the Creative Commons Attribution 4.0 License.
the Creative Commons Attribution 4.0 License.
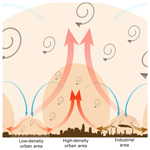
Opinion: Gigacity – a source of problems or the new way to sustainable development
Markku Kulmala
Tom V. Kokkonen
Juha Pekkanen
Sami Paatero
Tuukka Petäjä
Veli-Matti Kerminen
The eastern part of China as a whole is practically a gigacity; it is a conglomeration of megacities with circa 650 million inhabitants. The gigacity, with its emissions, processes in pollution cocktail, numerous feedbacks and interactions, has a crucial and big impact on regional air quality within itself and on global climate. A large-scale research and innovation program is needed to meet the interlinked grand challenges in this gigacity and to serve as a platform for finding pathways for sustainable development of the whole globe.
- Article
(2715 KB) - Full-text XML
- BibTeX
- EndNote
Humanity faces a multitude of severe global environmental changes, such as climate change, air, water and soil pollution, disturbances to food and water supplies and global epidemic diseases (Burnett et al., 2018; Figueres et al., 2017; Parrish and Zhu, 2009; Zhang et al., 2017). On a global scale, these environmental challenges are called grand challenges, which are the main factors controlling human wellbeing and security, as well as the stability of future societies. Since the grand challenges are highly connected and interlinked, they cannot be solved separately (Fig. 1). The main driving forces behind these are the growth of population and gross domestic production (GDP) globally, as well as the increasing urbanization, which is closely related to the former two.
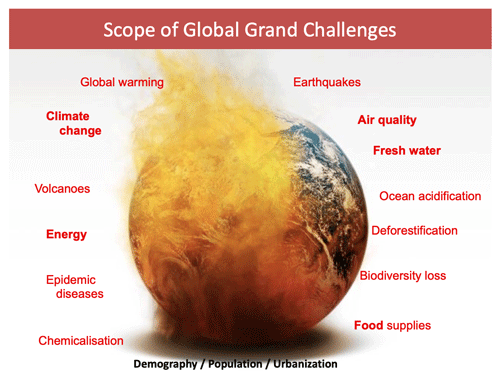
Figure 1The burning planet and the scope of interlinked grand challenges. The main driving forces behind these challenges are the growth of population and gross domestic production globally, as well as the growth of urbanization, which is closely related to the two former trends. The growing population needs clean air, more fresh water, food and energy, which will cause challenges such as climate change, declining air quality, ocean acidification, loss of biodiversity and shortages of fresh water, food and energy supplies, as well as regional and even global epidemic diseases.
Cities provide the citizens with many health services. These are, however, being counteracted with, e.g., increasing risks of cardiovascular diseases and diabetes, violence and injuries, outbreaks of infectious disease, like the current pandemic of COVID-19 (Tian et al., 2020), and inequity between people living in urban areas. These problems are especially acute in large and rapidly growing cities, which concurrently struggle to build sufficient infrastructure to provide clean air and water, energy, food, transportation, waste management and public spaces, all of which are essential to human wellbeing (Yang et al., 2018).
The urban sprawl is a major contributor to the destruction of natural, biologically diverse habitats and the current rapid decline in biodiversity (e.g., Liu et al., 2016). The cities rely on the surrounding countryside for several immediate ecosystem services (Ramaswami et al., 2016), and limited contact with nature has been shown to reduce human wellbeing. There are some indications that the loss of biodiversity favors the emergence of new infectious diseases and antibiotic resistance. In the long run, the survival of humankind depends on a diverse and functioning ecosystem.
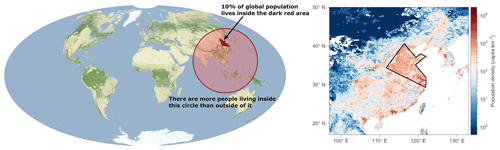
Figure 2Population density map, including the gigacity in eastern China. Half of the population of the world lives inside a circle that covers China, India and the South East Asian peninsula. Approximately 10 % of the world's population lives inside the triangle. This region is the most important economic driver of the world's economy, and the GDP, particularly in this gigacity, has increased very rapidly during the last few decades. The data are obtained from Stamen Design (CC-BY 3.0) and the Gridded Population of the World (GPW v4.11; CC-BY 4.0; CIESIN, 2018).
A clear example of future development is the rapid, large-scale urbanization of China, being, so far, unique in history (Zhao et al., 2015). Already about 10 % of the global population is living in the area of 1 Mkm2 in eastern China inside the triangle confined roughly by the lines Shanghai–Nanjing–Xian–Beijing–Shanghai (Fig. 2). The population is still increasing and gradually filling the less urbanized areas between the individual cities, so that this region is becoming practically one city – termed gigacity here – particularly from the grand challenges point of view. Clean air is lacking, the frequency of severe weather events with human casualties is increasing, biodiversity is going down and sources of food and water are polluted (Ding et al., 2017; Fang et al., 2018; Kulmala, 2015; Lu et al., 2019), with all this happening concurrently with an increasing demand for water, food and energy. Since this gigacity has roughly 50 times more people and 60 times larger surface area than Beijing – a typical megacity – its future is crucial not only for local people but also globally. The area is a huge emitter of greenhouse gases and air pollution as well as being a potential source for local, regional and global epidemiological diseases due to loss of biodiversity and a huge population density. What will happen there in the future will affect the whole globe.
On a positive note, as a starting point of the New Silk Road and economic Belt and Road Initiative, the Chinese gigacity could serve as an example for other, similar areas in the future on how to meet and even solve the grand challenges. On the one hand, the GDP of China has increased at a rate of several percent per year during the past few decades. On the other hand, the Chinese government has estimated that the GDP is reduced by 4 %–8 % due to air pollution, and it is very likely that other grand challenges have a similar impact, which underlines that there are economic incentives to solving the interconnected grand challenges.
