the Creative Commons Attribution 4.0 License.
the Creative Commons Attribution 4.0 License.
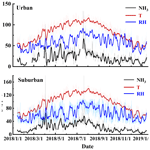
Measurement report: Exploring NH3 behavior in urban and suburban Beijing: comparison and implications
Ziru Lan
Weiwei Pu
Zhiqiang Ma
Ammonia (NH3) plays an important role in particulate matter formation; hence, its atmospheric level is relevant to human health and climate change. Due to different relative distributions of NH3 sources, concentrations of atmospheric NH3 may behave differently in urban and rural areas. However, few parallel long-term observations of NH3 exist to reveal the different behaviors of NH3 concentrations at urban and rural sites in a same region. In this study, online ammonia analyzers were used to continuously observe atmospheric NH3 concentrations at an urban site and a suburban site in Beijing from 13 January 2018 to 13 January 2019. The observed mixing ratio of NH3 averaged 21±14 ppb (range of 1.6–133 ppb) at the urban site and 22±15 ppb (range of 0.8–199 ppb) at the suburban site. The NH3 mixing ratios at the urban and suburban sites exhibited similar seasonal variations, with high values in summer and spring and low values in autumn and winter. The hourly mean NH3 mixing ratios at the urban site were highly correlated (R=0.849, P<0.01) with those at the suburban site; however, the average diurnal variations in the NH3 mixing ratios at the urban and suburban sites differed significantly, which implies different contributions from NH3 sources and sinks at the urban and suburban sites. In addition to the emission sources, meteorological factors were closely related to the changes in the NH3 concentrations. For the same temperature (relative humidity) at the urban and suburban sites, the NH3 mixing ratios increased with relative humidity (temperature). Relative humidity was the factor with the strongest influence on the NH3 mixing ratio in different seasons at the two sites. The relationships between the NH3 concentrations and temperature (relative humidity) varied from season to season and showed differences between the urban and suburban sites. The reasons for the different relationships need to be investigated in future studies. Higher wind speed mainly from the northwest sector lowered the NH3 mixing ratios at both sites. Similarly to other primary pollutants in Beijing, the NH3 mixing ratios were high when impacted by air masses from the southern sector.
- Article
(4071 KB) - Full-text XML
-
Supplement
(1008 KB) - BibTeX
- EndNote
Ammonia (NH3) is the most abundant alkaline trace gas in the atmosphere (Meng et al., 2017). An excessive NH3 concentration directly harms the ecosystem; causes water eutrophication and soil acidification; and leads to forest soil erosion, biodiversity reduction, and carbon uptake variations (Pearson and Stewart, 1993; Reay et al., 2008; Van Breemen et al., 1983; Erisman et al., 2007). NH3 can react with acidic gases to form ammonium salts, which might significantly influence the mass concentration and composition of particulate matter (Wu et al., 2009). As major components of fine particulate matter, ammonium salts contribute largely to the scattering of solar radiation and, hence, influence climate change (Charlson et al., 1991). Therefore, atmospheric NH3 is one of the key species relevant to human health as well as ecosystem and climate change.
After the implementation of policies such as the “12th Five-Year Plan for the Key Regional Air Pollution Prevention and Control in Key Regions” (Ministry of Ecology and Environment of the People's Republic of China, 2012) and the “Air Pollution Prevention and Control Action Plan” (General Office of the State Council, PRC, 2013), China, especially the capital city Beijing, has been effectively controlling the emissions of sulfur dioxide (SO2) and nitrogen oxide (NOx), which are key precursors of fine particles. However, the pollution caused by fine particles is still serious (Krotkov et al., 2016; UN Environment, 2019), particularly in winter in the North China Plain, where excess NH3 promotes haze formation through heterogeneous reactions (Ge et al., 2019). Studies have indicated that when the SO2 and NOx concentrations are reduced to a certain extent, reducing NH3 emissions is the most economical and effective method to decrease the PM2.5 concentration (Pinder et al., 2008). In China, the main anthropogenic sources of NH3 are livestock and poultry feces (54 %) and fertilizer volatilization (33 %) (Huang et al., 2012). Moreover, the atmospheric NH3 concentration in China has increased with the expansion of agricultural activities, control of SO2 and NOx, and an increase in temperature (Warner et al., 2017). This increase in the NH3 concentration might weaken the effectiveness of SO2 and NOx emission control in reducing PM2.5 pollution (Fu et al., 2017).
The North China Plain is a region with high NH3 emissions (Zhang et al., 2017), and Beijing has one of the highest NH3 concentrations in the world (Chang et al., 2016b; Pan et al., 2018). Compared with studies on pollutants such as SO2 and NOx, considerably fewer studies have been conducted on the NH3 concentration in Beijing. Chang et al. (2016a) collected gaseous NH3 samples during the 2014 APEC (Asia-Pacific Economic Cooperation) summit (18 October to 29 November 2014) in the Beijing urban area and concluded that the overall contributions of traffic, garbage, livestock, and fertilizers to the NH3 concentration were 20.4 %, 25.9 %, 24.0 %, and 29.7 %, respectively. According to the data from Huang et al. (2012), the NH3 emissions in Beijing were from livestock and poultry farming (34.55 %), nitrogen-fixing plants (33.57 %), fertilizer use (13.06 %), household garbage treatment (8.29 %), traffic emissions (5.20 %), industrial emissions (0.14 %), biomass combustion (0.42 %), and agricultural soil (0.84 %). Zhang (2016) measured the NH3 concentrations in urban and rural areas of Beijing from January to July 2014 and found that the NH3 concentration in urban areas was approximately 65 % higher than that in rural areas. Meng et al. (2011) reported that the highest NH3 concentration in Beijing occurred in summer and the lowest concentration occurred in winter, and their results indicated that traffic is a significant source of NH3 in urban areas. Zhang et al. (2018) reported the vertical variability of NH3 in urban Beijing based on 1-year passive sampling in 2016–2017 and concluded that local sources such as traffic emissions were important contributors to urban NH3. Meng et al. (2020) investigated the significant increase in winter NH3 and its contribution to the increasing nitrate in PM2.5 from 2009 to 2016, and they also concluded that vehicle exhaust was an important contributor to NH3 in urban Beijing in winter.
