the Creative Commons Attribution 4.0 License.
the Creative Commons Attribution 4.0 License.
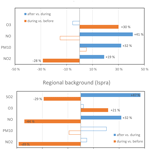
Impacts of the COVID-19 lockdown on air pollution at regional and urban background sites in northern Italy
Jean-Philippe Putaud
Luca Pozzoli
Enrico Pisoni
Sebastiao Martins Dos Santos
Friedrich Lagler
Guido Lanzani
Umberto Dal Santo
Augustin Colette
The COVID-19 lockdown measures gradually implemented in Lombardy (northern Italy) from 23 February 2020 led to a downturn in several economic sectors with possible impacts on air quality. Several communications claimed in the first weeks of March 2020 that the mitigation in air pollution observed at that time was actually related to these lockdown measures without considering that seasonal variations in emissions and meteorology also influence air quality. To determine the specific impact of lockdown measures on air quality in northern Italy, we compared observations from the European Commission Atmospheric Observatory of Ispra (regional background) and from the regional environmental protection agency (ARPA) air monitoring stations in the Milan conurbation (urban background) with expected values for these observations using two different approaches. On the one hand, intensive aerosol variables determined from specific aerosol characterisation observations performed in Ispra were compared to their 3-year averages. On the other hand, ground-level measured concentrations of atmospheric pollutants (NO2, PM10, O3, NO, SO2) were compared to expected concentrations derived from the Copernicus Atmosphere Monitoring Service Regional (CAMS) ensemble model forecasts, which did not account for lockdown measures. From these comparisons, we show that NO2 concentrations decreased as a consequence of the lockdown by −30 % and −40 % on average at the urban and regional background sites, respectively. Unlike NO2, PM10 concentrations were not significantly affected by lockdown measures. This could be due to any decreases in PM10 (and PM10 precursors) emissions from traffic being compensated for by increases in emissions from domestic heating and/or from changes in the secondary aerosol formation regime resulting from the lockdown measures. The implementation of the lockdown measures also led to an increase in the highest O3 concentrations at both the urban and regional background sites resulting from reduced titration of O3 by NO. The relaxation of the lockdown measures beginning in May resulted in close-to-expected NO2 concentrations in the urban background and to significant increases in PM10 in comparison to expected concentrations at both regional and urban background sites.
- Article
(3685 KB) -
Supplement
(1401 KB) - BibTeX
- EndNote
The COVID-19 pandemic is an epidemic of the coronavirus disease 2019 (COVID-19), of which the outbreak was first identified in Wuhan, China, in late December 2019. The World Health Organization declared COVID-19 a pandemic on 11 March 2020. The first case of COVID-19 in northern Italy was detected on 20 February 2020 in Codogno, about 60 km south-east of Milan (Fig. 1). To reduce the virus spreading, the Italian government quickly adopted a series of measures, such as the quarantine for 10 municipalities, the cancellation of all main public events, and the closure of schools and universities in northern Italy (DL, 23 February 2020a). The lockdown started in all of Italy on 9 March 2020 (DPCM, 8 March 2020a). All commercial and retail activities were closed on 11 March, except for grocery shops and pharmacies (DPMCM, 11 March 2020b), and it was forbidden to move outside the place of residence, except for health issues or work. Further lockdown measures were decreed on 22 March 2020 (DPCM, 22 March 2020c), including the suspension of all non-essential industrial production activities. The lockdown lasted until 4 May 2020 (DPCM, 26 April 2020d), when a gradual relaxation of the measures was decided by the government. The reopening of manufacturing industries and construction sites was allowed, but schools and universities as well as some commercial activities such as restaurants remained closed. Movements from a region to another were still forbidden, but moving short distances to work and to visit relatives was possible. From 18 May 2020, most commercial businesses could reopen, and free movement was granted within regional borders (DL, 16 May 2020b). This lockdown provided a unique opportunity to determine how such dramatic measures can eventually influence air quality. This is the focus of this paper.
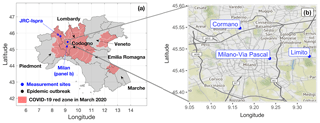
Figure 1Northern Italy areas impacted by COVID-19 at the beginning of March 2020 (red zones) and location of the air pollution measurement sites in Ispra and Milan considered in this study. (b) © OpenStreetMap contributors 2020. Distributed under a Creative Commons BY-SA License.
Lombardy, Piedmont and Emilia-Romagna in northern Italy produce roughly 50 % of the national gross domestic product (GDP), with Lombardy alone producing 22 % of the national GDP (data from ISTAT, 2020). This economic dynamism (mainly linked to industrial production and service-related activities) is associated with significant pollutant emissions, which together with unfavourable conditions for pollution dispersion (due to low wind speeds and particular orography) cause high pollution levels, leading to exceedances of the EU standards for nitrogen dioxide (NO2), particulate matter (PM10) and ozone (O3) in northern Italy (European Environment Agency, 2019). In this area, the impact of the lockdown on economic activities was quite important, as illustrated by data relative to the production of electricity and energy for heating and to transport-related activities (ARPA Lombardia, 2020a). Compared to 2019, the Italian thermal electricity production (Fig. 2) fell in March (−18 %), April (−24 %) and May 2020 (−16 %). The consumption of natural gas by the industrial sector as reported by the Italian natural gas provider (https://www.snam.it, last access: 9 October 2020) also fell by roughly −30 % at the end of March in comparison to the beginning of March 2020.
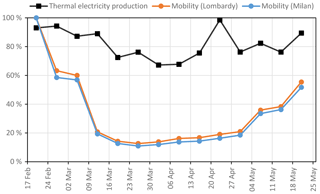
Figure 2Variations in activities resulting from lockdown measures (2020). Percentages are calculated in comparison with 2019 data for thermal energy production (source: https://www.terna.it, last access: 9 October 2020) and in comparison with data from the third week of February 2020 for mobility data (source: https://www.apple.com/covid19/mobility, last access: 9 October 2020).
Regarding transport, the Monitoring of Polluting Vehicles project (MOVE-IN) managed by the Lombardy region provided data on the traffic changes derived from its monitoring of “vehicle km” (the sum of kilometres travelled by all vehicles in the area) driven by light-duty vehicles and passenger cars (for a small number of vehicles compared to the full fleet circulating in the region though). MOVE-IN data show that the number of vehicle km driven by light-duty vehicles remained quite constant till 9 March 2020, then dropped by −75 % to reach a minimum between 16 March and 13 April 2020 before returning to “usual” (i.e. as before the lockdown period) values after 4 May 2020 (ARPA Lombardia, 2020a). For private cars, the number of vehicle km driven also decreased by roughly −70 % between the beginning and the end of March and started increasing again after 4 May but with a slower recovery than for light-duty vehicles. The number of requests for driving directions (https://www.apple.com/covid19/mobility, last access: 9 October 2020) showed similar variations (Fig. 2).