Air pollution is recognized as being the biggest environmental challenge in China today (Huang et al., 2021; Parrish and Zhu, 2009; Zhang et al., 2017; Yang et al., 2018), and it is estimated to cause more than a million deaths annually in China (Zhang et al., 2017; Yang et al., 2018). Fine particulate air pollution, as the most harmful air pollutant (Burnett et al., 2018; Parrish and Zhu, 2009; Zhang et al., 2017), reduces life expectancy and increases the risk of cardiovascular and respiratory diseases. Transport causes 20 % to 40 % of air pollutant emissions in eastern China (Huang et al., 2014, 2021). Therefore, reduction in the amount of motorized transport could improve air quality, but such reductions might even cause negative impact on the air quality due to nonbalanced reduction, as was demonstrated, e.g., by Huang et al. (2021) with the data obtained during the COVID-19 lockdown in China. It is already well known that reduced concentrations of nitrogen oxides will, under otherwise polluted conditions, increase ozone production and increase secondary aerosol particle concentrations (Ding et al., 2013; Liu and Wang, 2020). The air quality can be further deteriorated due to aerosol–boundary layer–weather feedback (Huang et al., 2020). In the gigacity domain, nonlinear interactions between the atmospheric composition, meteorology, city planning or land use, human adaption and behavior need to be understood in a comprehensive and holistic way (Baklanov et al., 2018). Compared with megacities, the synergic effects of such interactions may result in much higher exposures and health effects in a gigacity. Future research is needed into this direction.
Although high-resolution modeling is capable of forecasting air quality and studying the urban climate phenomena inside a megacity with a grid scale of about 10 m, incorporating local-scale phenomena onto a gigacity scale is not straightforward as it requires scaling from 100 km up to more than 1000 km (Huang et al., 2020, 2021). This calls for a seamless modeling approach (Baklanov et al., 2018) that couples the urban atmosphere and hydrology driven by synoptic-scale numerical weather prediction models or even Earth system models. This further allows for bridging the gigacity environment to the continental scale, while retaining the micro- and mesoscale process-level understanding. Regional-scale climate and air quality are influenced by sources both outside and inside the gigacity area, such as windblown dust, biogenic emissions, biomass burning, traffic and industry (Ding et al., 2017; Huang et al., 2020). On a larger scale, the gigacity is a huge source of atmospheric pollution and anthropogenic heat. A combination of the urban heat island (UHI), increased surface roughness and aerosol–planetary boundary layer interaction will influence not only the haze pollution within the urban areas but also precipitation patterns, hydrological cycle and regional-scale weather patterns, for example, by disrupting and bifurcating storm fronts (Dou et al., 2015). However, the gigacity acting as one huge urban area has the potential to disrupt even larger-scale weather patterns, such as the Asian monsoon, thereby influencing global climate.
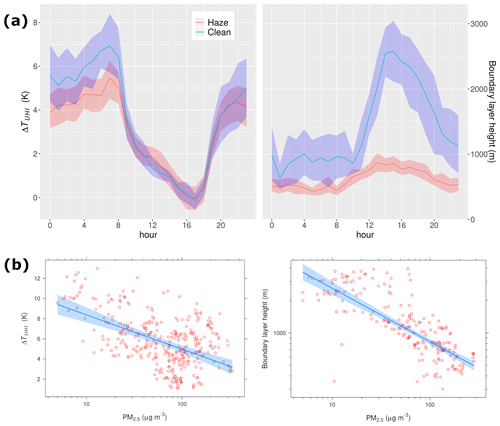
Figure 3(a) Mean diurnal cycle of the magnitude of an urban heat island (ΔTUHI) and boundary layer (BL) height during hazy (30 d) and clean conditions (24 d) between 6 February and 31 March 2018. The shaded areas are the 95 % confidence intervals of the fitted curve. ΔTUHI is calculated as a difference in air temperature between the Beijing University of Chemical Technology (BUCT) and a rural station in southeast of Beijing (Daxing; 39.654∘ N, 116.695∘ E; see Ma, et al., 2018). (b) Scatterplots of boundary layer height vs. PM2.5 concentration for daytime (14:00–18:00 LT – local time; right panel) and urban heat island magnitude (ΔTUHI) vs. PM2.5 concentration for nighttime (0:00–8:00 LT; left panel). The values where ΔTUHI is under 4 K, with low PM2.5 concentrations (< 40), have been filtered out since those low values of ΔTUHI are presumably caused by phenomena other than haze (e.g., cloudiness). The shaded areas indicate 95 % confidence intervals.
In a typical megacity suffering from atmospheric pollution, haze formation and planetary boundary layer (PBL) processes include a suite of feedback mechanisms and nonlinear interactions. The haze reduces the amount of solar radiation reaching the surface (Arnfield, 2003), which decreases the near-surface air temperature and, thereby, reduces the vertical turbulent mixing of air and, consequently, daytime BL height (Ding et al., 2017; Ma et al., 2020; Petäjä et al., 2016; Wang et al., 2020). During the winter 2018 in Beijing, we found that, compared with clean days, the reduced incoming solar radiation on the haze days decreased the BL height on average by 1660 m during daytime (Fig. 3a). The daytime BL height decreased by approximately 70 % when the PM2.5 concentration increased by a factor of 10 (Fig. 3b). At the same time, solar heat stored in urban built-up surfaces was reduced due to the attenuated incoming solar radiation on haze days, which weakened the nocturnal UHI by about 1.6 K (Fig. 3a), while the temporal evolution of UHI during daytime remained relatively unchanged. The average magnitude of nighttime UHI decreased by about 40 % when the PM2.5 concentration increased by a factor of 10 (Fig. 3b). In addition, the reduced nighttime UHI and heat emissions produced lower nocturnal BL during haze days (on average by 490 m; Fig. 3a). As a summary, in a typical megacity the feedbacks associated with the BL height and particulate pollution will force the emissions into a smaller and smaller volume, further enhancing the accumulation of pollutants, and the overall weakening of the UHI will decrease the urban heat dome flow, inhibiting the lateral ventilation of urban areas (Miao et al., 2015) on a regional scale.
