, C. A., Eglinton, T. I., Pearson, A., Reddy, C. M., Gustafsson, O., Quinn, J. G., Hartmann, P. C., Hedges, J. I., Prentice, K. M., Kirchstetter, T. W., Novakov, T., Puxbaum, H., and Schmid, H.: A critical evaluation of interlaboratory data on total, elemental, and isotopic carbon in the carbonaceous particle reference material, NIST SRM 1649a, J. Res. Natl. Inst. Stand. Technol., 107, 279–298, https://doi.org/10.6028/jres.107.022, 2002.
Dasari, S. and Widory, D.: Radiocarbon (14C) Analysis of Carbonaceous Aerosols: Revisiting the Existing Analytical Techniques for Isolation of Black Carbon, Front. Environ. Sci., 10, 907467, https://doi.org/10.3389/fenvs.2022.907467, 2022.
de la Rosa, J. M., Garcia, L. S., de Andres, J. R., Gonzalez-Vila, F. J., Gonzalez-Perez, J. A., and Knicker, H.: Contribution of black carbon in recent sediments of the Gulf of Cadiz: Applicability of different quantification methodologies, Quaternary Int., 243, 264–272, https://doi.org/10.1016/j.quaint.2011.01.034, 2011.
Elmquist, M., Cornelissen, G., Kukulska, Z., and Gustafsson, O.: Distinct oxidative stabilities of char versus soot black carbon: Implications for quantification and environmental recalcitrance, Glob. Biogeochem. Cy., 20, 11, https://doi.org/10.1029/2005gb002629, 2006.
Fuzzi, S., Andreae, M. O., Huebert, B. J., Kulmala, M., Bond, T. C., Boy, M., Doherty, S. J., Guenther, A., Kanakidou, M., Kawamura, K., Kerminen, V.-M., Lohmann, U., Russell, L. M., and Pöschl, U.: Critical assessment of the current state of scientific knowledge, terminology, and research needs concerning the role of organic aerosols in the atmosphere, climate, and global change, Atmos. Chem. Phys., 6, 2017–2038, https://doi.org/10.5194/acp-6-2017-2006, 2006.
Gustafsson, O., Krusa, M., Zencak, Z., Sheesley, R. J., Granat, L., Engstrom, E., Praveen, P. S., Rao, P. S. P., Leck, C., and Rodhe, H.: Brown Clouds over South Asia: Biomass or Fossil Fuel Combustion?, Science, 323, 495–498, https://doi.org/10.1126/science.1164857, 2009.
Hammes, K., Schmidt, M. W. I., Smernik, R. J., Currie, L. A., Ball, W. P., Nguyen, T. H., Louchouarn, P., Houel, S., Gustafsson, O., Elmquist, M., Cornelissen, G., Skjemstad, J. O., Masiello, C. A., Song, J. Z., Peng, P. A., Mitra, S., Dunn, J. C., Hatcher, P. G., Hockaday, W. C., Smith, D. M., Hartkopf-Froeder, C., Boehmer, A., Luer, B., Huebert, B. J., Amelung, W., Brodowski, S., Huang, L., Zhang, W., Gschwend, P. M., Flores-Cervantes, D. X., Largeau, C., Rouzaud, J. N., Rumpel, C., Guggenberger, G., Kaiser, K., Rodionov, A., Gonzalez-Vila, F. J., Gonzalez-Perez, J. A., de la Rosa, J. M., Manning, D. A. C., Lopez-Capel, E., and Ding, L.: Comparison of quantification methods to measure fire-derived (black/elemental) carbon in soils and sediments using reference materials from soil, water, sediment and the atmosphere, Glob. Biogeochem. Cycle, 21, 18, https://doi.org/10.1029/2006gb002914, 2007.
Heal, M. R., Naysmith, P., Cook, G. T., Xu, S., Duran, T. R., and Harrison, R. M.: Application of C-14 analyses to source apportionment of carbonaceous PM2.5 in the UK, Atmos. Environ., 45, 2341–2348, https://doi.org/10.1016/j.atmosenv.2011.02.029, 2011.
Huang, R. J., Zhang, Y. L., Bozzetti, C., Ho, K. F., Cao, J. J., Han, Y. M., Daellenbach, K. R., Slowik, J. G., Platt, S. M., Canonaco, F., Zotter, P., Wolf, R., Pieber, S. M., Bruns, E. A., Crippa, M., Ciarelli, G., Piazzalunga, A., Schwikowski, M., Abbaszade, G., Schnelle-Kreis, J., Zimmermann, R., An, Z. S., Szidat, S., Baltensperger, U., El Haddad, I., and Prevot, A. S. H.: High secondary aerosol contribution to particulate pollution during haze events in China, Nature, 514, 218–222, https://doi.org/10.1038/nature13774, 2014.