Numerous early communications based on preliminary measurement data analyses associated observed improvements in air quality with the lockdown measures taken to contain the spread of the COVID-19 epidemic. In Brazil, the lockdown in São Paulo was followed by drastic reductions in NO (up to −77 %) and NO2 (up to −54 %) and by an increase in O3 (approximately +30 %) compared to the previous 5-year means for the same period (Nakada et al., 2020). In the Yangtze River Delta region (China), Li et al. (2020) showed that concentrations of PM2.5, NO2 and SO2 decreased by 32 %, 45 % and 20 % during the first lockdown phase and by 33 %, 27 % and 7 % during the second lockdown phase, compared with the 2017–2019 average for the same period. O3 also increased in that region. Across Europe, Grange et al. (2021) estimated that NO2 and O3 concentrations at urban background sites were 32 % lower and 21 % higher than expected, respectively, when maximum mobility restrictions were in place. A clear decrease in NO2 concentrations in Barcelona and Madrid (Spain) during the lockdown was also described by Baldasano (2020). In France, the analysis by INERIS (Institut national de l'environnement industriel et des risques) compared air pollution forecast data (calculated without incorporating changes in emissions due to lockdown measures) with adjusted simulations performed a posteriori by assimilating observation data influenced by the lockdown measures. They estimated that NO2 concentrations were on average approximately 50 % lower than expected in France's largest cities (INERIS, 2020).
Regarding Italy, maps of NO2 surface concentrations estimated from satellite data (e.g. Sentinel-5p) were published by several websites and media showing large reductions in NO2 concentrations over northern Italy in March 2020 as compared to the previous months and to March 2019 (e.g. Copernicus Atmosphere Monitoring Service headlines published on 17 and 26 of March 2020; CAMS, 2020a, b). Observations and models were also combined in the analysis from the German Aerospace Center (DLR) which estimated a decrease of about 40 % in the total column-integrated NO2 tropospheric concentrations over norther Italy due to the lockdown measures using Sentinel-5p data. They also estimated reductions in ground level NO2 concentrations of about −20 µg m−3 (−45 %) by comparing ground-based observations from 25 stations in Lombardy to a model simulation with pre-lockdown emission levels (German Aerospace Center, 5 May 2020). In situ observations also showed reduced ground level NO2 concentrations as lockdown measures were implemented. The environmental protection agency ARPA Lombardia showed that March 2020 NO2 concentrations were below the standard deviation calculated from previous years, indicating a possible signal of reduced emissions from traffic and economic sectors (ARPA Lombardia, 2020a). The European Environment Agency (EEA) developed a viewer that tracks NO2 and particulate matter (PM10 and PM2.5) weekly average concentrations (https://www.eea.europa.eu/themes/air/air-quality-and-covid19, last access: 9 October 2020). It shows that NO2 concentrations in Milan were at least 24 % lower after the lockdown implementation than during previous weeks and 21 % lower compared to the same period in 2019. Similar trends were found in other cities of northern Italy and European countries where strong measures were taken to contain the epidemic. In contrast, no consistent effect of the lockdown measures on particulate matter (PM2.5 and PM10) could be observed in the main European cities (European Environment Agency, 2020).
Air pollution did decline in northern Italy from February to May in 2020 as it does every year, mainly due to seasonal variations in emissions and weather conditions. The strength of certain sources does indeed change during the course of the year, like, for example, domestic heating, while weather conditions influence pollution concentration in diverse ways: advection and dispersion of pollutants resulting from horizontal winds; dilution of pollutants throughout the mixed boundary layer resulting from convection; and pollutant lifetimes resulting from photochemical reactions (sun radiation), wet removal (clouds and rain), etc. It is therefore not straightforward to disentangle the effects of changing emissions due to lockdown measure implementation from those of seasonal changes in emissions and variability in meteorological conditions between different seasons and different years. In the present study we determine how much of the changes in air pollution observed during the lockdown period in northern Italy were actually due to lockdown measures, independently from expected variations in pollutants' emissions, lifetime and dispersion. Our results are based on comparisons between air pollution observation data from Ispra (regional background site) and the Milan conurbation (urban background sites) with CAMS regional ensemble (hereafter ENSEMBLE) model forecast data for the same sites. To help understand the effect of the lockdown measures in the regional background area, we also use 4 years of specific aerosol measurements from Ispra.
Model and observation air pollution data from four sites located in Lombardy covering the time periods 17 February–24 May 2019 and 2020 were collected and analysed. We selected three sites located in the Milan conurbation as representative of the Milan urban background and the site of Ispra as representative of the regional background of the upper Po Valley (Fig. 1). Ground level concentrations of NO, NO2, SO2, O3 and PM10 as measured in situ at the monitoring stations and as calculated by the ENSEMBLE model forecast were considered. Particle number size distribution and aerosol light absorption Ångström exponent data from Ispra for the 2017–2020 period were also utilised.
2.1 Site description
The European Commission Atmospheric Observatory (ECAtmO) has been operated in Ispra (45.815∘ N, 8.636∘ E; 209 m a.s.l.) since November 1985. It has contributed to the CLRTAP-EMEP (co-operative programme for monitoring and evaluation of the long-range transmission of air pollutants in Europe under the Convention on Long-range Transboundary Air Pollution) and WMO-GAW (World Meteorological Organization – Global Atmosphere Watch) air pollution measurement programmes for several decades and to the European Research Infrastructures ICOS (Integrated Carbon Observation System) and ACTRIS (Research Infrastructure for the observation of Aerosol, Clouds and Trace Gases) for several years. ECAtmO is located on the north-western edge of the Po Valley, 20–60 km away from major pollution point sources but still in a densely populated area (ca. 500 km−2) with significant economic activity (GDP per capita = EUR 29 000; EUROSTAT, 2017). Wood burning for domestic heating is also an important source of particulate matter during the cold period of the year (Gilardoni et al., 2011). Past measurements of HCHO NO2 ratios compared to the threshold values proposed by Tonnensen and Dennis (2000) suggest that the photochemical production of O3 is limited by the availability of volatile organic compounds (VOCs) in February–May in Ispra.
The Milan metropolitan area is the second most densely populated area in Italy (ca. 2300 km−2), with a GDP per capita of about EUR 54 000 (EUROSTAT, 2017) and about 4100 circulating vehicles km−2 (ISTAT, 2020). Three stations in the Milan conurbation were selected as representative of the urban background in Milan city, namely “Milan via Pascal” (45.478∘ N, 9.236∘ E; 122 m a.s.l.), “Cormano” (45.548∘ N, 9.167∘ E; 155 m a.s.l.) and “Limito di Pioltello” (45.483∘ N, 9.328∘ E; 123 m a.s.l.). All three stations are operated by ARPA Lombardia. We selected only urban background stations because pollutant concentrations at traffic sites are hardly reproducible by regional air quality models with a horizontal resolution of about 10 km. The station in Milan via Pascal is located near the university, and it is considered to be the urban background station of the city, while the other two stations are located in the hinterland, near (<500 m) two main roadways used by commuters at the northern (Cormano, with about 75 000 vehicles per day) and eastern (Limito, 20 000 vehicles per day) entrances of the city. Average population densities are 7500, 4500 and 2800 km−2 in Milan, Cormano and Limito di Pioltello, respectively (ISTAT, 2020).