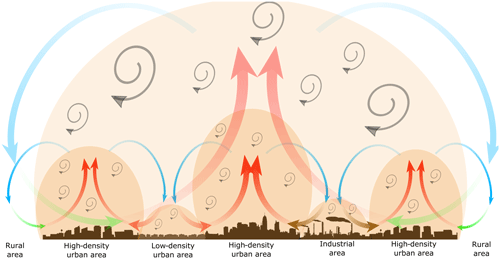
Figure 4A schematic figure showing a complex structure of an urban heat island (UHI) in the gigacity, where, within one large UHI, multiple individual UHI circulation patterns are formed between the lower- and higher-density urban areas with a lack of rural areas. Within the gigacity, the UHI circulation is not bringing cooler and cleaner air from rural areas but draws in already warm air from the lower-density urban areas and even highly polluted air from the industrial areas. Only at the sides of the gigacity is cooler and cleaner air drawn into the city, and it will not penetrate into the middle parts of the gigacity. If the temperature difference between the high-density urban areas in the middle and the lower-density urban areas is not sufficient, the UHI circulation might also be totally suppressed in the middle parts of the gigacity. The complex internal circulation can lead to high ambient temperatures and a high concentration of atmospheric pollutant levels inside the gigacity.
In typical urban areas, the maximum values of UHI are restricted by the UHI-induced circulation between the rural and urban areas, bringing cooler and cleaner air into the city. However, within the gigacity, multiple smaller-scale UHI circulations are formed, leading to almost closed internal circulation patterns relying on warm and polluted air (Fig. 4) as the influx. The UHI dynamics are highly dependent on the season, latitude, local climate, relative geographical location and the size and shape of the city (Han et al., 2020; Huang et al., 2020). Therefore, the details remain unclear. However, we can foresee that an enhanced frequency and intensity of extreme rainfall and flash floods within and downwind of the gigacity and a decreased amount of total cumulative rainfall (Mahmood et al., 2014; Zhang et al., 2019) are likely to occur. These changes are connected to the increased surface roughness and modified energy and water balances associated with the combination of UHI, high concentration of aerosols and higher fraction of constructed regions. Such consequences pose challenges to the air quality, water supply and agricultural production within the gigacity (Ding et al., 2017). Due to the nonlinear nature of these interactions, the consequences can be stronger, or even reversed, in the gigacity compared with an isolated megacity.
The atmospheric pollution at the gigacity area is a complex mixture of local and regional-scale phenomena (e.g., Harrison et al., 2021; Huang et al., 2020; Kulmala, 2015; Wu et al., 2020). On the one hand, we have emissions from the local emission hot spots within the gigacity (e.g., industrial sources) and local and regional-scale secondary aerosol formation (Huang et al., 2020). On the other hand, we have regional transport of pollutants within the gigacity and transport from outside the gigacity area (e.g., industry, biomass burning, etc.; Ding et al., 2017). Many recent studies (e.g., Wang et al., 2018) have demonstrated that the impact of anthropogenic processes, such as those related to air pollution, have not been included in the current weather forecast models. As a result, these models show notable biases in the prediction of free tropospheric air temperature in the gigacity region. In fact, in the gigacity region, anthropogenic aerosols (e.g., black carbon) could significantly influence the development of PBL via not only the reduction in surface solar radiation but also its dome effect by heating the upper PBL (Ding et al., 2016; Wang et al., 2018). In addition, aerosol–PBL feedback could also occur at the gigacity scale by amplifying the transboundary transport of haze, including secondary pollutants, between different megacity clusters, such as the Yangtze River Delta and the Beijing–Tianjin–Hebei area (Huang et al., 2020).
The Chinese government has started to tackle the air pollution with the Clean Air Act from 2013 onwards, which has already shown a substantial reduction in pollutants in eastern China (Ding et al., 2019; Vu et al., 2019). However, nonbalanced reductions in otherwise polluted regions could even lead to a negative effect as was demonstrated during the COVID-19 lockdown (Huang et al., 2021). Therefore, further research is needed in order to better understand the synergic effects of multi-pollutant emission reduction under the influence of regional-scale aerosol–PBL–weather interaction (Ding et al., 2017).
Even though the reduction in pollutants will lead also to increased boundary layer height (BLH), which will reduce the pollution further, the cleaner air will also allow more incoming solar radiation to reach urban surfaces, which will increase the UHI effect in the gigacity area. Already the individual megacities within the gigacity are suffering from extreme heat during the summertime (e.g., Nanjing). An increase in UHI and the lack of cooler air as an input flux from the diminishing rural areas could lead to unbearable urban summertime temperatures inside the gigacity. Therefore, a comprehensive research program would be needed in order to understand the interactions of the interlinked local and regional-scale phenomena, the associated feedbacks and the highly complex atmospheric chemical processes.
We need an integrated research program comprising all the scales from understanding the processes in the atmospheric pollution cocktail via local boundary layer dynamics and regional air quality to the global scale in order to understand the effect of the whole gigacity region on the local or regional air pollution and larger-scale weather patterns and climate. For example, anthropogenic effects (urban structures, air pollution and anthropogenic heat) have been shown to substantially modify the water balance on a neighborhood scale (Best and Grimmond, 2016; Grimmond and Oke, 1986; Kokkonen et al., 2018a, 2019) and on the scale of the whole city (Dou et al., 2015). Urban areas can also disrupt and bifurcate storm fronts (Dou et al., 2015), and because the gigacity is a huge source of anthropogenic heat, air pollution and a vast area of increased surface roughness, it has the potential to affect the Asian monsoon and, therefore, definitely regional and even global climate.
The Asian monsoon is very important for the whole gigacity region because disturbances in the Asian monsoon can affect the fresh water availability in the region and can also cause catastrophic flooding (Ding et al., 2015; Li et al., 2016). However, as the focus of the ongoing and past research has been on the effects of the global climate change on the Asian monsoon (Goodkin et al., 2019; Li et al., 2016; Seth et al., 2019; Zhang, 2015), we need to understand better how all these anthropogenic phenomena, e.g., the effects of urban structures, air pollution, anthropogenic heat and also anthropogenic climate change, together affect the very large scale of the gigacity and its downwind areas.