Currently, NH3 is not included in the routine environmental monitoring operation in China. Research data on NH3 monitoring, particularly on the synchronous observations of NH3 concentrations with a high temporal resolution in urban and suburban areas, are relatively scarce. In this study, high-time-resolution observations of NH3 were obtained simultaneously at an urban site and a suburban site in Beijing. The variation characteristics and influencing factors of the NH3 concentration were analyzed with meteorological data to provide a scientific basis for NH3 pollution control in Beijing.
2.1 Measurement sites
From January 2018 to January 2019, continuous and simultaneous observations of atmospheric NH3 were conducted at an urban site and a suburban site in Beijing. The urban site was located on the roof of the Science and Technology Building of Minzu University of China (39.95∘ N, 116.32∘ E, altitude: 102 m) and the suburban site was in the Changping Meteorological Station (40∘13′ N, 116∘13′ E; 77 m altitude). The suburban site is in the northwestern direction relative to the urban site, and the shortest distance between these two sites is approximately 32 km (Fig. 1). More farmland and grassland is located around the suburban site than the urban site.
2.2 Measurements and data acquisition
NH3 concentrations were measured using two NH3 analyzers (Economical ammonia analyzer, Los Gatos Research Inc., USA), which have a minimum detection limit of <0.2 ppb and a maximum drift of 0.2 ppb over 24 h. The NH3 analyzers were deployed in air-conditioned rooms. These analyzers use off-axis integrated cavity output spectroscopy (OA-ICOS) technology, which is a fourth-generation cavity-enhanced absorption technique, to simultaneously measure NH3 and water vapor (H2O) in the atmosphere. The incident laser beam of the OA-ICOS technology deviates from the optical axis, which differs from the traditional coaxial incidence mode. The axial incidence mode of the OA-ICOS technology can increase the optical path, stimulate additional high-order transverse modes, effectively suppress the noise of the cavity mode, reduce the cross interferences and errors due to contaminants existing in the cavity, and improve the detection sensitivity (Baer et al., 2002, 2012). The analyzer method is a quasi-absolute measurement, which theoretically does not require calibration. However, to ensure the comparability of the obtained data with other monitoring data, NH3 standard gas (Beijing AP BAIF Gases Industry Co., Ltd.) was used for a comparison measurement before the observation. The recorded concentrations were revised with respect to the reference NH3 concentration in the standard gas mixture.
Ambient air was drained at >0.4 L min−1 through Teflon lines ( o.d.) from a manifold. The lengths of the Teflon lines were designed to be as short as possible (less than 2 m from the manifold). Particulate matter was filtered by Teflon membranes with a pore size of less than 5 µm. As NH3 easily “sticks” to surfaces (such as the inside walls of tubes), heated sample lines have been suggested by many measurement studies. However, according to our laboratory test (Fig. S1), when the heating (70 ∘C) was on, there was a peak lasting 5–6 min that then deceased to normal levels in ambient air, which means that a new balancing process was established in less than 10 min. This suggests that heating is not necessarily a solution for NH3 sticking. Maintaining the relatively stable balance between adsorption and desorption of NH3 in the sampling system is important. When tested using air with different humidity levels, only very sharp changes in humidity obviously influenced and altered the balance, and a new balance required tens of minutes to be reestablished (Fig. S2). Under normal weather conditions, humidity changes in a relatively smooth way unless a quickly changing weather system, like rain, is approaching. The minute-level data were converted into hourly averages during the data analysis process, and the hourly resolution can smooth the effect caused by variations in humidity and temperature during the observation to some extent.
The balancing idea was also used to carry out multi-point calibrations on the NH3 analyzers (Fig. S3). A high mixing ratio (e.g., 400 ppb or higher) of NH3 mixing gases was firstly produced by a dynamic diluter and measured by the NH3 analyzers overnight. After the signals reached a stable level, other lower span values were switched in turn. At each span point, the measurement time was at least 40 min or longer. A linear regression function was then obtained with an R2 higher than 0.999. Nowadays, NH3 in a compressed gas cylinder is also trustworthy, as confirmed by the comparison with the NH3 standard in a permeation tube (Fig. S4).
In total, 7645 and 8342 valid hourly mean observations were obtained for the urban (Haidian) and suburban (Changping) sites, respectively. In addition, the urban and suburban meteorological data (temperature, relative humidity, wind direction, and wind speed) during the sampling period were obtained from the Haidian Meteorological Observation Station and Changping Meteorological Station, respectively.
3.1 Overall variations in the NH3 mixing ratios
Figure 2 displays the time series variations in the NH3 mixing ratios, temperatures, and relative humidity at the urban and suburban sites in Beijing. At the urban site, the mean ±1σ, median, maximum, and minimum values of the hourly average NH3 mixing ratio during the observation period were 21±14, 17, 133 and 1.6 ppb, respectively. At the suburban site, the corresponding values were 22±15, 18, 199, and 0.8 ppb, respectively. The annual average and range of the NH3 mixing ratio at the suburban site were marginally higher than those at the urban site. The characteristics of the weekly smoothed data indicate that the NH3 variations and temperature and humidity fluctuations at the two sites were practically consistent, which suggests that both sites were under the influence of similar weather systems. The hourly mean NH3 concentrations at the urban site were significantly correlated (R=0.849, P<0.01) with those at the suburban site.
Table 1 shows the comparison of the atmospheric NH3 concentrations (ppb) observed in different areas. Meng et al. (2011) obtained an average NH3 mixing ratio of 22.8±16.3 ppb for the period from 2008 to 2010 in the Beijing urban area, which is very close to our result (21±14 ppb) for 2018–2019. Therefore, the annual average NH3 mixing ratio in urban Beijing did not change significantly from 2008 to 2019. Moreover, results from this study and Meng et al. (2011) indicate that the NH3 concentrations at the urban and suburban sites were higher than those in the background areas. The observed NH3 concentrations in Beijing were higher than those in northwestern China (Meng et al., 2010) and the Yangtze River Delta region (Chang et al., 2019). The average annual NH3 concentration in the urban area of Shanghai, a megacity in Southeast China (31∘ N), was approximately 50 % lower than that in urban Beijing. This might be related to the fact that the North China Plain, in which Beijing is located, is one of the most intensive agricultural production regions in China. The differences in the soil properties of Beijing and Shanghai may be another reason for this difference, as the loss of soil NH3 can increase with an increase in the soil pH (Ju et al., 2009). Shanghai and its surrounding areas are dominated by the acidic soil of paddy fields (Zhao et al., 2009), whereas Beijing is dominated by the alkaline soils of dry lands (Wei et al., 2013). In addition, the climate in Beijing is much drier than in Shanghai; thus, less atmospheric NH3 can be removed by wet deposition in Beijing compared with Shanghai.