2.2 Measurements
At ECAtmO in Ispra, online in situ air pollution measurements are performed from appropriate inlets located at 6.5 and 9 m above ground level for gaseous and particulate pollutants, respectively. The inlet for reactive gas is made of PTFE (inner diameter: 2.7 cm). The sample residence time in the inlet tube is ca. 2 s. Each analyser samples from the main inlet through a Nafion dryer. In 2019–2020, the measurement programme included CO, NO, NOx, NO2, SO2, O3, non-methane hydrocarbons (until 6 March 2020) and NH3 (since 28 January 2020) as gaseous pollutants. The NOx (i.e. NO and NO2), SO2 and O3 data reported in this work were obtained with trace level instruments based on infrared (IR) (1200 nm) chemiluminescence and a Mo converter (Thermo Fisher 42iTL), ultraviolet (UV) (214 nm) fluorescence (Thermo Fisher 43i TLE), and UV (254 nm) absorption (Thermo Fisher 49C), respectively. These instruments are calibrated every 3 months using zero air and certified gas cylinders (NO and SO2) or a primary standard ozone generator (O3). In 2019, annual average concentrations of NO, NO2, SO2, O3 and PM10 were 4, 16, 0.4, 38 and 21 µg m−3, respectively. Particulate matter is sampled through metal-made inlets characterised by negligible losses. Each instrument samples isokinetically from the main aerosol inlet through Nafion dryers. In 2019–2020, the aerosol online in situ measurement programme included PM10 mass concentration, particle number concentration and number size distribution, particle light extinction, absorption, scattering, and backscattering at several wavelengths. The PM10 mass, particle number concentration and light absorption data reported in this work were obtained with a TEOM-FDMS (Thermo Fisher 1405-DF), a differential mobility particle sizer (home-made Vienna-type differential mobility analyser + TSI 3772 condensation particle counter) covering the particle mobility diameter range 10–800 nm and a seven-wavelength aethalometer (Magee AE31), respectively. The TEOM has been calibrated using a standard filter provided by the manufacturer, while the differential mobility particle sizer (DMPS) and the aethalometer are operated, maintained and controlled according to ACTRIS guidelines (https://www.actris.eu, last access: 9 October 2020). They were both calibrated at the specific ACTRIS central facility (https://www.actris-ecac.eu, last access: 9 October 2020) on 3–7 June 2019. Near-real-time data are available from the JRC data catalogue at http://data.jrc.ec.europa.eu/collection/abcis (last access: 9 October 2020).
The three stations in the Milan conurbation are part of the ARPA Lombardia air quality network, compliant with Directive 2008/50/EC requirements in terms of measurement methods, macro and micro localisation, and data coverage. Inlets are located at 2.5 m above ground level for all pollutants. The measurement programmes comprise NO, NOx, NO2, SO2 and O3 at all three sites. Additional measurements include benzene, toluene, xylenes, PM10, PM2.5, benzo[a]pyrene and NH3 in Milan and CO and PM10 in Limito. Each gas analyser samples from the main inlet through a Nafion dryer. The NOx data reported in this work for the Milan conurbation were obtained with trace level instruments based on IR (1200 nm) chemiluminescence and a Mo converter (Teledyne API 201E, Thermo Fisher 42i and Thermo Fisher 42c in Milan Pascal, Limito and Cormano, respectively), and O3 was measured by UV (254 nm) fluorescence (Thermo Fisher 49i at all three sites). All measurements are performed according to a specific quality assurance and quality control programme. All gas monitors are calibrated every 3 months using zero air and certified gas cylinders (NO) and every 6 months using a primary standard ozone generator for O3. The PM10 mass concentrations in the Milan conurbation reported in this work were measured using beta absorption analysers (FAI SWAM DC and 5A models in Milan Pascal and Limito, respectively). The PM analysers are checked for temperature, pressure, flow rates, leaks and other operational parameters every 3 months. A periodical comparison with gravimetric samples has been performed once yearly in Milan Pascal and upon a specific audit programme in Limito. In 2019, annual average concentrations of NO, NO2 and O3 were, respectively, 25, 37 and 46 µg m−3 in Milan Pascal; 29, 45 and 46 µg m−3 in Cormano; and 26, 34 and 44 µg m−3 in Limito. For PM10, 2019 annual averages were 29 and 31 µg m−3 in Milan Pascal and Limito, respectively. Data are available online at https://www.arpalombardia.it (last access: 9 October 2020).
2.3 CAMS regional ensemble forecast description
The Copernicus Atmospheric Monitoring Service (CAMS) provides 4 d ahead air quality forecasts daily for Europe from currently nine different regional air quality models (CHIMERE, DEHM, EMEP, EURAD-IM, GEM-AQ, LOTOS-EUROS, MATCH, MOCAGE, SILAM). Hourly pollutant concentrations are calculated for altitudes ranging from the 40 m thick surface layer to 5 km. The outputs of the different individual models are interpolated on a common regular 0.1∘ × 0.1∘ latitude × longitude grid (about 10 km × 10 km) over the European domain (30–72∘ N, 25∘ W–45∘ E). Forecasts are performed independently by all the individual regional air quality systems: each air quality model is based on different chemical (gas and aerosols) and physical parameterisations but uses the same meteorological drivers as input (the ECMWF Integrated Forecasting System, IFS) and the same anthropogenic emissions data (Kuenen et al., 2014; Denier van der Gon et al., 2015) based on 2011 emission inventories until June 2019 and on 2016 emission inventories afterwards. An ensemble (named “ENSEMBLE forecast”) is calculated from individual model outputs with a median approach (Marécal et al., 2015). This method provides an optimal estimate (Riccio et al., 2007) which is rather insensitive to outliers and generally yields better estimates than the individual models (Galmarini et al., 2018). CAMS regional air quality forecasts are routinely quality-controlled, and dedicated evaluation reports are published every third month for both individual and the ENSEMBLE models (see https://atmosphere.copernicus.eu/regional-services, last access: 9 October 2020). In this work, we used daily averages of the ENSEMBLE surface concentration forecast each day for the next 24 h (D0). For the period March–May 2019, the differences between daily mean D0 forecasts and measurements performed at various reference stations across northern Italy (expressed as median of the root mean square errors, RMSEs) were 10.5, 10.6 and 24.5 µg m−3 for NO2, PM10 and O3, respectively. Additional statistical scores are available in quarterly CAMS reports (CAMS, 2019, 2020c). Note that the actual ENSEMBLE RMSEs relative to the stations and time periods we analyzed are part of our statistical analysis described in Sect. 2.4.1.
As the anthropogenic emissions used by the individual models did not change to account for any lockdown measure, the ENSEMBLE model continued to forecast pollutants' concentrations as if the COVID-19 epidemic had not occurred in 2020.