Currently, detailed studies within the gigacity are typically covering the different phenomena, such as weather forecast, urbanization or air quality, as separate issues. As mentioned above, in gigacity areas, human activities could influence the climate system all the way from the nanoscale to the global scale. The lack of comprehensive studies is partly due to the lack of openly available, comprehensive observation data. If suitable observations are lacking either high enough spatial coverage or some of the needed variables, one option is to use reanalysis data. However, such data are not available in a high enough resolution, and they do not describe urban areas, especially local-scale air pollution, in high enough accuracy for local-scale urban studies (Best and Grimmond, 2016; Fowler et al., 2007; Kokkonen et al., 2018b, 2019).
Therefore, we need comprehensive observations and high-resolution, fully coupled models to accurately describe the molecular-scale chemistry, the microphysics of aerosol–cloud interaction and the multi-scale processes of aerosol–PBL–weather feedback at the gigacity and even global scale. The spatial coverage of observations and the model resolution need to be high enough to characterize the complexity of emissions and chemistry, land-surface processes, and their interaction with the PBL meteorology well. We also need to understand aerosol physics and atmospheric chemistry in molecular and nanometer scales, as the majority of particle number and mass concentrations, as well as ozone, is caused by secondary processes taking place in the atmosphere (e.g., Kulmala et al., 2021). To understand such secondary processes, we need proper observations and process level models, including quantum chemistry (e.g., Kulmala et al., 2021). Since some of the processes might be unique to the gigacity area, and possibly still unknown, we need comprehensive observations and concurrent models that cover different regions of the gigacity (Kulmala 2015, 2018) in order to characterize different processes and their interactions and to force the models and validate the results.
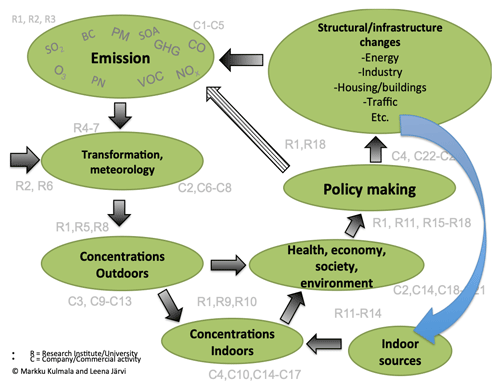
Figure 5Schematic figure for a holistic research and innovation program. Emissions include all potential emissions. The arrow from the left to the “transformation, meteorology” oval means different air masses. The arrows from the concentrations (indoors or outdoors) lead to the impact oval and then to policy making. There could be either direct decisions to cut emissions or indirect ones, by changing either the structure or infrastructure.
The different aspects of a comprehensive research and innovation program for the gigacity are presented in Fig. 5. The program should include all the potential emissions and explore pathways for their reduction. It should also include atmospheric processes, air composition and concentrations, atmospheric dynamics, as well as pollution levels outdoors and indoors. It is also crucial to include environmental, health, economic and societal impacts and decision-making processes. The decision-making will then feedback to the emissions and influence air quality indoors and outdoors.
The aims and main research questions of the program should include the following:
- a.
Quantifying emission patterns in a high spatiotemporal resolution and finding pathways to reduce emissions that are most harmful in the pollution cocktail
- b.
Understanding processes in the atmospheric pollution cocktail in order to reduce secondary pollution within the gigacity
- c.
Understanding the effect of PBL dynamics in enhancing haze episodes on the gigacity scale and, furthermore, finding out ways of avoiding them
- d.
Understanding the UHI and its dynamics and reducing the adverse impacts of both UHI and pollution on the gigacity scale
- e.
Understanding interactions and feedbacks between air pollution and weather
- f.
Understanding and quantifying interactions and feedbacks between air pollution and climate
- g.
Finding out and quantifying the contribution of the gigacity to the global climate
- h.
Finding out sustainable ways to solve the other grand challenges, such as sustainable water and food supply
- i.
Exploring feedbacks between biodiversity and air quality
- j.
Finding out interactions and feedbacks between air quality and pandemic
- k.
Finding out pathways for protecting biodiversity within the gigacity
- l.
Quantifying nonlinear interactions between the atmospheric composition, meteorology, city planning or land use, human adaption and behavior.
In addition, it is already foreseen that, as soon as we have comprehensive open data sets available from the gigacity environment, we will be able to pose questions that we not yet have identified and provide quantitative answers to the global grand challenges.
At the moment there are no other truly gigacity areas in the world where the cluster of individual megacities could be classified as one huge continuous urban area. However, there are already other possible areas of which we know that might struggle with similar problems as those in the Chinese gigacity in the future. Especially the Ganges basin, with a population of about 400 million people, is definitely already quite close to having the characteristics of the Chinese gigacity. In addition, western Europe (population 197 M) and the Boston–Washington (Bos-Wash) megalopolis area (population ∼ 50 M) in the northeastern USA are both still predicted to have an increasing population density in the future (Hoornweg and Pope, 2017). These areas could show a degree of connectivity between the cities, where the problems cannot be solved by one municipality or even one country acting alone. In the future, the north shore of the Gulf of Guinea will also be another potential gigacity area, especially around Lagos, which is projected to be the largest city in the world (∼ 100 million people) by the end of the century (Hoornweg and Pope, 2017).
The experiences gained from the Chinese gigacity could reveal a tipping point in which the whole area starts to act as one enormous urban area with interlinked problems instead of individual megacities. In addition, the critical areas of development and the number of observation stations needed to understand the changes induced could be identified and, therefore, used to tackle the upcoming problems. This increased knowledge gained from the Chinese gigacity can greatly benefit the other potential gigacity areas in the sustainable development of these regions in the future.
In order to have an integrated research program covering all the scales, we first need to expand the network of comprehensive research stations and provide the data freely to whomever might need it. We need enough measurement stations to capture the spatial variation in different phenomena and circulation of air within the individual megacities as well as within the whole gigacity and the surrounding regions.