Table 1 also shows observational results of atmospheric NH3 from some other countries. The NH3 concentrations in the USA (Edgerton et al., 2007; Nowak et al., 2006; Zhou et al., 2019), Scotland (Burkhardt et al., 1998), Canada (Hu et al., 2014), Japan (Osada et al., 2019), and Germany (Vogt et al., 2005) were 0.23–13, 1.6–2.3, 0.1–4, 4.1, and 5.2 ppb, respectively. These values are considerably lower than those in Beijing. However, Delhi, India (Saraswati et al., 2019), exhibited a higher NH3 concentration (53.4±14.9 ppb) than Beijing did. This result might be attributed to the well-developed livestock breeding activities in Delhi. This comparison indicates that the NH3 concentration in Beijing did not change considerably in the decade before 2019; however, the NH3 concentration in Beijing is the highest of the big cities in China and much higher than those observed in developed countries in America, Europe, and Asia.
3.2 Seasonal variations
Figure 3 displays the monthly statistics for the NH3 mixing ratios at the urban and suburban sites in Beijing. The seasonal variations in the NH3 mixing ratios were very similar at the urban and suburban sites, with higher mixing ratios in the spring and summer and low values in the autumn and winter (Table 2). The daily mean concentrations fluctuated considerably in the spring, particularly in April. The highest mean NH3 concentrations at the urban and suburban sites were 42±17 and 42±8.2 ppb, respectively. Both occurred in July, when the NH3 concentrations also fluctuated considerably. On average, the seasonal NH3 mixing ratios at the urban and suburban sites can be arranged as follows: summer > spring > autumn > winter. The main grain crops in the rural area of Beijing are corn and wheat. Corn is categorized as spring corn and summer corn, which are sown in April and June, respectively. Usually, a large amount of base fertilizer is applied when planting corn, and the topdressing is applied after 2 months. Wheat is sown from September to October, and the topdressing is applied in the following spring. The volatilization of nitrogen fertilizers can cause an increase in atmospheric NH3 mixing ratios and their fluctuations in fertilization seasons (Zhang et al., 2016). In addition, the high temperature in summer should also be responsible for the high NH3 mixing ratios in this season. An increase in the temperature can increase the biological activity and, thus, enhance the NH3 production and emission. High temperature is also conducive for the volatilization of the urea and diammonium phosphate applied to crops. Moreover, the equilibrium among ammonium nitrate particles, gaseous NH3, and nitric acid is transferred to the gas phase at high temperature, which increases the NH3 concentration (Behera et al., 2013). Sewage treatment, household garbage, golf courses, and human excreta are crucial NH3 sources that are easily neglected (Pu et al., 2020). The relatively low NH3 concentrations in the autumn and winter might be caused by the decrease in NH3 emission in the soil and vegetation, the decrease in the NH4NO3 decomposition capacity at low temperatures, and the reduced human activities caused by a large floating population returning to their native locations outside of Beijing during the Spring Festival (Liao et al., 2014). In spring and summer, the NH3 mixing ratios at the suburban site were higher than those at the urban site, which might be related to the higher agricultural activity around the suburban site. In the autumn and winter, the NH3 mixing ratios at the urban site were marginally higher than those at the suburban site. In the autumn and winter seasons, the influences of agricultural activities on the NH3 concentration were weakened, whereas the influences of other sources (such as traffic sources) were enhanced. According to Wang et al. (2019), the traffic NH3 emissions per unit area in Haidian (urban site) were 3 times higher than in Changping (suburban site). This difference in traffic source emissions might have resulted in higher NH3 concentrations at the urban site than at the suburban site in the autumn and winter.
3.3 Diurnal variations
Figure 4 displays the average diurnal variations in the NH3 and H2O mixing ratios in different seasons at the urban and suburban sites in Beijing. Ambient NH3 exhibited different diurnal behaviors in different seasons.
In spring, the average diurnal variations in the NH3 mixing ratio were similar at the urban and suburban sites. The diurnal variations exhibited a single-peak pattern with high values in the daytime and low values at night. The NH3 mixing ratio began to increase in the morning, reached its maximum value at 16:00 Beijing time, UTC+8, and then decreased gradually. The lowest mixing ratios at the urban and suburban sites occurred at 03:00 and 09:00, respectively. The NH3 mixing ratio began to increase earlier at the urban site than at the suburban site. A plausible explanation for the earlier increase in NH3 emissions at the urban site is traffic emissions during the morning rush hours. In spring, the mixing ratio of NH3 was higher at the suburban site than that at the urban site, with an average difference of 4.1 ppb and a maximum difference of 6.1 ppb. The average diurnal amplitude of the NH3 mixing ratio at the suburban site was 5.3 ppb, which was higher than that (2.6 ppb) at the urban site. At the urban site, the average diurnal variations in the NH3 and H2O mixing ratios exhibited nearly opposite trends. The H2O mixing ratio had high values at night and low values during the day. At the suburban site, the variation characteristics of NH3 and H2O were very similar; however, the peak NH3 concentration occurred 5 h earlier than the peak H2O concentration. In spring, in contrast to the NH3 mixing ratio, the H2O mixing ratio at the urban site was 1279 ppm higher than that at the suburban site.