2.4 Data analysis
2.4.1 Pollutant concentrations
To determine the specific impact of lockdown measures on concentrations of air pollutants, we compared daily observations (Obs2020) with daily expected concentrations (Exp2020) for the period 17 February–24 May 2020, which comprises the 8 lockdown weeks (D= 9 March–3 May 2020), the 3 weeks before the beginning (A= 17 February–8 March 2020) and the 3 weeks after the end of the lockdown period (P= 4–24 May 2020). NO2, PM10, NO, O3 and SO2 observed and expected concentrations are shown in Fig. 3. Expected concentrations were derived from 2020 ENSEMBLE forecasts, which account for variations in meteorological conditions and seasonal changes in emission source strengths in a “business as usual” world, i.e. without lockdown measures. However, since data from 2019 show that the agreement between ENSEMBLE forecasts (CAMS2019) and observations (Obs2019) improves from February to May (see Figs. S1–S3 in Supplement), CAMS2020 was corrected for this seasonality. Thus, 2020 daily expected pollutant concentrations (Exp) were calculated as follows:
The comparison of observations with these expected concentrations for 2020 has the great advantage of being insensitive to the fact that the emissions inventories used to calculate ENSEMBLE forecast data for 2019 and 2020 were different. The disadvantage of this approach is that Obs and Exp cannot be compared to each other on a daily basis since Exp values are affected by random variations in the Obs2019 CAMS2019 ratio. Therefore Obs2020 and Exp2020 data were compared statistically for the three periods A, D and P. Since changes in pollutant emission rates are expected to result in changes in pollutant concentrations in terms of percentages or ratios, statistical analyses were performed on Obs2020 Exp2020 daily ratios. We calculated occurrence frequency distributions of the Obs2020 Exp2020 ratio using eight class bins ranging from <0.25 to >2, all equally wide on a logarithmic scale (except the last one when specifically indicated). Cumulative frequencies of occurrence were also plotted to facilitate comparisons (Fig. 4). To detect possible specific impacts of lockdown measures on the highest concentrations, specific occurrence frequency distributions were also calculated by selecting the 28 d on which ENSEMBLE forecast data were greater than the median during the lockdown period. These days are different for each pollutant and each site. The statistical significance of the differences in Obs2020 Exp2020 ratios during the lockdown period in comparison with before and after the lockdown period (i.e. between A and D or P and D) was assessed by applying a t test assuming unequal variances to the means , and , defined as follows:
The null hypotheses ( and ) were tested at the 95 % confidence level, and results were used to determine if differences between and and and were statistically significant.
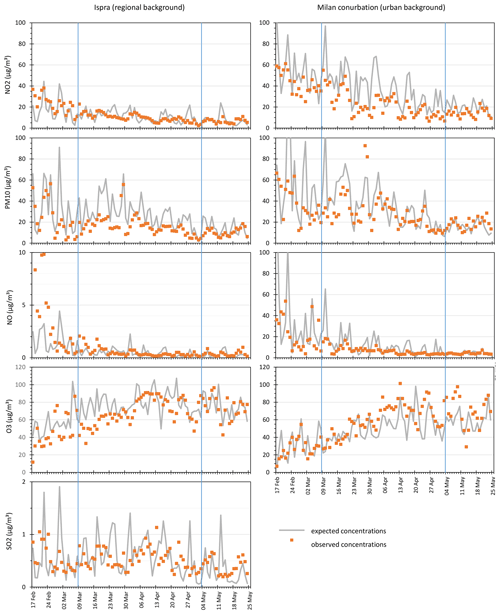
Figure 3Observed (dots) and expected (lines) 2020 concentrations (µg m−3) of NO2, PM10, NO, O3 and SO2 in Ispra (left-hand side) and the Milan conurbation (right-hand side). Vertical lines indicate the beginning and end of the lockdown period.
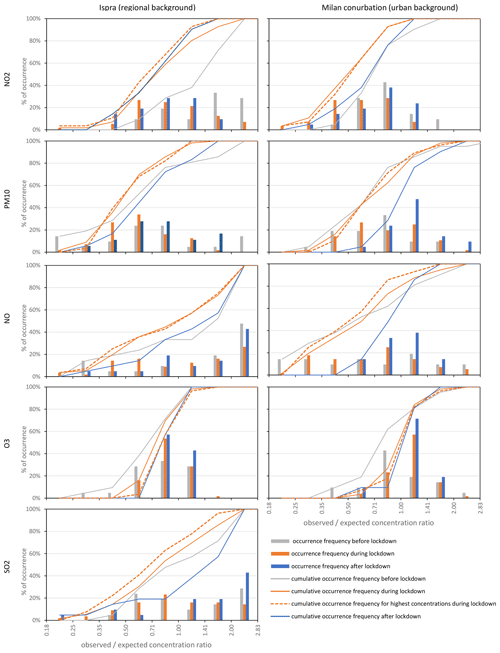
Figure 4Occurrence frequency distributions of 2020 observedexpected concentration ratios (Obs2020 Exp2020) for NO2, PM10, NO, O3 and SO2 during the lockdown period and during the 3 weeks before and after the lockdown period in Ispra (left) and the Milan conurbation (right). Lines show cumulative frequencies of occurrence. Dashed lines show the cumulative frequency of occurrence of Obs2020 Exp2020 ratios for the 28 d corresponding to the highest CAMS forecast values. Note: the last bin for NO in Ispra contains all values >2.
2.4.2 Intensive aerosol variables
To complement our analyses based on pollutant concentrations, we also looked at two characteristics of the atmospheric aerosol measured at ECAtmO in Ispra. The first one is the percentage of number of tiny particles with mobility diameters (Dp) between 15 and 70 nm as compared with the “total” number of particles with mobility diameters between 15 and 800 nm. This percentage was calculated from full particle number size distributions (10<Dp<800 nm). The smallest particles (10<Dp<15 nm) were not considered because their measurement is affected by larger uncertainties (Wiedensohler et al., 2018) and by nucleation particle bursts. The range 15<Dp<70 nm was selected as representative of particles emitted by primary sources (Giechaskiel et al., 2019; Giechaskiel, 2020; Ozgen et al., 2017; Tiwari et al., 2014). The second variable is the aerosol light absorption Ångström exponent (AÅE). It represents the wavelength dependence of light absorption by aerosol particles. AÅE values vary with particle sources and have commonly been used to apportion pollution particles between, for example, traffic and wood burning (Sandradewi et al., 2008). Traffic-emitted particles (mainly from diesel engines) have an AÅE close to 1, while particles from wood combustion have more variable AÅEs around 2 (Sandradewi et al., 2008). The mixture of pollution particles with primary or secondary aerosol of biogenic origin can also lead to AÅE values much greater than 1. Since both variables are insensitive to air pollution dispersion, they are much less variable than the extensive variables (i.e. atmospheric concentrations) they are derived from (e.g. Putaud et al., 2014). The values expected for these so-called intensive variables were calculated as the arithmetic averages observed during the 2017–2019 period.
The observation and ENSEMBLE forecast data used to estimate the values expected for the air pollution variables discussed in this section are described in Sects. 1 and 2 of the Supplement to this article. The high PM10 concentrations observed at all sites on 28 and 29 March 2020 were related to desert dust advection from the east (see maps from the World Meteorological Organisation Sand and Dust Storm Warning Advisory and Assessment System, 2021). The data from these two dates were not excluded from our statistical analysis since they did not affect its results.
3.1 Regional background (Ispra)
The trend in AÅE observed in Ispra in 2017–2019 (and also in 2020) is consistent with a decreasing contribution of wood burning to particulate pollution from winter to summer. The AÅE values measured in 2020 can of course not be compared point to point to the 2017–2019 average in Fig. 5 because the use of wood fuel for domestic heating also depends on weekend and cold-evening occurrences. However, the clear increase in the AÅE average between 9 March and 4 May 2020 compared to the 3 weeks before, the 3 weeks after and the corresponding period in 2017–2019 undoubtedly shows a change in particle sources related to lockdown measures (Table 1). A specific analysis focused on the first 4 weeks of the lockdown period (before significant numbers of biogenic aerosols are expected) suggests a −45 % reduction in aerosol from traffic (and a concomitant +45 % increase in aerosol from wood combustion) during that period.