To establish an integrated comprehensive research program is the key to go beyond the present, ongoing research by collecting and utilizing openly available big data from in situ measurements, satellites and models. Instead of research focusing on individual sites and phenomena, we will perform interlinked investigations, including all the interactions between different scales, feedbacks, meteorology, hydrology, air pollution, etc.
A framework is needed in which a multidisciplinary scientific approach has the required critical mass and is strongly connected to dynamic policy making. Dynamic proactive policy making requires a lot of reliable data through a chain of harmonized network of observations. This is true in the case of fighting against global grand challenges, including climate change, air quality and viruses like COVID-19. The following items are proper actions to proceed with, according to the roadmap:
- i.
From unknown to data. It is crucial to collect comprehensive data. These include environmental variables, health and socioeconomic data as well as behavioral patterns and movement of people. We need to continuously harvest data (Kulmala, 2018), even for the research questions of which we are not yet aware but may or will emerge in the future. With big, open data we can, in future, answer such questions that do not exist yet, but it will be possible only if the needed data exist at that moment.
- ii.
Platform and analytics. We need a platform for integrative analytics. We have to focus on reliable ways to collect, share, analyze and integrate data. We have to apply artificial intelligence and take full advantage of modern telecommunication systems, such as 5G, to make rapid data synthesis beyond the current state of the art.
- iii.
Innovative cooperation. We need innovative and open cooperation on local, regional, national and international levels. An open discussion and different views are needed to understand our own aspects better. Globally, we need to strengthen multilateral cooperation. While a bigger player may have more influence, a smaller may have more resilience.
Finally, the decision-makers should take the responsibility of steering the process towards a sustainable gigacity with the steps identified above. A comprehensive, long-term research and innovation program supported by comprehensive data platform in environmental, societal, health, economic and policy data would support the sustainable urban development on the gigacity level and help society to respond to the urgent challenges (e.g., the COVID-19 pandemic). Therefore, it is crucial to promote this kind of program and start it as soon as reasonable. In the case of success, the gigacity has the potential to be a visible example of a sustainable future society.
The codes used to analyze and visualize the data in this publication are available from the authors upon request.
The data sets in this paper are available from the authors upon request.
MK and AD conceived the paper. TVK performed the data analysis. TVK, AD, MK, VMK and TP interpreted the data. MK, JP, AD, VMK, TVK, TP and SP contributed to specific grand challenges. MK, SP and TP provided the upscaling plan. All the authors contributed to writing the paper.
The authors declare that they have no conflict of interest.
Leena Järvi and Pauli Paasonen are acknowledged.
This research has been supported by the Academy of Finland (grant nos. 272041, 316114, 315203 and 307537), the European Research Council, H2020 European Research Council (ATM-GTP; grant no. 742206), Business Finland (Megasense project grant), the European Commission, Horizon 2020 (ERA-PLANET; grant no. 689443), the National Key Research and Development Program of China (grant no. 2016YFC0200500), and the National Natural Science Foundation of China (grant nos. 91544231 and 41725020).
Open-access funding was provided by the Helsinki University Library.
This paper was edited by Ken Carslaw and reviewed by Roy M. Harrison and one anonymous referee.
Arnfield, A. J.: Two decades of urban climate research: a review of turbulence, exchanges of energy and water, and the urban heat island, Int. J. Climatol., 23, 1–26, https://doi.org/10.1002/joc.859, 2003.
Baklanov, A., Grimmond, C. S. B., Carlson, D., Terblanche, D., Tang, X., Bouchet, V., Lee, B., Langendijk, G., Kolli, R. K., and Hovsepyan, A.: From urban meteorology, climate and environment research to integrated city services, Urban Climate, 23, 330–341, https://doi.org/10.1016/j.uclim.2017.05.004, 2018.
Best, M. J. and Grimmond, C. S. B: Modeling the partitioning of turbulent fluxes at urban sites with varying vegetation cover, J. Hydrometeorol., 17, 2537–2553, https://doi.org/10.1175/JHM-D-15-0126.1, 2016.
Burnett, R., Chen, H., Szyszkowicz, M., Fann, N., Hubbell, B., Pope, C. A., Apte, J. S., Brauer, M., Cohen, A., Weichenthal, S., Coggins, J., Di, Q., Brunekreef, B., Frostad, J., Lim, S. S., Kan, H. D., Walker, K. D., Thurston, G. D., Hayes, R. B., Lim, C. C., Turner, M. C., Jerrett, M., Krewski, D., Gapstur, S. M., Diver, W. R., Ostro, B., Goldberg, D., Crouse, D. L., Martin, R. V., Peters, P., Pinault, L., Tjepkema, M., Donkelaar, A., Villeneuve, P. J., Miller, A. B., Yin, P., Zhou, M. G., Wang, L. J., Janssen, N. A. H., Marra, M., Atkinson, R. W., Tsang, H., Thach, Q., Cannon, J. B., Allen, R. T., Hart, J. E., Laden, F., Cesaroni, G., Forastiere, F., Weinmayr, G., Jaensch, A., Nagel, G., Concin, H., and Spadaro, J. V.: Global estimates of mortality associated with long-term exposure to outdoor fine particulate matter, P. Natl. Acad. Sci. USA, 115, 9592–9597, https://doi.org/10.1073/pnas.1803222115, 2018.
CIESIN – Center for International Earth Science Information Network – Columbia University: Gridded Population of the World, Version 4 (GPWv4): Population Density, Revision 11, NASA Socioeconomic Data and Applications Center (SEDAC), Palisades, NY, https://doi.org/10.7927/H49C6VHW, 2018.
Ding, A., Huang, X., and Fu, C.: Air pollution and Weather Interaction in East Asia, Oxford Research Encyclopedias: Environmental Science, https://doi.org/10.1093/acrefore/9780199389414.013.536, 2017.
Ding, A., Huang, X., Nie, W., Chi, X., Xu, Z., Zheng, L., Xu, Z., Xie, Y., Qi, X., Shen, Y., Sun, P., Wang, J., Wang, L., Sun, J., Yang, X.-Q., Qin, W., Zhang, X., Cheng, W., Liu, W., Pan, L., and Fu, C.: Significant reduction of PM2.5 in eastern China due to regional-scale emission control: evidence from SORPES in 2011–2018, Atmos. Chem. Phys., 19, 11791–11801, https://doi.org/10.5194/acp-19-11791-2019, 2019.