The diurnal variation in the NH3 mixing ratio at the suburban site in summer was similar to that in spring. This phenomenon was also observed in the rural areas of Shanghai by Chang et al. (2019). The diurnal variations in NH3 at the suburban site were considerably affected by the temperature and the contribution from volatile NH3 sources. However, the diurnal summer variation of NH3 at the urban site was completely different from that at the suburban site. The summer level of NH3 at the urban site was obviously lower during the daytime and evening than that at the suburban site, increased gradually from 21:00 to levels higher its suburban counterpart, dropped after reaching its peak value at 07:00, and then reached its lowest value at 14:00. This diurnal pattern (with a peak in early morning) has been observed in other areas, such as rural (Ellis et al., 2011), urban (Gong et al., 2011), and steppe areas located far away from human activity (Wentworth et al., 2016). Kuang et al. (2020) believed that such a diurnal pattern of NH3 was caused by the evaporation of dew in the morning, which resulted in the release of NH3 originally stored in the droplets. A lag was observed between the changes in the NH3 and H2O concentrations in the early morning, which supported the hypothesis of Kuang et al. (2020). In addition, the increase in the NH3 concentration in the morning might have been caused by the breakup of the boundary layer formed at night. The downward mixing of air with a higher NH3 concentration in the residual layer led to a morning increase in the NH3 concentration on the ground (Bash et al., 2010). In summer, the NH3 concentrations at the suburban site were significantly higher than those at the urban site during the daytime and first half of the night. The average diurnal amplitude of the NH3 concentration was 7.5 and 3.7 ppb at the urban and suburban sites, respectively. Similar to the situation in spring, the H2O concentrations at the urban site were significantly higher than those at the suburban site in the summer.
In autumn, the NH3 concentration at the suburban site was relatively stable and remained almost consistently lower than that at the urban site, which showed low values during the day and high values during the night, with a peak at midnight and a minimum (about 2.0 ppb lower than the peak) at 17:00. The H2O concentration was marginally lower (250 ppm) at the urban site than at the suburban site. The diurnal profiles of H2O at both sites resemble that of NH3 at the urban site, but the lowest values of H2O occurred earlier than the lowest value of NH3 at the urban site.
The diurnal patterns of NH3 and H2O in winter were similar to those in autumn, although the mixing ratios of NH3 and H2O were lower than their autumn counterparts. There were two slight differences: (1) the mixing ratios of NH3 at both sites exhibited lower fluctuations than those in autumn, and (2) the mixing ratio of NH3 at the urban site reached its minimum in winter earlier than that in autumn.
The above results indicate that although the two sites were under the influence of similar weather systems, the diurnal variations in the NH3 mixing ratios at the two sites were different in different seasons. This finding suggests that different NH3 sources and possibly sinks had different contributions to the NH3 concentrations at the urban and suburban sites. Additional studies should be conducted to better understand the behaviors of atmospheric NH3 and its influencing factors.
3.4 Effect of meteorological factors on the NH3 levels
Table 3 presents the annual and seasonal correlation coefficients between the daily means of NH3 mixing ratios and those of the temperature, relative humidity, and wind speed at the two sites. Annually, the NH3 mixing ratios at both sites were positively correlated with temperature and relative humidity and negatively correlated with wind speed, and the correlations are all highly significant. However, the correlations deteriorated somewhat in warm seasons. In summer and autumn, no significant correlations were noted between ambient NH3 and temperature at the two sites. The correlation between NH3 and wind speed in summer was much weaker than in the other seasons. The relative humidity was more strongly correlated with the NH3 concentration at the two sites than temperature, which can be perceived in Fig. 2. Also, the correlation between NH3 and relative humidity did not vary much from season to season. This implies the possibility that relative humidity exerts a certain influence on the variation of the NH3 level in the surface layer.
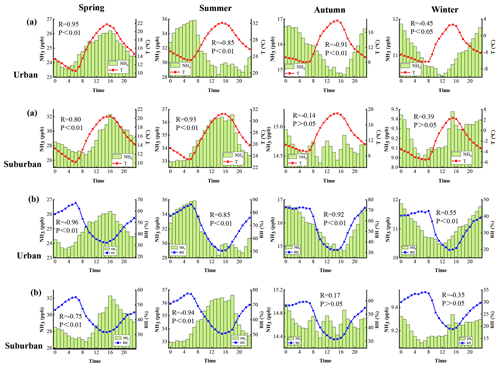
Figure 5Diurnal variations in and correlation coefficients between the NH3 mixing ratios and the respective temperature (a) and relative humidity (b) in different seasons at the urban and suburban sites in Beijing.
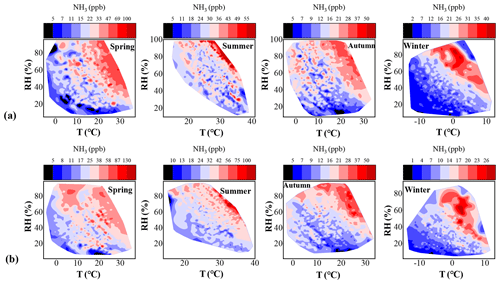
Figure 6Contour maps of the NH3 mixing ratio, temperature, and relative humidity in different seasons at the (a) urban and (b) suburban sites in Beijing.
Figure 5 displays the seasonal mean diurnal variations in the NH3 mixing ratio, temperature, and relative humidity in different seasons at the urban and suburban sites, with their correlation coefficients shown in Fig. S5. At the urban site, the seasonal hourly means of the NH3 mixing ratio were positively (negatively) correlated with those of temperature (relative humidity) in spring, but the correlations were reversed in the other seasons. At the suburban site, the seasonal hourly means of the NH3 mixing ratio were positively (negatively) correlated with those of temperature (relative humidity) in the spring and summer, but they were less correlated in autumn and winter. Similar correlation behaviors (diurnal variations) were found at both sites in spring, but the correlations (diurnal variations) at the urban site behaved differently from those at the suburban site in other seasons. The inconsistent behavior in summer, autumn, and winter caused urban–suburban differences in the annual diurnal patterns of NH3, temperature, and relative humidity as well as the NH3–temperature (relative humidity) correlations, as can be seen from Fig. S6. Figure 6 displays the contour maps of the NH3 mixing ratio, temperature, and relative humidity in different seasons at the urban and suburban sites. The annual contour maps are shown in Fig. S7. As shown in these contour maps, the NH3 mixing ratios at both sites increased with relative humidity at the same temperature and increased with temperature at the same relative humidity. Although there is some scatter in the contour maps, high NH3 levels are generally associated with high temperature and humidity. In winter, when the air temperature was low (<0 ∘C), the NH3 mixing ratios at both sites often had low values except at high humidity (>60 %). An increase in temperature caused higher NH3 mixing ratios at both sites; however, the NH3 concentration at the suburban site was more significantly correlated with temperature than that at the urban site (Table 3), suggesting that volatile NH3 sources might have a higher contribution to the NH3 concentration in suburban than in urban area. A higher amount of NH3 removal via chemical transformation is expected during the day at the urban site than at the suburban site, as the urban area had a higher relative humidity, higher particulate matter concentrations, and higher acid gas emissions (particularly NOx) than the suburban area. In 2018, the concentrations of PM2.5, SO2, and NO2 were 50, 5, and 43 µg m−3 in Haidian and 46, 6, and 35 µg m−3 in Changping, respectively, as reported by the Beijing Ecology and Environment Statement.