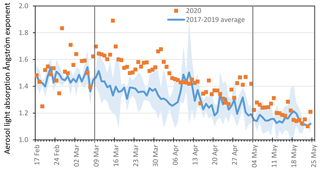
Figure 5Aerosol light absorption Ångström exponent (AÅE) in 2020 (dots) compared to its 2017–2019 average (lines). The shaded area represents ±1 standard deviation of the average. Vertical lines indicate the beginning and the end of the lockdown period.
Table 1Observedexpected concentration ratios for pollutant concentrations and aerosol characteristics before, during and after the lockdown measures in Ispra (regional background) and Milan (urban background).
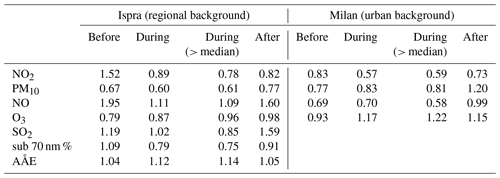
Particle number size distribution measurements in Ispra typically show modes at 25–50 nm during morning rush hours as well as in the evening in winter. Particle primary sources include fuel combustion by thermal engines and liquid (oil) or solid fuel (e.g. wood) combustion for domestic heating. The ultrafine mode diameters of primary particle emissions range from 50 to 100 nm for domestic heating (e.g. Tiwari et al., 2014; Ozgen et al., 2017) and range from 10 to 90 nm for engines (e.g. Giechaskiel et al., 2019; Giechaskiel, 2020). Measurements also show that peaks in the number of 15–70 nm particles can result from the growth of nucleation particles in the afternoon. The percentage of 15–70 nm particles generally increased from mid-February till end of May in 2017–2019 (Fig. 6). Considering that (1) wood burning combustion for domestic heating did not decrease during the lockdown period, (2) nucleation and growth of secondary aerosol particles were observed on sunny days during the lockdown period from 6 April 2020, and (3) that mostly morning peaks in particle number diminished during the lockdown period especially from 11 March to 13 April 2020, the relative “disappearance” of 15–70 nm particles during the lockdown period (Fig. 6) can be attributed to a decrease in traffic related to lockdown measures. Since the atmospheric lifetime of 15–70 nm particles is 3–12 h, local to regional traffic was concerned. Although it significantly increased after 4 May 2020, the percentage of 15–70 nm particles did not get back to the level observed before the lockdown as lockdown measures were relaxed.
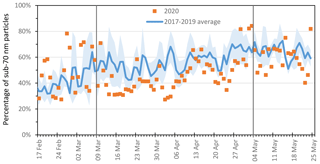
Figure 6Percentage of sub-70 nm particles in 2020 (dots) compared to 2017–2019 average (line). The shaded area represents ±1 standard deviation of the average. Vertical lines indicate the beginning and the end of the lockdown period.
The decrease in emissions from local traffic indicated by the drop in the percentage of the smallest particles (Fig. 6) is the most probable cause for the decrease in NO related to the lockdown measures in Ispra (Fig. 4). NO daily mean concentrations are indeed dominated by their morning peak corresponding to traffic rush hours (which disappears during weekends). During daytime, NO is rapidly converted to NO2, and NO concentrations reach very low steady-state values. Decreased NO emissions should therefore result in decreases in NO2. Such a decrease in NO2 (−40 % on average) actually occurred in Ispra as a result of the lockdown measures from 9 March 2020 (Fig. 4). In contrast, NO2 did not “recover” from the lockdown measures, unlike NO, of which concentrations increased again in comparison to expected concentrations as lockdown measures were relaxed on 4 May 2020 (Table 1). Due to its lifetime of about 1–2 d (Seinfeld and Pandis, 2016), NO2 can travel rather long distances. Nitrogen oxides are also emitted by large combustion sources like thermal power plants, which also emit SO2. However, our analysis of SO2 data also reveals that sources of SO2 that affect concentrations in Ispra decreased due to lockdown measures (Figs. 3 and 4). The fact that NO2 observedexpected concentration ratios remained as low after as during the lockdown period could be explained by a slower increase in traffic on the regional scale as compared to the local scale.
Regarding secondary pollutants, the highest O3 concentrations significantly increased compared to expected concentrations during the lockdown period in comparison with the 3 weeks before (Figs. 7 and 4). This suggests that O3 peaks are usually diminished by NO titration during this period of the year in Ispra and that the abatement in NOx emissions revealed by NO and NO2 data analyses led to a reduction in this effect. The relaxation of lockdown measures led to a further increase in O3. Since O3 production is generally VOC-limited in May in Ispra, this increase in O3 is probably due to an increase in anthropogenic emissions of VOCs from, for example, local traffic. In the case of PM10, which is mainly composed of secondary particulate species in Ispra (Larsen et al., 2012), no significant decrease compared to expected concentrations could be identified as lockdown measures were implemented (Figs. 3 and 4). This is because the decrease in PM10 related to traffic was compensated by the increase from wood burning for domestic heating, at least during the first half of the lockdown period. In contrast, PM10 significantly but marginally increased as lockdown measures were relaxed on 4 May 2020, at a time of the year (from May onwards) when wood burning combustion for domestic heating is largely reduced.
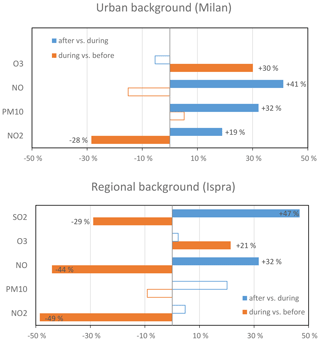
Figure 7Changes in observed and expected concentrations for the 3 weeks before and after the lockdown in comparison with the 28 d of lockdown corresponding to the CAMS daily forecasts above the median for each pollutant. Filled bars represent statistically significant differences. Empty bars represent differences that are not significantly different from zero.
3.2 Urban background (Milan conurbation)
To represent the Milan urban background, we used data from three urban background sites located in Milan's hinterland and in Milan's city centre (Fig. 1). NO2 significantly decreased (−30 % on average) compared to expected concentrations as lockdown measures were implemented (Figs. 3 and 4). NO2 significantly but not totally “recovered” when lockdown measures were relaxed (Table 1), which suggests that not all sources determining NO2 concentrations in Milan were fully reopened. However, the increase in NO after the end of the lockdown period suggests that local traffic largely resumed. Perhaps NOx emissions on a broader scale did not yet reach their usual intensity during the first 3 weeks after the end of the lockdown period, as already suggested by NO2 data from Ispra. Regarding NO, it should be noted that a significant decrease in comparison to the weeks before the lockdown period could only be detected at the city centre station (Fig. S8). Both sites in the hinterland are much closer to highways and might reflect more NO emissions from heavy-duty vehicles, whose traffic did not decrease that much, at least during the first weeks of the lockdown period.