Ding, A. J., Fu, C. B., Yang, X. Q., Sun, J. N., Zheng, L. F., Xie, Y. N., Herrmann, E., Nie, W., Petäjä, T., Kerminen, V.-M., and Kulmala, M.: Ozone and fine particle in the western Yangtze River Delta: an overview of 1 yr data at the SORPES station, Atmos. Chem. Phys., 13, 5813–5830, https://doi.org/10.5194/acp-13-5813-2013, 2013.
Ding, A. J., Huang, X., Nie, W., Sun, J. N., Kerminen, V.-M., Petäjä, T., Su, H., Cheng, Y. F., Yang, X.-Q., Wang, M. H., Chi, X. G., Wang, J. P., Virkkula, A., Guo, W. D., Yuan, J., Wang, S. Y., Zhang, R. J., Wu, Y. F., Song, Y., Zhu, T., Zilitinkevich, S., Kulmala, M., and Fu, C. B.: Enhanced haze pollution by black carbon in megacities in China, Geophys. Res. Lett., 43, 2873–2879, https://doi.org/10.1002/2016GL067745, 2016.
Ding, Y., Liu, Y., Song, Y., and Zhang, J.: From MONEX to the global monsoon: A review of monsoon system research, Adv. Atmos. Sci., 32, 10–31, https://doi.org/10.1007/s00376-014-0008-7, 2015.
Dou, J., Wang, Y., Bornstein, R., and Miao, S.: Observed spatial characteristics of Beijing urban climate impacts on summer thunderstorms, J. Appl. Meteorol. Clim., 54, 94–105, https://doi.org/10.1175/JAMC-D-13-0355.1, 2015.
Fang, J., Yu, G. R., Liu, L. L., Hu, S. J., and Chapin, F. S.: Climate change, human impacts, and carbon sequestration in China, P. Natl. Acad. Sci. USA, 115, 4015–4020, https://doi.org/10.1073/pnas.1700304115, 2018.
Figueres, C., Schellnhuber, H. J., Whiteman, G., Rockstrom, J., Hobley, A., and Rahmstorf, S.: Three years to safeguard our climate, Nature, 546, 593–595, https://doi.org/10.1038/546593a, 2017.
Fowler, H. J., Blenkinsop, S., and Tebaldi, C.: Linking climate change modelling to impacts studies: recent advances in downscaling techniques for hydrological modelling, Int. J. Climatol., 27, 1547–1578, https://doi.org/10.1002/joc.1556, 2007.
Goodkin, N. F., Bolton, A., Hughen, K. A., Karnauskas, K. B., Griffin S., Phan, K. H., Vo, S. T., Ong M. R., and Druffel, E. R. M.: East Asian monsoon variability sine the sixteenth century, Geophys. Res. Lett., 46, 4790–4798, https://doi.org/10.1029/2019GL081939, 2019.
Grimmond, C. S. B. and Oke, T. R.: Urban water balance: 2. Results from a suburb of Vancouver, British Columbia, Water Resour. Res., 22, 1404–1412, https://doi.org/10.1029/WR022i010p01404, 1986.
Han, W., Li, Z., Wu, F., Zhang, Y., Guo, J., Su, T., Cribb, M., Fan, J., Chen, T., Wei, J., and Lee, S.-S.: The mechanisms and seasonal differences of the impact of aerosols on daytime surface urban heat island effect, Atmos. Chem. Phys., 20, 6479–6493, https://doi.org/10.5194/acp-20-6479-2020, 2020.
Harrison, R. M., Vu, T. V., Jafar, H., and Shi, Z.: More mileage in reducing urban air pollution from road traffic, Environ. Int., 149, 106329, https://doi.org/10.1016/j.envint.2020.106329, 2021.
Hoornweg, D. and Pope, K.: Population predictions for the world's largest cities in the 21st century, Environ. Urban., 29, 195–216, https://doi.org/10.1177/0956247816663557, 2017.
Huang, R., Zhang, Y. L., Bozzetti, C., Ho, K. F., Cao, J. J., Han, Y. M., Daellenbach, K. R., Slowik, J. G., Platt, S. M., Canonaco, F., Zotter, P., Wolf, R., Pieber, S. M., Bruns, E. A., Crippa, M., Ciarelli, G., Piazzalunga, A., Schwikowski, M., Abbaszade, G., Schnelle-Kreis, J., Zimmermann, R., An, Z. S., Szidat, S., Baltensperger, U., El Haddad, I., and Prevot, A. S. H.: High secondary aerosol contribution to particulate pollution during haze events in China, Nature, 514, 218–222, https://doi.org/10.1038/nature13774, 2014.
Huang, X., Ding, A., Wang, Z., Ding, K., Gao, J., Chai, F., and Fu, C.: Amplified transboundary transport of haze by aerosol-boundary layer interaction in China, Nat. Geosci., 13, 428–434, https://doi.org/10.1038/s41561-020-0583-4, 2020.
Huang, X., Ding, A., Gao, J., Zheng, B., Zhou, D., Qi, X., Tang, R., Wang, J., Ren, C., Nie, W., Chi, X., Xu, Z., Chen, L., Li, Y., Che, F., Pang, N., Wang, H., Tong, D., Qin, W., Cheng, W., Liu, W., Fu, Q., Liu, B., Chai, F., Davis, S. J., Zhang, Q., and He, K.: Enhanced secondary pollution offset reduction of primary emissions during COVID-19 lockdown in China, Natl. Sci. Rev., 8, nwaa137, https://doi.org/10.1093/nsr/nwaa137, 2021.
Kokkonen, T. V., Grimmond, C. S. B., Christen, A., Oke, T. R., and Järvi, L.: Changes to the water balance over a century of urban development in two neighborhoods: Vancouver, Canada, Water Resour. Res., 54, 6625–6642, https://doi.org/10.1029/2017WR022445, 2018a.