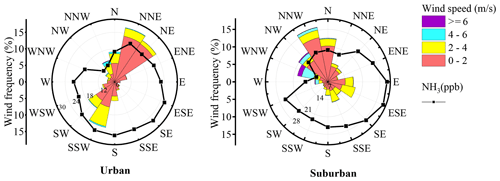
Figure 7Wind rose diagrams of the NH3 mixing ratios, wind frequency, and wind speed in different wind direction sectors.
To explore the influence of wind on the NH3 mixing ratios, wind rose diagrams were drawn for the hourly mean concentration of NH3, wind direction frequency, and wind speed during the observation period (Fig. 7). The large-scale wind circulation in the North China Plain is often influenced by the mountain–plain topography; therefore, the dominant winds in this region are southerly (from noon to midnight) and northerly (from midnight to noon) (Lin et al., 2009, 2011). As displayed in Fig. 7, some differences existed in the distributions of the surface wind between the urban and suburban sites. The prevailing surface winds were northeasterly and southwesterly at the urban site and northwesterly and easterly at the suburban site. At the urban site, the NH3 mixing ratios were relatively high when the winds originated from the southern sectors and relatively low when the winds originated from the northwestern sectors. Therefore, under southwesterly wind conditions, air masses from the south of Beijing carry not only air pollutants but also higher levels of NH3 to the urban site. Meng et al. (2017) examined the effect of long-range air transport on the urban NH3 levels in Beijing during the summer using trajectory analysis. They concluded that the air mass from the southeast has a cumulative effect on the NH3 concentration. Although the dominant wind direction at the suburban site was different from that at the urban site, the NH3 mixing ratios were also relatively high in the southern sectors. Thus, winds from the southeast, south, and southwest can elevate levels of atmospheric NH3 at both the urban and suburban sites. The NH3 mixing ratios were relatively low when air masses originated from the northwestern sector at the urban site and from the western sector at the suburban site. The western and northwestern winds were stronger and promoted the dilution and diffusion of NH3 emitted into the boundary layer.
As a water-soluble gas, NH3 can be impacted by precipitation. Heavy rainfall occurred on 18 August 2018 (Fig. 8). Before the rainfall, the NH3 concentration at the urban site was higher than the average level in August. After the rainfall, the NH3 concentration decreased rapidly, and it was significantly lower than the mean value in August. However, the diurnal pattern of NH3 on that day did not differ considerably from the average diurnal pattern in August. On the same day, the NH3 mixing ratio at the suburban site remained at a low level during the rainfall period, which was considerably lower than the August mean NH3 concentration during the same time of day. However, the NH3 mixing ratio increased rapidly after the precipitation and reached the mean level at 17:00. The rainfall might have had an obvious clearing effect on NH3, but more case studies are needed to reach a robust conclusion.
In this study, the atmospheric NH3 concentrations at an urban site and a suburban site in Beijing were continuously and simultaneously observed from January 2018 to January 2019. The mean NH3 mixing ratios were 21±14 and 22±15 ppb at the urban and suburban sites, respectively. These NH3 levels are among the highest mean values found in China and much higher than those reported for some developed countries in America, Europe, and Asia. In the summer and spring, the NH3 mixing ratios at the suburban site were slightly higher than those at the urban site. In the autumn and winter, however, the situation was reversed. The highest NH3 mixing ratios at the urban and suburban sites were all found in July. The lowest NH3 mixing ratio occurred in February at the urban site and in January at the suburban site. A comparison with data from the literature shows that the mean concentration of NH3 in Beijing did not change considerably in the decade before 2019.
The hourly mean NH3 mixing ratios at the urban site were highly correlated (R=0.849, P<0.01) with those at the suburban site. However, the mean diurnal variations in the NH3 mixing ratios at the urban and suburban sites were different. At the urban site, lower NH3 mixing ratios were observed in the daytime and higher mixing ratios were observed at night. The opposite trend was observed at the suburban site. Although both sites were under the influence of similar weather systems, the seasonal diurnal variations in the NH3 mixing ratio were different at the urban and suburban sites, suggesting that NH3 sources had different relative contributions to the NH3 levels at the urban and suburban sites.
The relationship of meteorological factors with the NH3 mixing ratio was complex. Overall, the NH3 mixing ratios increased with relative humidity and temperate at both sites. Relative humidity was more strongly correlated with the NH3 mixing ratio at both sites. The situation in different seasons varied and was site dependent, which warrants further studies. A high wind speed (mainly under northwesterly wind conditions) suppressed the levels of NH3 at both sites. The NH3 mixing ratios were higher under southerly wind conditions. Rainfall had a certain scavenging effect on NH3 but had little effect on the diurnal variations in the NH3 concentration.
The stationary measurement data are available upon reasonable request from the corresponding author, Weili Lin (linwl@muc.edu.cn).
The supplement related to this article is available online at: https://doi.org/10.5194/acp-21-4561-2021-supplement.
ZL and WL developed the idea for this paper, formulated the research goals, and carried out the measurements at the urban site. WP and ZM carried out the NH3 field observations at the suburban site.
The authors declare that they have no conflict of interest.
The authors wish to thank Xu Xiaobin for help with language editing and professor Guo Le for providing the land use map of Beijing.
This research has been supported by the National Natural Science Foundation of China (grant no. 91744206) and the Beijing Municipal Science and Technology Commission (grant no. Z181100005418016).
This paper was edited by James Allan and reviewed by two anonymous referees.