As in Ispra, the implementation of lockdown measures on 9 March 2020 led to increases in O3 in the Milan conurbation compared to expected concentrations (Figs. 3 and 4). This can be explained by the decrease in O3 titration by NO in a pollution regime where photochemical O3 production is limited by the availability of volatile organic compounds. The relaxation of lockdown measures did not lead to the expected decrease in O3 (Fig. 4), perhaps because NOx emissions did not fully recover during the 3 weeks following the end of the lockdown period.
Again, as in Ispra, no significant change in PM10 could be detected when lockdown measures were implemented. This is very likely due to the fact that decreased emissions of PM10 (and PM10 precursors) were compensated by increases from other sources like domestic heating and enhanced formation of secondary PM. In particular, Huang et al. (2021) reported that increased oxidative capacities of the atmosphere (e.g. higher O3 concentrations) resulted from the drastic reductions in NOx emissions following from the lockdown measures in China, which in turn lead to an increase in the formation rate of nitric acid (HNO3). Such a phenomenon in northern Italy, together with sustained emissions of ammonia from agriculture, which was not affected by the lockdown (ARPA Lombardia, 2020a), could have resulted in increased formation of particulate ammonium nitrate (NH4NO3) and therefore an increase in PM10 concentrations beyond expected concentrations in the Milan conurbation. For the 3 weeks following 4 May 2020, the relaxation of lockdown measures led to a further increase in PM10 in comparison to expected concentrations in Milan. This might be attributed to the upturn in traffic and particularly to the re-suspension of dust from roads that had been little used for several weeks.
Northern Italy has been an air pollution hotspot for decades. Northern Italy also hosted the very first clusters of the COVID-19 epidemic in Europe, and from February 2020, containment measures were gradually implemented, culminating in strict lockdown measures in force between 9 March and 4 May 2020. We isolated specific impacts of the lockdown measures on air pollution by comparing observed with expected data at one regional background site (Ispra) and three urban background sites (in the Milan conurbation) across the period 17 February–24 May 2020. All four stations were in the COVID-19 “red zone”. Expected pollutant concentrations were derived from ENSEMBLE forecasts, which are based on actual meteorological conditions and historical emissions estimates that ignored the COVID-19 epidemic and related lockdown measures. Changes in observed versus computed expected concentrations for the lockdown period and the 3 weeks before and after the lockdown period should therefore directly reflect the impact of lockdown measures on air pollution.
We showed that lockdown measures had statistically significant impacts on concentrations of most gaseous pollutants (Table 1). However, we were not able to highlight systematic significant effects on PM10 concentrations.
Focusing on those days for which the ENSEMBLE model forecast concentrations were above the median for the lockdown period (Fig. 7), the following can be said:
-
NO2 concentrations decreased by about −30 % and −50 % at the urban and regional background sites, respectively, as a result of the lockdown implementation on 9 March 2020. The relaxation of lockdown measures on 4 May led to a partial recovery in NO2 concentrations in Milan (urban background) but not in Ispra (regional background).
-
Unlike NO2, PM10 concentrations were not significantly affected by the lockdown measures. We showed that the decrease in traffic-related PM10 was compensated by an increase in PM10 associated with wood burning for domestic heating in Ispra. PM10 concentrations in Milan are to a great extent influenced by PM10 “non-urban” and “non-traffic” sources (Thunis et al., 2018), including the formation of secondary aerosol. Sustained regional background PM10 concentrations and a modified HNO3 production regime associated with continuing NH3 emissions from agriculture could explain the lack of decrease in PM10 resulting from the lockdown measures in Milan too. In contrast, the relaxation of lockdown measures led to an increase in PM10 concentrations at both urban and regional background sites (+30 % and +20 %, respectively) in May, when domestic heating is much reduced.
-
The lockdown measures led to an increase in the highest O3 concentrations at both the urban and regional background sites.
The sad experience of the COVID-19 epidemic and subsequent lockdown measures shows that drastic changes in mobility and economic activity can lead to 0 % (insignificant) to −30 % reductions in air pollution in urban background areas. These figures suggest that the abatement of air pollution down to levels that do not have adverse effects on human health in northern Italy may require structural changes in other sectors, including energy production, domestic heating and agriculture in addition to transport.
Observation data from Ispra are available at https://data.jrc.ec.europa.eu/collection/abcis (European Commission, 2021) and https://actris.nilu.no/ (NILU, Norsk Institutt for Luftforskning, 2021). Observation data from Milan are available at https://www.arpalombardia.it/Pages/Aria/Richiesta-Dati.aspx (ARPA Lombardia, 2020b). Model forecast data for all sites are available at https://ads.atmosphere.copernicus.eu/cdsapp#!/dataset/cams-europe-air-quality-forecasts?tab=form (CAMS, 2020d).
The supplement related to this article is available online at: https://doi.org/10.5194/acp-21-7597-2021-supplement.
JPP, EP and LP contributed to conception and design. SMDS, FL and UDS contributed to acquisition of data. JPP, EP, LP, GL and AC contributed to analysis and interpretation of data. JPP, EP, LP, GL and AC drafted the article.
The authors declare that they have no conflict of interest.
The authors thank the Copernicus Atmosphere Monitoring Service Information, in particular the Regional Production Service. Jean-Philippe Putaud, Luca Pozzoli, Enrico Pisoni, Sebastiao Martins Dos Santos and Friedrich Lagler thank their colleagues from JRC for fruitful tele-discussions during the whole lockdown period and for helpful comments on the manuscript.
This research has been supported by the European Commission, H2020 Research Infrastructures (ACTRIS-2 (grant no. 654109) and ACTRIS IMP (grant no. 871115)).
This paper was edited by Rolf Müller and reviewed by S. Fadnavis and one anonymous referee.
ARPA Lombardia: Stima preliminare delle emissioni in Lombardia durante l'emergenza COVID-19, available at: https://www.arpalombardia.it/sites/DocumentCenter/Documents/ARIA/report-stima-emissiva-COVID19-lombardia.pdf, last access: 9 October 2020a.
ARPA Lombardia: Richiesta Dati, available at: https://www.arpalombardia.it/Pages/Aria/Richiesta-Dati.aspx, last access: 9 October 2020b.
Baldasano, J. M.: COVID-19 lockdown effects on air quality by NO2 in the cities of Barcelona and Madrid (Spain), Sci. Total Environ., 741, 140353, https://doi.org/10.1016/j.scitotenv.2020.140353, 2020.
Copernicus Atmosphere Monitoring Service (CAMS): Quarterly report on ENSEMBLE NRT productions (daily analyses and forecasts) and their verification, at the surface and above surface March–April–May 2019, available at: https://atmosphere.copernicus.eu/sites/default/files/2020-02/CAMS50_2018SC1_D5.2-3.1.ENSEMBLE-2019MAM_201908_NRTProduction_Report_v1_APPROVED_Ver1.pdf (last access: 15 January 2021), 2019.
Copernicus Atmosphere Monitoring Service (CAMS): Air quality information confirms reduced activity levels due to lockdown in Italy, 17 March 2020, available at: https://atmosphere.copernicus.eu/air-quality, last access: 9 October 2020a.