Kokkonen, T. V., Grimmond, C. S. B., Räty, O., Ward, H. C., Christen, A., Oke, T. R., Kotthaus, S., and Järvi, L.: Sensitivity of Surface Urban Energy and Water Balance Scheme (SUEWS) to downscaling of reanalysis forcing data, Urban Climate, 23, 36–52, https://doi.org/10.1016/j.uclim.2017.05.001, 2018b.
Kokkonen, T. V., Grimmond, S., Murto, S., Liu, H., Sundström, A.-M., and Järvi, L.: Simulation of the radiative effect of haze on the urban hydrological cycle using reanalysis data in Beijing, Atmos. Chem. Phys., 19, 7001–7017, https://doi.org/10.5194/acp-19-7001-2019, 2019.
Kulmala, M.: Atmospheric chemistry: China's choking cocktail, Nature, 526, 497–499, https://doi.org/10.1038/526497a, 2015.
Kulmala, M.: Build a global Earth observatory, Nature, 553, 21–23, https://doi.org/10.1038/d41586-017-08967-y, 2018.
Kulmala, M., Dada, L., Daellenbach, K. R., Yan, C., Stolzenburg, D., Kontkanen, J., Ezhova, E., Hakala, S., Tuovinen, S., Kokkonen, T. V., Kurppa, M., Cai, R., Zhou, Y., Yin, R., Baalbaki, R., Chan, T., Chu, B., Deng, C., Fu, Y., Ge, M., He, H., Heikkinen, L., Junninen, H., Liu, Y., Lu, Y., Nie, W., Rusanen, A., Vakkari, V., Wang, Y., Yang, G., Yao, L., Zheng, J., Kujansuu, J., Kangasluoma, J., Petäjä, T., Paasonen, P., Järvi, L., Worsnop, D., Ding, A., Liu, Y., Wang, L., Jiang, J., Bianchi, F., and Kerminen, V.-M.: Is reducing new particle formation a plausible solution to mitigate particulate air pollution in Beijing and other Chinese megacities?, Faraday Discuss., 226, 334–347, https://doi.org/10.1039/D0FD00078G, 2021.
Li, Z., Lau, W. K.-M., Ramanathan, V., Wu, G., Ding, Y., Manoj, M. G., Liu, J., Qian, Y., Li, J., Zhou, T., Fan, J., Rosenfeld, D., Ming, Y., Wang, Y., Huang, J., Wang, B., Xu, X., Lee, S.-S., Cribb, M., Zhang, F., Yang, X., Zhao, C., Takemura, T., Wang, K., Xia, X., Yin, Y., Zhang, H., Guo, J., Zhai, P. M., Sugimoto, N., Babu, S. S., and Brasseur, G. P.: Aerosol and monsoon climate interactions over Asia, Rev. Geophys., 54, 866–929, https://doi.org/10.1002/2015RG000500, 2016.
Liu, Y. and Wang, T.: Worsening urban ozone pollution in China from 2013 to 2017 – Part 2: The effects of emission changes and implications for multi-pollutant control, Atmos. Chem. Phys., 20, 6323–6337, https://doi.org/10.5194/acp-20-6323-2020, 2020.
Liu, Z., He, C., and Wu, J.: The relationship between habitat loss and fragmentation during urbanization: an empirical evaluation from 16 World cities, PLOS One, 11, e0154613, https://doi.org/10.1371/journal.pone.0154613, 2016.
Lu, X., Lin, C. Q., Li, W. K., Chen, Y., Huang, Y. Q., Fung, J. C. H., and Lau, A. K. H.: Analysis of the adverse health effects of PM2.5 from 2001 to 2017 in China and the role of urbanization in aggravating the health burden, Sci. Total Environ., 652, 683–695, https://doi.org/10.1016/j.scitotenv.2018.10.140, 2019.
Ma, J. Z., Chu, B. W., Liu, J., Liu, Y. C., Zhang, H. X., and He, H.: NOx promotion of SO2 conversion to sulfate: An important mechanism for the occurrence of heavy haze during winter in Beijing, Environ. Pollut., 233, 662–669, https://doi.org/10.1016/j.envpol.2017.10.103, 2018.
Ma, Y. J., Ye, J. H., Xin, J. Y., Zhang, W. Y., de Arellano, J. V. G., Wang, S. G., Zhao, D. D., Dai, L. D., Ma, Y. X., Wu, X. Y., Xia, X. G., Tang, G. Q., Wang, Y. S., Shen, P. K., Lei, Y. L., and Martin, S. T.: The Stove, Dome, and Umbrella Effects of Atmospheric Aerosol on the Development of the Planetary Boundary Layer in Hazy Regions, Geophys. Res. Lett., 47, e2020GL087373, https://doi.org/10.1029/2020GL087373, 2020.
Mahmood, R., Pielke, R. A., Hubbard, K. G., Niyogi, D., Dirmeyer, P. A., McAlpine, C., Carleton, A. M., Hale, R., Gameda, S., Beltran-Przekurat, A., Baker, B., McNider, R., Legates, D. R., Shepherd, M., Du, J. Y., Blanken, P. D., Frauenfeld, O. W., Nair, U. S., and Fall, S.: Land cover changes and their biogeophysical effects on climate, Int. J. Climatol., 34, 929–953, https://doi.org/10.1002/joc.3736, 2014.
Miao, Y., Liu, S. H., Zheng, Y. J., Wang, S., Chen, B. C., Zheng, H., and Zhao, J. C.: Numerical study of the effects of local atmospheric circulations on a pollution event over Beijing–Tianjin–Hebei, China, J. Environ. Sci., 30, 9–20, https://doi.org/10.1016/j.jes.2014.08.025, 2015.
Parrish, D. D. and Zhu, T.: Clean air for megacities, Science, 326, 674–675, https://doi.org/10.1126/science.1176064, 2009.
Petäjä, T., Järvi, L., Kerminen, V. M., Ding, A. J., Sun, J. N., Nie, W., Kujansuu, J., Virkkula, A., Yang, X. Q., Fu, C. B., Zilitinkevich, S., and Kulmala, M.: Enhanced air pollution via aerosol-boundary layer feedback in China, Sci. Rep., 6, 18998, https://doi.org/10.1038/srep18998, 2016.