Adon, M., Yoboué, V., Galy-Lacaux, C., Liousse, C., Diop, B., Doumbia, E. H. T., Gardrat, E., Ndiaye, S. A., and Jarnot, C.: Measurements of NO2, SO2, NH3, HNO3 and O3 in West African urban environments, Atmos. Environ., 135, 31–40, https://doi.org/10.1016/j.atmosenv.2016.03.050, 2016.
Baer, D. S., Paul, J. B., Gupta, M., and O'Keefe, A.: Sensitive absorption measurements in the near-infrared region using off-axis integrated-cavity-output spectroscopy, Appl. Phys. B-Lasers O., 75, 261–265, https://doi.org/10.1007/s00340-002-0971-z, 2002.
Baer, D. S., Gupta, M., Leen, J. B., and Berman, E.: Environmental and atmospheric monitoring using off-axis integrated cavity output spectroscopy (OA-ICOS), Am. Lab., 44, 20–23, 2012.
Bash, J. O., Walker, J. T., Katul, G. G., Iones, M. R., Nemitz, E., and Robarge, W. P.: Estimation of in-canony ammonia sources and sinks in a fertilized zea mays field, Environ. Sci. Technol., 44, 1683–1689, https://doi.org/10.1021/es9037269, 2010.
Behera, S. N., Sharma, M., Aneja, V. P., and Balasubramanian, R.: Ammonia in the atmosphere: A review on emission sources, atmospheric chemistry and deposition on terrestrial bodies, Environ. Sci. Pollut. R., 20, 8092–8131, https://doi.org/10.1007/s11356-013-2051-9, 2013.
Burkhardt, J., Sutton, M. A., Milford, C., Storeton-West, R. L., and Fowler, D.: Ammonia concentrations at a site in southern Scotland from 2 yr of continuous measurements, Atmos. Environ., 32, 325–331, https://doi.org/10.1016/S1352-2310(97)00198-2, 1998.
Cao, J.-J., Zhang, T., Chow, J. C., Watson, J. G., Wu, F., and Li, H.: Characterization of Atmospheric Ammonia over Xi'an, China, Aerosol Air Qual. Res., 9, 277–289, https://doi.org/10.4209/aaqr.2008.10.0043, 2009.
Chang, Y., Liu, X., Deng, C., Dore, A. J., and Zhuang, G.: Source apportionment of atmospheric ammonia before, during, and after the 2014 APEC summit in Beijing using stable nitrogen isotope signatures, Atmos. Chem. Phys., 16, 11635–11647, https://doi.org/10.5194/acp-16-11635-2016, 2016a.
Chang, Y., Zou, Z., Deng, C., Huang, K., Collett, J. L., Lin, J., and Zhuang, G.: The importance of vehicle emissions as a source of atmospheric ammonia in the megacity of Shanghai, Atmos. Chem. Phys., 16, 3577–3594, https://doi.org/10.5194/acp-16-3577-2016, 2016b.
Chang, Y., Zou, Z., Zhang, Y., Deng, C., Hu, J., Shi, Z., Dore, A. J., and Collett, J. L.: Assessing Contributions of Agricultural and Nonagricultural Emissions to Atmospheric Ammonia in a Chinese Megacity, Environ. Sci. Technol., 53, 1822–1833, https://doi.org/10.1021/acs.est.8b05984, 2019.
Charlson, R. J., Langner, J., Rodhe, H., Leovy, C. B., and Warren, S. G.: Perturbation of the northern hemisphere radiative balance by backscattering from anthropogenic sulfate aerosols, Tellus B, 43, 152–163, https://doi.org/10.1034/j.1600-0889.1991.t01-1-00013.x, 1991.
Edgerton, E. S., Saylor, R. D., Hartsell, B. E., Jansen, J. J., and Alan Hansen, D.: Ammonia and ammonium measurements from the southeastern United States, Atmos. Environ., 41, 3339–3351, https://doi.org/10.1016/j.atmosenv.2006.12.034, 2007.
Ellis, R. A., Murphy, J. G., Markovic, M. Z., VandenBoer, T. C., Makar, P. A., Brook, J., and Mihele, C.: The influence of gas-particle partitioning and surface-atmosphere exchange on ammonia during BAQS-Met, Atmos. Chem. Phys., 11, 133–145, https://doi.org/10.5194/acp-11-133-2011, 2011.
Erisman, J. W., Bleeker, A., Galloway, J., and Sutton, M. S.: Reduced nitrogen in ecology and the environment, Environ. Pollut., 150, 140–149, https://doi.org/10.1016/j.envpol.2007.06.033, 2007.
Fu, X., Wang, S., Xing, J., Zhang, X., Wang, T., and Hao, J.: Increasing Ammonia Concentrations Reduce the Effectiveness of Particle Pollution Control Achieved via SO2 and NOX Emissions Reduction in East China, Environ. Sci. Tech. Let., 4, 221–227, https://doi.org/10.1021/acs.estlett.7b00143, 2017.
Ge, B., Xu, X., Ma, Z., Pan, X., Wang, Z., Lin, W., Ouyang, B., Xu, D., Lee, J., Zheng, M., Ji, D., Sun, Y., Dong, H., Squires, F. A., Fu, F., and Wang, Z.: Role of ammonia on the feedback between AWC and inorganic aerosol formation during heavy pollution in the North China Plain, Earth and Space Science, 6, 1675–1693, https://doi.org/10.1029/2019EA000799, 2019.
General Office of the State Council, PRC: Air Pollution Prevention and Control Action Plan, available at: http://www.gov.cn/zwgk/2013-09/12/content_2486773.htm (last access: 22 October 2020), 2013.
Gong, L., Lewicki, R., Griffin, R. J., Flynn, J. H., Lefer, B. L., and Tittel, F. K.: Atmospheric ammonia measurements in Houston, TX using an external-cavity quantum cascade laser-based sensor, Atmos. Chem. Phys., 11, 9721–9733, https://doi.org/10.5194/acp-11-9721-2011, 2011.
He, Y., Pan, Y., Zhang, G., Ji, D., Tian, S., Xu, X., Zhang, R., and Wang, Y.: Tracking ammonia morning peak, sources and transport with 1 Hz measurements at a rural site in North China Plain, Atmos. Environ., 235, 117630, https://doi.org/10.1016/j.atmosenv.2020.117630, 2020.