Copernicus Atmosphere Monitoring Service (CAMS): Flawed estimates of the effects of lockdown measures on air quality derived from satellite observations, 26 March 2020, available at: https://atmosphere.copernicus.eu/flawed-estimates-effects-lockdown-measures-air-quality-derived-satellite-observations, last access: 9 October 2020b.
Copernicus Atmosphere Monitoring Service (CAMS): Quarterly report on ENSEMBLE NRT productions (daily analyses and forecasts) and their verification, at the surface and above surface, available at: https://atmosphere.copernicus.eu/sites/default/files/2020-09/CAMS50_2018SC2_D5.2-3.1.ENSEMBLE-2020MAM_202008_NRTProduction_Report_v1.pdf (last access: 15 January 2021), 2020c.
Copernicus Atmosphere Monitoring Service (CAMS): CAMS European air quality forecasts, available at: https://ads.atmosphere.copernicus.eu/cdsapp#!/dataset/cams-europe-air-quality-forecasts?tab=form (last access: 15 January 2021), 2020d.
Decreto del Presidente del Consiglio dei Ministri (DPCM): Ulteriori disposizioni attuative del decreto-legge 23 febbraio 2020, n. 6, recante misure urgenti in materia di contenimento e gestione dell'emergenza epidemiologica da COVID-19, 8th of March, 2020, (20A01522) (GU Serie Generale n. 59 del 08-03-2020), 2020a.
Decreto del Presidente del Consiglio dei Ministri (DPCM): Ulteriori disposizioni attuative del decreto-legge 23 febbraio 2020, n. 6, recante misure urgenti in materia di contenimento e gestione dell'emergenza epidemiologica da COVID-19, applicabili sull'intero territorio nazionale, 11th of March 2020, (20A01605) (GU Serie Generale n. 64 del 11-03-2020), 2020b.
Decreto del Presidente del Consiglio dei Ministri (DPCM): Ulteriori disposizioni attuative del decreto-legge 23 febbraio 2020, n. 6, recante misure urgenti in materia di contenimento e gestione dell'emergenza epidemiologica da COVID-19, applicabili sull'intero territorio nazionale, 22nd of March 2020, (20A01807) (GU Serie Generale n. 76 del 22-03-2020), 2020c.
Decreto del Presidente del Consiglio dei Ministri (DPCM): Ulteriori disposizioni attuative deldecreto-legge 23 febbraio 2020, n. 6, recante misure urgenti in materia di contenimento e gestione dell'emergenza epidemiologica da COVID-19,applicabili sull'intero territorio nazionale, 26th of April 2020, Pubblicato nella Gazz. Uff. n. 108, 27 aprile 2020, 2020d.
Decreto Legge (DL): Misure urgenti in materia di contenimento e gestione dell'emergenza epidemiologica da COVID-19, 23rd of February 2020, n. 6, (20G00020) (GU Serie Generale n. 45 del 23-02-2020), 2020a.
Decreto Legge (DL): Ulteriori misure urgenti per fronteggiare l'emergenza epidemiologica da COVID-19, 16th of May 2020, n. 33, (20G00051) (GU Serie Generale n. 125 del 16-05-2020), 2020b.
Denier van der Gon, H. A. C., Bergström, R., Fountoukis, C., Johansson, C., Pandis, S. N., Simpson, D., and Visschedijk, A. J. H.: Particulate emissions from residential wood combustion in Europe – revised estimates and an evaluation, Atmos. Chem. Phys., 15, 6503–6519, https://doi.org/10.5194/acp-15-6503-2015, 2015.
European Commission: Joint Research Centre Data Catalogue, available at: https://data.jrc.ec.europa.eu/collection/abcis (last access: 9 October 2020), 2021.
European Environment Agency (EEA): Air quality in Europe – 2019 report, European Environment Agency Report No 10/2019, available at: https://www.eea.europa.eu/publications/air-quality-in-europe-2019 (last access: 25 March 2020), https://doi.org/10.2800/822355, 2019.
European Environment Agency (EEA): Air quality and COVID-19, available at: https://www.eea.europa.eu/themes/air/air-quality-and-covid19/air-quality-and-covid19, last access: 25 March 2020.
EUROSTAT: Data Browser, available at: https://ec.europa.eu/eurostat/, last access: 9 October 2020.
Galmarini, S., Kioutsioukis, I., Solazzo, E., Alyuz, U., Balzarini, A., Bellasio, R., Benedictow, A. M. K., Bianconi, R., Bieser, J., Brandt, J., Christensen, J. H., Colette, A., Curci, G., Davila, Y., Dong, X., Flemming, J., Francis, X., Fraser, A., Fu, J., Henze, D. K., Hogrefe, C., Im, U., Garcia Vivanco, M., Jiménez-Guerrero, P., Jonson, J. E., Kitwiroon, N., Manders, A., Mathur, R., Palacios-Peña, L., Pirovano, G., Pozzoli, L., Prank, M., Schultz, M., Sokhi, R. S., Sudo, K., Tuccella, P., Takemura, T., Sekiya, T., and Unal, A.: Two-scale multi-model ensemble: is a hybrid ensemble of opportunity telling us more?, Atmos. Chem. Phys., 18, 8727–8744, https://doi.org/10.5194/acp-18-8727-2018, 2018.
German Aerospace Center (DLR): Despite the influence of weather patterns, the effect of the Coronavirus on air quality is now visible, available at: https://www.dlr.de/content/en/articles/news/2020/02/20200505_effect-of-the-coronavirus-on-air-quality-is-now-visible.html, last access: 9 October 2020.
Giechaskiel, B.: Particle Number Emissions of a Diesel Vehicle during and between Regeneration Events. Catalysts, 10, 587, https://doi.org/10.3390/catal10050587, 2020.
Giechaskiel, B., Joshi, A., Ntziachristos, L., and Dilara, P.: European Regulatory Framework and Particulate Matter Emissions of Gasoline Light-Duty Vehicles: A Review, Catalysts, 9, 586, https://doi.org/10.3390/catal9070586, 2019.
Gilardoni, S., Vignati, E., Cavalli, F., Putaud, J. P., Larsen, B. R., Karl, M., Stenström, K., Genberg, J., Henne, S., and Dentener, F.: Better constraints on sources of carbonaceous aerosols using a combined 14C – macro tracer analysis in a European rural background site, Atmos. Chem. Phys., 11, 5685–5700, https://doi.org/10.5194/acp-11-5685-2011, 2011.
Grange, S. K., Lee, J. D., Drysdale, W. S., Lewis, A. C., Hueglin, C., Emmenegger, L., and Carslaw, D. C.: COVID-19 lockdowns highlight a risk of increasing ozone pollution in European urban areas, Atmos. Chem. Phys., 21, 4169–4185, https://doi.org/10.5194/acp-21-4169-2021, 2021.
Huang, X., Ding, A., Gao, J., Zheng, B., Zhou, D., Qi, X., Tang, R., Wang, J., Ren, C., Nie, W., Chi, X., Xu, Z., Chen, L., Li, Y., Che, F., Pang, N., Wang, H., Tong, D., Qin, W., Cheng, W., Liu, W., Fu, Q., Liu, B., Chai, F., Davis, S. J., Zhang, Q., and He, K.: Enhanced secondary pollution offset reduction of primary emissions during COVID-19 lockdown in China, Natl. Sci. Rev., 8, nwaa137, https://doi.org/10.1093/nsr/nwaa137, 2021.