Ramaswami, A., Russell, A. G., Culligan, P. J., Sharma, K. R., and Kumar, E.: Meta-principles for developing smart, sustainable, and healthy cities, Science, 352, 940–943, https://doi.org/10.1126/science.aaf7160, 2016.
Seth, A., Giannini, A., Rojas, M., Rauscher, S. A., Bordoni, S., Singh, D., and Camargo S. J.: Monsoon responses to climate changes – connecting part, present and future, Current Climate Change Reports, 5, 63–79, https://doi.org/10.1007/s40641-019-00125-y, 2019.
Tian, H., Liu, Y. H., Li, Y. D., Wu, C. H., Chen, B., Kraemer, M. U. G., Li, B. Y., Cai, J., Xu, B., Yang, Q. Q., Wang, B., Yang, P., Cui, Y. J., Song, Y. M., Zheng, P., Wang, Q. Y., Bjornstad, O. N., Yang, R. F., Grenfell, B. T., Pybus, O. G., and Dye, C.: An investigation of transmission control measures during the first 50 days of the COVID-19 epidemic in China, Science, 368, 638–642, https://doi.org/10.1126/science.abb6105, 2020.
Vu, T. V., Shi, Z., Cheng, J., Zhang, Q., He, K., Wang, S., and Harrison, R. M.: Assessing the impact of clean air action on air quality trends in Beijing using a machine learning technique, Atmos. Chem. Phys., 19, 11303–11314, https://doi.org/10.5194/acp-19-11303-2019, 2019.
Wang, Y., Yu, M., Wang, Y., Tang, G., Song, T., Zhou, P., Liu, Z., Hu, B., Ji, D., Wang, L., Zhu, X., Yan, C., Ehn, M., Gao, W., Pan, Y., Xin, J., Sun, Y., Kerminen, V.-M., Kulmala, M., and Petäjä, T.: Rapid formation of intense haze episodes via aerosol–boundary layer feedback in Beijing, Atmos. Chem. Phys., 20, 45–53, https://doi.org/10.5194/acp-20-45-2020, 2020.
Wang, Z., Huang, X., and Ding, A.: Dome effect of black carbon and its key influencing factors: a one-dimensional modelling study, Atmos. Chem. Phys., 18, 2821–2834, https://doi.org/10.5194/acp-18-2821-2018, 2018.
Wu, X., Chen, C., Vu, T. V., Liu, D., Baldo, C., Shen, X., Zhang, Q., Cen, K., Zheng, M., He, K., Shi, Z., and Harrison R. M.: Source apportionment of fine organic carbon (OC) using receptor modelling at a rural site of Beijing: Insight into seasonal and diurnal variation of source contributions, Environ. Pollut., 266, 115078, https://doi.org/10.1016/j.envpol.2020.115078, 2020.
Yang, J., Siri, J. G., Remais, J. V., Cheng, Q., Zhang, H., Chan, K. K. Y., Sun, Z., Zhao, Y. Y., Cong, N., Li, X. Y., Zhang, W., Bai, Y. Q., Bi, J., Cai, W. J., Chan, E. Y. Y., Chen, W. Q., Fan, W. C., Fu, H., He, J. Q., Huang, H., Ji, J. S., Jia, P., Jiang, X. P., Kwan, M. P., Li, T. H., Li, X. G., Liang, S., Liang, X. F., Liang, L., Liu, Q. Y., Lu, Y. M., Luo, Y., Ma, X. L., Schwartlander, B., Shen, Z. Y., Shi, P. J., Su, J., Wu, T. G., Yang, C. H., Yin, Y. Y., Zhang, Q., Zhang, Y. P., Zhang, Y., Xu, B., and Gong, P.: The Tsinghua–Lancet Commission on Healthy Cities in China: unlocking the power of cities for a healthy China, Lancet, 391, 2140–2184, https://doi.org/10.1016/S0140-6736(18)30486-0, 2018.
Zhang, H., Wu, C. H., Chen, W. J., and Huang, G. R.: Effect of urban expansion on summer rainfall in the Pearl River Delta, South China, J. Hydrol., 568, 747–757, https://doi.org/10.1016/j.jhydrol.2018.11.036, 2019.
Zhang, Q., Jiang, X. J., Tong, D., Davis, S. J., Zhao, H. Y., Geng, G. N., Feng, T., Zheng, B., Lu, Z. F., Streets, D. G., Ni, R. J., Brauer, M., van Donkelaar, A., Martin, R. V., Huo, H., Liu, Z., Pan, D., Kan, H. D., Yan, Y. Y., Lin, J. T., He, K. B., and Guan, D. B.: Transboundary health impacts of transported global air pollution and international trade, Nature, 543, 705–709, https://doi.org/10.1038/nature21712, 2017.
Zhang, R.-H.: Natural and human-induced changes in summer climate over the East Asian monsoon region in the last half century: A review, Advances in Climate Change Research, 6, 131–140, https://doi.org/10.1016/j.accre.2015.09.009, 2015.
Zhao, S., Zhou, D. C., Zhu, C., Sun, Y., Wu, W. J., and Liu, S. G.: Spatial and temporal dimensions of urban expansion in China, Environ. Sci. Technol., 49, 9600–9609, https://doi.org/10.1021/acs.est.5b00065, 2015.
- Abstract
- Grand challenges and urban development
- Challenges specific for the gigacities
- Feedback mechanisms in the gigacity
- Future research needs in the gigacity
- Other potential gigacity areas
- Required actions and the roadmap
- Code availability
- Data availability
- Author contributions
- Competing interests
- Acknowledgements
- Financial support
- Review statement
- References
- Abstract
- Grand challenges and urban development
- Challenges specific for the gigacities
- Feedback mechanisms in the gigacity
- Future research needs in the gigacity
- Other potential gigacity areas
- Required actions and the roadmap
- Code availability
- Data availability
- Author contributions
- Competing interests
- Acknowledgements
- Financial support
- Review statement
- References