Hu, Q., Zhang, L., Evans, G. J., and Yao, X.: Variability of atmospheric ammonia related to potential emission sources in downtown Toronto, Canada, Atmos. Environ., 99, 365–373, https://doi.org/10.1016/j.atmosenv.2014.10.006, 2014.
Huang, X., Song, Y., Li, M., Li, J., Huo, Q., Cai, X., Zhu, T., Hu, M., and Zhang, H.: A high-resolution ammonia emission inventory in China, Global Biogeochem. Cy., 26, GB1030, https://doi.org/10.1029/2011GB004161, 2012.
Ju, X. T., Xing, G. X., Chen, X. P., Zhang, S. L., Zhang, L. J., Liu, X. J., Cui, Z. L., Yin, B., Christie, P., Zhu, Z. L., and Zhang, F. S.: Reducing environmental risk by improving N management in intensive Chinese agricultural systems, P. Natl. Acad. Sci. USA, 106, 3041–3046, https://doi.org/10.1073/pnas.0813417106, 2009.
Krotkov, N. A., McLinden, C. A., Li, C., Lamsal, L. N., Celarier, E. A., Marchenko, S. V., Swartz, W. H., Bucsela, E. J., Joiner, J., Duncan, B. N., Boersma, K. F., Veefkind, J. P., Levelt, P. F., Fioletov, V. E., Dickerson, R. R., He, H., Lu, Z., and Streets, D. G.: Aura OMI observations of regional SO2 and NO2 pollution changes from 2005 to 2015, Atmos. Chem. Phys., 16, 4605–4629, https://doi.org/10.5194/acp-16-4605-2016, 2016.
Kuang, Y., Xu, W., Lin, W., Meng, Z., Zhao, H., Ren, S., Zhang, G., Liang, L., and Xu, X.: Explosive morning growth phenomena of NH3 on the North China Plain: Causes and potential impacts on aerosol formation, Environ. Pollut., 257, 113621, https://doi.org/10.1016/j.envpol.2019.113621, 2020.
Liao, X., Zhang, X., Wang, Y., Liu, W., Du, J., and Zhao, L.: Comparative Analysis on Meteorological Condition for Persistent Haze Cases in Summer and Winter in Beijing, Environ. Sci., 35, 2031–2044, https://doi.org/10.13227/j.hjkx.2014.06.001, 2014.
Lin, W., Xu, X., Ge, B., and Zhang, X.: Characteristics of gaseous pollutants at Gucheng, a rural site southwest of Beijing, J. Geophys. Res., 114, D00G14, https://doi.org/10.1029/2008JD010339, 2009.
Lin, W., Xu, X., Ge, B., and Liu, X.: Gaseous pollutants in Beijing urban area during the heating period 2007–2008: variability, sources, meteorological, and chemical impacts, Atmos. Chem. Phys., 11, 8157–8170, https://doi.org/10.5194/acp-11-8157-2011, 2011.
Meng, Z. Y., Xu, X.-B., Wang, T., Zhang, X. Y., Yu, X. L., Wang, S. F., Lin, W. L., Chen, Y. Z., Jiang, Y. A., and An, X. Q.: Ambient sulfur dioxide, nitrogen dioxide, and ammonia at ten background and rural sites in China during 2007-2008, Atmos. Environ., 44, 2625–2631, https://doi.org/10.1016/j.atmosenv.2010.04.008, 2010.
Meng, Z. Y., Lin, W. L., Jiang, X. M., Yan, P., Wang, Y., Zhang, Y. M., Jia, X. F., and Yu, X. L.: Characteristics of atmospheric ammonia over Beijing, China, Atmos. Chem. Phys., 11, 6139–6151, https://doi.org/10.5194/acp-11-6139-2011, 2011.
Meng, Z. Y., Lin, W., Zhang, R., Han, Z., and Jia, X.: Summertime ambient ammonia and its effects on ammonium aerosol in urban Beijing, China, Sci. Total Environ., 579, 1521–1530, https://doi.org/10.1016/j.scitotenv.2016.11.159, 2017.
Meng, Z. Y., Wu, L., Xu, X., Xu, W., Zhang, R., Jia, X., Liang, L., Miao, Y., Cheng, H., Xie, Y., He, J., and Zhong, J.: Changes in ammonia and its effects on PM2.5 chemical property in three winter seasons in Beijing, China, Sci. Total Environ., 749, 142208, https://doi.org/10.1016/j.scitotenv.2020.142208, 2020.
Ministry of Ecology and Environment of the People's Republic of China: 12th Five-Year Plan for the Key Regional Air Pollution Prevention and Control in Key Regions, available at: http://www.mee.gov.cn/gkml/hbb/bwj/201212/t20121205_243271.htm (last access: 12 October 2020), 2012.
Nowak, J. B., Huey, L. G., Russell, A. G., Tian, D., Neuman, J. A., Orsini, D., Sjostedt, S. J., Sullivan, A. P., Tanner, D. J., Weber, R. J., Nenes, A., Edgerton, E., and Fehsenfeld, F. C.: Analysis of urban gas phase ammonia measurements from the 2002 Atlanta Aerosol Nucleation and Real-Time Characterization Experiment (ANARChE), J. Geophys. Res.-Atmos., 111, D17308, https://doi.org/10.1029/2006JD007113, 2006.
Osada, K., Saito, S., Tsurumaru, H., and Hoshi, J.: Vehicular exhaust contributions to high NH3 and PM2.5 concentrations during winter in Tokyo, Japan, Atmos. Environ., 206, 218–224, https://doi.org/10.1016/j.atmosenv.2019.03.008, 2019.
Pan, Y., Tian, S., Zhao, Y., Zhang, L., Zhu, X., Gao, J., Huang, W., Zhou, Y., Song, Y., Zhang, Q., and Wang, Y.: Identifying Ammonia Hotspots in China Using a National Observation Network, Environ. Sci. Technol., 52, 3926–3934, https://doi.org/10.1021/acs.est.7b05235, 2018.
Pearson, J. and Stewart, G. R.: The deposition of atmospheric ammonia and its effects on plants, New Phytol., 125, 283–305, https://doi.org/10.1111/j.1469-8137.1993.tb03882.x, 1993.