INERIS (Institut national de l’environnement industriel et des risques): The lockdown and air quality: nitrogen-dioxide pollution falls in France’s largest cities, available at: https://www.ineris.fr/en/ineris/news/lockdown-and-air-quality-nitrogen-dioxide-pollution-falls-france-largest-cities, last access: 9 October 2020.
Istituto Nazionale di Statistica (ISTAT): Principali fattori di pressione sull'ambiente nelle città italiane, available at: https://www.istat.it/, last access: 9 October 2020.
Kuenen, J. J. P., Visschedijk, A. J. H., Jozwicka, M., and Denier van der Gon, H. A. C.: TNO-MACC_II emission inventory; a multi-year (2003–2009) consistent high-resolution European emission inventory for air quality modelling, Atmos. Chem. Phys., 14, 10963–10976, https://doi.org/10.5194/acp-14-10963-2014, 2014.
Larsen, B. R., Gilardoni, S., Stenström, K., Niedzialek, J., Jimenez, J., and Belis C. A.: Sources for PM air pollution in the Po Plain, Italy: II. Probabilistic uncertainty characterization and sensitivity analysis of secondary and primary sources, Atmos. Environ., 50, 203–213, https://doi.org/10.1016/j.atmosenv.2011.12.038, 2012.
Li, L., Li, Q., Huang, L., Wang, Q., Zhu, A., Xu, J., Liu, Z., Li, H., Shi, L., Li R., Azari, M., Wang, Y., Zhang, X., Liu, Z., Zhu Y., Zhang K., Xue S., Chel, M., Ooi, G., Zhang, D., and Chan, A.: Air quality changes during the COVID-19 lockdown over the Yangtze river delta region: An insight into the impact of human activity pattern changes on air pollution variation, Sci. Total Environ., 732, 139282, https://doi.org/10.1016/j.scitotenv.2020.139282, 2020.
Marécal, V., Peuch, V.-H., Andersson, C., Andersson, S., Arteta, J., Beekmann, M., Benedictow, A., Bergström, R., Bessagnet, B., Cansado, A., Chéroux, F., Colette, A., Coman, A., Curier, R. L., Denier van der Gon, H. A. C., Drouin, A., Elbern, H., Emili, E., Engelen, R. J., Eskes, H. J., Foret, G., Friese, E., Gauss, M., Giannaros, C., Guth, J., Joly, M., Jaumouillé, E., Josse, B., Kadygrov, N., Kaiser, J. W., Krajsek, K., Kuenen, J., Kumar, U., Liora, N., Lopez, E., Malherbe, L., Martinez, I., Melas, D., Meleux, F., Menut, L., Moinat, P., Morales, T., Parmentier, J., Piacentini, A., Plu, M., Poupkou, A., Queguiner, S., Robertson, L., Rouïl, L., Schaap, M., Segers, A., Sofiev, M., Tarasson, L., Thomas, M., Timmer mans, R., Valdebenito, Á., van Velthoven, P., van Versendaal, R., Vira, J., and Ung, A.: A regional air quality forecasting system over Europe: the MACC-II daily ensemble production, Geosci. Model Dev., 8, 2777–2813, https://doi.org/10.5194/gmd-8-2777-2015, 2015.
Nakada, L. Y. K. and Urban, R. C.: COVID-19 pandemic: Impacts on the air quality during the partial lockdown in São Paulo state, Brazil, Sci. Total Environ., 730, 139087, https://doi.org/10.1016/j.scitotenv.2020.139087, 2020.
Norsk Institutt for Luftforskning (NILU): ACTRIS Data Centre, available at: https://actris.nilu.no/ (last access: 9 October 2020), 2021.
Ozgen, S., Becagli, S., Bernardoni, V., Caserini, S., Caruso, D., Corbella, L., Dell'Acqua, M., Fermo, P., Gonzalez, R., Lonati, G., Signorini, S., Tardivo, R., Tosi, E., Valli, G., Vecchi, R., and Marinovich, M.: Analysis of the chemical composition of ultrafine particles from two domestic solid biomass fired room heaters under simulated real-world use, Atmos. Environ., 150, 87–97, doi.org/10.1016/j.atmosenv.2016.11.048, 2017.
Putaud, J. P., Cavalli, F., Martins dos Santos, S., and Dell'Acqua, A.: Long-term trends in aerosol optical characteristics in the Po Valley, Italy, Atmos. Chem. Phys., 14, 9129–9136, https://doi.org/10.5194/acp-14-9129-2014, 2014.
Riccio, A., Giunta, G., and Galmarini, S.: Seeking for the rational basis of the Median Model: the optimal combination of multi-model ensemble results, Atmos. Chem. Phys., 7, 6085–6098, https://doi.org/10.5194/acp-7-6085-2007, 2007.
Sandradewi, J., Prevot, A. S. H., Szidat, S., Perron, N., Alfarra, M. R., Lanz, V. A., Weingartner, E., and Baltensperger, U.: Using aerosol light absorption measurements for the quantitative de-termination of wood burning and traffic emission contributions to particulate matter, Environ. Sci. Technol., 42, 3316–3323, https://doi.org/10.1021/Es702253m, 2008.
Seinfeld, J. H. and Pandis, S. N., Atmospheric Chemistry and Physics: From Air Pollution to Climate Change, John Wiley & Sons, Inc., New York, USA, 2016.
Thunis, P., Degraeuwe, B., Pisoni, E., Trombetti, M., Peduzzi, E., Belis, C. A., Wilson, J., Clappier, A., and Vignati, E.: PM2.5 source allocation in European cities: A SHERPA modelling study, Atmos. Environ., 187, 93–106, doi.org/10.1016/j.atmosenv.2018.05.062, 2018.
Tiwari, M., Sahu, S. K., Bhangare, R. C., Yousaf, A., and Pandit, G. G.: Particle size distributions of ultrafine combustion aerosols generated from household fuels, Atmos. Pollut. Res., 5, 145–150, https://doi.org/10.5094/APR.2014.018, 2014.
Tonnensen, G. S. and Dennis, R. L.: Analysis of radical propagation efficiency to assess ozone sensitivity to hydrocarbons and NOx: 2. Long-lived species as indicators of ozone concentration sensitivity, J. Geophys. Res., 105, 9227–9241, https://doi.org/10.1029/1999JD900372, 2000.
Wiedensohler, A., Wiesner, A., Weinhold, K., Birmili, W., Hermann, M., Merkel, M., Müller, T., Pfeifer, S., Schmidt, A. Tuch, T., Velarde, F., Quincey, P., Seeger, S., and Nowak, A.: Mobility particle size spectrometers: Calibration procedures and measurement uncertainties. Aerosol Sci. Technol. 52, 146–164, https://doi.org/10.1080/02786826.2017.1387229, 2018.
World Meteorological Organisation Sand and Dust Storm Warning Advisory and Assessment System: Dust Forecasts, available at: https://sds-was.aemet.es/forecast-products/dust-forecasts/ensemble-forecast, last access: 15 January 2021.