Kelemen, S. R., Walters, C. C., Kwiatek, P. J., Freund, H., Afeworki, M., Sansone, M., Lamberti, W. A., Pottorf, R. J., Machel, H. G., Peters, K. E., and Bolin, T.: Characterization of solid bitumens originating from thermal chemical alteration and thermochemical sulfate reduction, Geochim. Cosmochim. Acta, 74, 5305–5332, https://doi.org/10.1016/j.gca.2010.06.013, 2010.
Kirillova, E. N., Andersson, A., Sheesley, R. J., Krusa, M., Praveen, P. S., Budhavant, K., Safai, P. D., Rao, P. S. P., and Gustafsson, O.: C-13- and C-14-based study of sources and atmospheric processing of water-soluble organic carbon (WSOC) in South Asian aerosols, J. Geophys. Res.-Atmos., 118, 614–626, https://doi.org/10.1002/jgrd.50130, 2013.
Li, X. M., Shen, Q. R., Zhang, D. Q., Mei, X. L., Ran, W., Xu, Y. C., and Yu, G. H.: Functional Groups Determine Biochar Properties (pH and EC) as Studied by Two-Dimensional C-13 NMR Correlation Spectroscopy, PLoS One, 8, 7, https://doi.org/10.1371/journal.pone.0065949, 2013.
Liu, D., Li, J., Zhang, Y. L., Xu, Y., Liu, X., Ding, P., Shen, C. D., Chen, Y. J., Tian, C. G., and Zhang, G.: The Use of Levoglucosan and Radiocarbon for Source Apportionment of PM2.5 Carbonaceous Aerosols at a Background Site in East China, Environ. Sci. Technol., 47, 10454–10461, https://doi.org/10.1021/es401250k, 2013.
Liu, J. W., Li, J., Ding, P., Zhang, Y. L., Liu, D., Shen, C. D., and Zhang, G.: Optimizing isolation protocol of organic carbon and elemental carbon for C-14 analysis using fine particulate samples, Atmos. Environ., 154, 9–19, https://doi.org/10.1016/j.atmosenv.2017.01.027, 2017.
Maenhaut, W., Raes, N., Chi, X. G., Cafmeyer, J., Wang, W., and Salma, I.: Chemical composition and mass closure for fine and coarse aerosols at a kerbside in Budapest, Hungary, in spring 2002, X-Ray Spectrom., 34, 290–296, https://doi.org/10.1002/xrs.820, 2005.
Meredith, W., Ascough, P. L., Bird, M. I., Large, D. J., Snape, C. E., Sun, Y., and Tilston, E. L.: Assessment of hydropyrolysis as a method for the quantification of black carbon using standard reference materials, Geochim. Cosmochim. Acta, 97, 131–147, https://doi.org/10.1016/j.gca.2012.08.037, 2012.
Reddy, C. M., Pearson, A., Xu, L., McNichol, A. P., Benner, B. A., Wise, S. A., Klouda, G. A., Currie, L. A., and Eglinton, T. I.: Radiocarbon as a tool to apportion the sources of polycyclic aromatic hydrocarbons and black carbon in environmental samples, Environ. Sci. Technol, 36, 1774–1782, https://doi.org/10.1021/es011343f, 2002.
Salma, I., Chi, X. G., and Maenhaut, W.: Elemental and organic carbon in urban canyon and background environments in Budapest, Hungary, Atmos. Environ., 38, 27–36, https://doi.org/10.1016/j.atmosenv.2003.09.047, 2004.
Schmidt, M. W. I., Skjemstad, J. O., Czimczik, C. I., Glaser, B., Prentice, K. M., Gelinas, Y., and Kuhlbusch, T. A. J.: Comparative analysis of black carbon in soils, Glob. Biogeochem. Cycle, 15, 163–167, https://doi.org/10.1029/2000gb001284, 2001.
Schwarz, J. P., Holloway, J. S., Katich, J. M., McKeen, S., Kort, E. A., Smith, M. L., Ryerson, T. B., Sweeney, C., and Peischl, J.: Black Carbon Emissions from the Bakken Oil and Gas Development Region, Environ. Sci. Technol. Lett., 2, 281–285, https://doi.org/10.1021/acs.estlett.5b00225, 2015.
Stevens, N., Lehmann, C. E. R., Murphy, B. P., and Durigan, G.: Savanna woody encroachment is widespread across three continents, Glob. Change Biol., 23, 235–244, https://doi.org/10.1111/gcb.13409, 2017.