Phan, N.-T., Kim, K.-H., Shon, Z.-H., Jeon, E.-C., Jung, K., and Kim, N.-J.: Analysis of ammonia variation in the urban atmosphere, Atmos. Environ., 65, 177–185, https://doi.org/10.1016/j.atmosenv.2012.10.049, 2013.
Pinder, R. W., Gilliland, A. B., and Dennis, R. L.: Environmental impact of atmospheric NH3 emissions under present and future conditions in the eastern United States, Geophys. Res. Lett., 35, 89–90, https://doi.org/10.1029/2008GL033732, 2008.
Pu, W., Ma, Z., Collett, J. L., Guo, H., Lin, W., Cheng, Y., Quan, W., Li, Y., Dong, F., and He, D.: Regional transport and urban emissions are important ammonia contributors in Beijing, China, Environ. Pollut., 265, 115062, https://doi.org/10.1016/j.envpol.2020.115062, 2020.
Reay, D. S., Dentener, F., Smith, P., Grace, J., and Feely, R. A.: Global nitrogen deposition and carbon sinks, Nat. Geosci., 1, 430–437, https://doi.org/10.1038/ngeo230, 2008.
Saraswati, Y., George, M. P., Sharma, S. K., Mandal, T. K., and Kotnala, R. K.: Simultaneous Measurements of Ambient NH3 and Its Relationship with Other Trace Gases, PM2.5 and Meteorological Parameters over Delhi, India, Mapan-J. Metrol. Soc. I., 34, 55–69, https://doi.org/10.1007/s12647-018-0286-0, 2019.
Singh, S. and Kulshrestha, U. C.: Rural versus urban gaseous inorganic reactive nitrogen in the Indo-Gangetic plains (IGP) of India, Environ. Res. Lett., 9, 125004, https://doi.org/10.1088/1748-9326/9/12/125004, 2014.
UN Environment: A Review of 20 Years' Air Pollution Control in Beijing, United Nations Environment Programme, Nairobi, Kenya, 2019.
Van Breemen, N., Mulder, J., and Driscoll, C. T.: Acidification and alkalinization of soils, Plant Soil, 75, 283–308, https://doi.org/10.1007/BF02369968, 1983.
Vogt, E., Held, A., and Klemm, O.: Sources and concentrations of gaseous and particulate reduced nitrogen in the city of Münster (Germany), Atmos. Environ., 39, 7393–7402, https://doi.org/10.1016/j.atmosenv.2005.09.012, 2005.
Wang, K., Fan, S., Guo, J., and Sun, G.: Characteristics of ammonia emission from motor vehicle exhaust in Beijing, Environ. Eng., 36, 98–101, https://doi.org/10.13205/j.hjgc.201803020, 2019.
Warner, J. X., Dickerson, R. R., Wei, Z., Strow, L. L., Wang, Y., and Liang, Q.: Increased atmospheric ammonia over the world's major agricultural areas detected from space, Geophys. Res. Lett., 44, 2875–2884, https://doi.org/10.1002/2016GL072305, 2017.
Wei, S., Dai, Y., Liu, B., Zhu, A., Duan, Q., Wu, L., Ji, D., Ye, A., Yuan, H., Zhang, Q., Chen, D., Chen, M., Chu, J., Dou, Y., Guo, J., Li, H., Li, J., Liang, L., Liang, X., Liu, H., Liu, S., Miao, C., and Zhang, Y.: A China data set of soil properties for land surface modeling, J. Adv. Model. Earth Sy., 5, 212–224, https://doi.org/10.1002/jame.20026, 2013.
Wentworth, G. R., Murphy, J. G., Benedict, K. B., Bangs, E. J., and Collett Jr., J. L.: The role of dew as a night-time reservoir and morning source for atmospheric ammonia, Atmos. Chem. Phys., 16, 7435–7449, https://doi.org/10.5194/acp-16-7435-2016, 2016.
Wu, Z., Hu, M., Shao, K., and Slanina, J.: Acidic gases, NH3 and secondary inorganic ions in PM10 during summertime in Beijing, China and their relation to air mass history, Chemosphere, 76, 1028–1035, https://doi.org/10.1016/j.chemosphere.2009.04.066, 2009.
Yao, X. and Zhang, L.: Trends in atmospheric ammonia at urban, rural, and remote sites across North America, Atmos. Chem. Phys., 16, 11465–11475, https://doi.org/10.5194/acp-16-11465-2016, 2016.
Zhang, B.: Atmospheric Distribution and Variation of NH3 in Beijing, Environmental Science and Management, 41, 119–122, 2016.
Zhang, S., Wag, A., Zhang, Z., Wang, J., Han, Y., Su, R., and Qu, Y.: On creating an anthropogenic ammonia emission inventory in capital Beijing, J. Safety Environ., 16, 242–245, https://doi.org/10.13637/j.issn.1009-6094.2016.02.047, 2016.
Zhang, X., Wu, Y., Liu, X., Reis, S., Jin, J., Dragosits, U., van Damme, M., Clarisse, L., Whitburn, S., Coheur, P. F., and Gu, B.: Ammonia emissions may be substantially underestimated in China, Environ. Sci. Technol., 51, 12089–12096, https://doi.org/10.1021/acs.est.7b02171, 2017.
Zhang, Y., Tang, A., Wang, D., Wang, Q., Benedict, K., Zhang, L., Liu, D., Li, Y., Collett Jr., J. L., Sun, Y., and Liu, X.: The vertical variability of ammonia in urban Beijing, China, Atmos. Chem. Phys., 18, 16385–16398, https://doi.org/10.5194/acp-18-16385-2018, 2018.
Zhao, X., Xie, Y. X., Xiong, Z. Q., Yan, X. Y., Xing, G. X., and Zhu, Z. L.: Nitrogen fate and environmental consequence in paddy soil under rice-wheat rotation in the Taihu lake region, China, Plant Soil, 319, 225–234, https://doi.org/10.1007/s11104-008-9865-0, 2009.
Zhou, C., Zhou, H., Holsen, T. M., Hopke, P. K., Edgerton, E. S., and Schwab, J. J.: Ambient Ammonia Concentrations Across New York State, J. Geophys. Res.-Atmos., 124, 8287–8302, https://doi.org/10.1029/2019JD030380, 2019.