Streets, D. G., Yarber, K. F., Woo, J. H., and Carmichael, G. R.: Biomass burning in Asia: Annual and seasonal estimates and atmospheric emissions, Glob. Biogeochem. Cycle, 17, 20, https://doi.org/10.1029/2003gb002040, 2003.
Szidat, S.: ATMOSPHERE Sources of Asian Haze, Science, 323, 470–471, https://doi.org/10.1126/science.1169407, 2009.
Szidat, S., Jenk, T. M., Gaggeler, H. W., Synal, H. A., Hajdas, I., Bonani, G., and Saurer, M.: THEODORE, a two-step heating system for the EC/OC determination of radiocarbon (C-14) in the environment, Nucl. Instrum. Methods Phys. Res. Sect. B-Beam Interact. Mater. Atoms, 223, 829–836, https://doi.org/10.1016/j.nimb.2004.04.153, 2004.
Szidat, S., Ruff, M., Perron, N., Wacker, L., Synal, H.-A., Hallquist, M., Shannigrahi, A. S., Yttri, K. E., Dye, C., and Simpson, D.: Fossil and non-fossil sources of organic carbon (OC) and elemental carbon (EC) in Göteborg, Sweden, Atmos. Chem. Phys., 9, 1521–1535, https://doi.org/10.5194/acp-9-1521-2009, 2009.
Szidat, S., Bench, G., Bernardoni, V., Calzolai, G., Czimczik, C. I., Derendorp, L., Dusek, U., Elder, K., Fedi, M. E., Genberg, J., Gustafsson, O., Kirillova, E., Kondo, M., McNichol, A. P., Perron, N., Santos, G. M., Stenstrom, K., Swietlicki, E., Uchida, M., Vecchi, R., Wacker, L., Zhang, Y. L., and Prevot, A. S. H.: INTERCOMPARISON OF C-14 ANALYSIS OF CARBONACEOUS AEROSOLS: EXERCISE 2009, Radiocarbon, 55, 1496–1509, https://doi.org/10.2458/azu_js_rc.55.16314, 2013.
Zencak, Z., Elmquist, M., and Gustafsson, O.: Quantification and radiocarbon source apportionment of black carbon in atmospheric aerosols using the CTO-375 method, Atmos. Environ., 41, 7895–7906, https://doi.org/10.1016/j.atmosenv.2007.06.006, 2007.
Zenker, K., Vonwiller, M., Szidat, S., Calzolai, G., Giannoni, M., Bernardoni, V., Jedynska, A. D., Henzing, B., Meijer, H. A. J., and Dusek, U.: Evaluation and Inter-Comparison of Oxygen-Based OC-EC Separation Methods for Radiocarbon Analysis of Ambient Aerosol Particle Samples, Atmosphere, 8, 21, https://doi.org/10.3390/atmos8110226, 2017.
Zhang, W. M., Brandt, M., Penuelas, J., Guichard, F., Tong, X. Y., Tian, F., and Fensholt, R.: Ecosystem structural changes controlled by altered rainfall climatology in tropical savannas, Nat. Commun., 10, 7, https://doi.org/10.1038/s41467-019-08602-6, 2019a.
Zhang, X. Y., Li, J., Mo, Y. Z., Shen, C. D., Ding, P., Wang, N., Zhu, S. Y., Cheng, Z. N., He, J. Z., Tian, Y. K., Gao, S. T., Zhou, Q., Tian, C. G., Chen, Y. J., and Zhang, G.: Isolation and radiocarbon analysis of elemental carbon in atmospheric aerosols using hydropyrolysis, Atmos. Environ., 198, 381-386, https://doi.org/10.1016/j.atmosenv.2018.11.005, 2019b.
Zhang, Y. L., Perron, N., Ciobanu, V. G., Zotter, P., Minguillón, M. C., Wacker, L., Prévôt, A. S. H., Baltensperger, U., and Szidat, S.: On the isolation of OC and EC and the optimal strategy of radiocarbon-based source apportionment of carbonaceous aerosols, Atmos. Chem. Phys., 12, 10841–10856, https://doi.org/10.5194/acp-12-10841-2012, 2012.
Zhang, Y. L., Schnelle-Kreis, J., Abbaszade, G., Zimmermann, R., Zotter, P., Shen, R. R., Schafer, K., Shao, L. Y., Prevot, A. S. H., and Szidat, S.: Source Apportionment of Elemental Carbon in Beijing, China: Insights from Radiocarbon and Organic Marker Measurements, Environ. Sci. Technol., 49, 8408–8415, https://doi.org/10.1021/acs.est.5b01944, 2015.