the Creative Commons Attribution 4.0 License.
the Creative Commons Attribution 4.0 License.
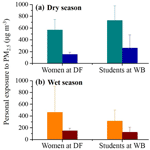
Personal exposure to PM2.5 emitted from typical anthropogenic sources in southern West Africa: chemical characteristics and associated health risks
Jean-François Léon
Cathy Liousse
Benjamin Guinot
Véronique Yoboué
Aristide Barthélémy Akpo
Jacques Adon
Kin Fai Ho
Steven Sai Hang Ho
Lijuan Li
Eric Gardrat
Zhenxing Shen
Junji Cao
Urbanization is an issue that is strongly emerging in southern West Africa (sWA). There is a lack of full understanding on chemical compositions and personal exposure levels to fine particulate matter (hereafter defined as PE PM2.5) and its health risks related to various anthropogenic sources in this region. In this study, PE PM2.5 was studied in dry (January) and wet (July) seasons of 2016 for the first time to characterize the contributions of a domestic fire site (DF) to the exposure of women and a waste burning site (WB) to that of students in Abidjan, Côte d'Ivoire, and a motorcycle traffic site (MT) to that of drivers in Cotonou, Benin.
The average PE PM2.5 mass concentrations were 331.7±190.7, 356.9±71.9 and 242.8±67.6 µg m−3 at DF, WB and MT sites for women, students and drivers, which were 2.4, 10.3 and 6.4 times the ambient PM2.5 concentrations, respectively. Elevated PE PM2.5 levels in the dry season were found at DF (358.8±100.5 µg m−3), WB (494.3±15.8 µg m−3) and MT (335.1±72.1 µg m−3) sites, on average 15 % higher than that at DF and 55 % higher at both WB and MT sites in the wet season. The seasonal variations were attributed to emission sources, meteorological factors and personal activities. In addition, the results show that geological material (35.8 %, 46.0 % and 42.4 %) and organic matter (34.1 %, 23.3 % and 24.9 %) were the major components of PE PM2.5 at DF, WB and MT sites. It is worth noting that the contribution of heavy metals was higher at WB (1.0 %) than at DF (0.7 %) and MT (0.4 %) sites, strongly influenced by waste burning emission. This results in the highest non-cancer risks of heavy metals to students, 5.1 and 4.8 times the values for women and drivers, respectively.
By conducting organic speciation, fingerprints were used to access the exposure and identify the source contributions from typical local anthropogenic sources. The women's exposure concentration to particulate polycyclic aromatic hydrocarbons (PAHs) at DF (77.4±47.9 ng m−3) was 1.6 and 2.1 times, respectively, that of students at WB (49.9±30.7 ng m−3) and of drivers at MT (37.0±7.4 ng m−3). This can be associated with the higher contributions from solid fuels' burning and meat grilling activities to women, resulting in a level 5 times in exceedance of the cancer risk safety threshold (). Phthalate esters (PAEs), commonly used as plasticizers in products, were in high levels in the student exposure PM2.5 samples (1380.4±335.2 ng m−3), owing to obvious waste burning activities nearby. The drivers' exposures to fossil fuel combustion markers of hopanes in PE PM2.5 at MT (50.9±7.9 ng m−3) was 3.0–3.3 times those for women at DF (17.1±6.4 ng m−3) and students at WB (15.6±6.1 ng m−3).
Overall, the current study shows that wood combustion, waste burning, fugitive dust and motor vehicle emissions were the dominant sources of PE PM2.5 and mainly contributed to its toxicities. The exposure to the heavy metals Pb and Mn caused high non-cancer risks to students at WB, while the severe cancer risk of PAHs was found for women at DF via inhalation. The result of this study provides original data, initial perspective of PM2.5 personal exposure and health risk assessment in the developing areas. The information encourages the governments to improve the air quality and living standards of residents in this region.
- Article
(4078 KB) - Full-text XML
-
Supplement
(771 KB) - BibTeX
- EndNote
The southern West Africa (sWA) region is experiencing an economic upturn. Anthropogenic emissions of air pollutants have been increasing in the last few years, leading to poor air quality in the region (IMF, 2017; Norman et al., 2007). Fine particulate matter (PM2.5 with equivalent aerodynamic diameters ≤2.5 µm) is one of the major concerns of international organizations and the public because of its high health impacts related to personal exposure (Bruce et al., 2000; Chen et al., 2013; Owili et al., 2017). Owili et al. (2017) found that four types of ambient PM2.5, including mineral dust, anthropogenic pollutants, biomass burning and mixed aerosols are significantly associated with under-five and maternal mortality in Africa. However, studies on PM2.5, especially tests of direct personal exposure to fine particulate matter (hereafter defined as PE PM2.5) (nonstationary sampling) and its health assessment, are very limited in these low gross domestic product (GDP) countries.
Since the 1990s, several international campaigns have been performed in Africa. Some of them were mainly focused on the particles or aerosols, such as DECAFE (Lacaux et al., 1995), EXPRESSO (Delmas et al. 1999; Ruellan et al., 1999), SAFARI-1992 (Lindesay et al., 1996), SAFARI-2000 (Swap et al., 2002), AMMA (Léon et al., 2009; Liousse et al., 2010; Marticorena et al., 2010) and INDAAF (Ouafo-Leumbe et al., 2017). In fact, Africa has the largest production of mineral dust particles from the Sahara and unpaved road surfaces (Laurent et al., 2008; Marticorena et al., 2010; Reeves et al., 2010) and carbonaceous aerosols originated from wild fires (mainly savannah fires) (Capes et al., 2008; Gaudichet et al., 1995) in the whole world. Therefore, these campaigns were more biased toward the natural sources of aerosols in Africa. In previous literature, the major contributions to the aerosol chemistry in northern Benin in the dry season were shown to be dust (26 %–59 %), primary organic matter (POC; 30 %–59 %), elemental carbon (EC; 5 %–9 %) and water-soluble inorganic ions (3 %–5 %) (Ouafo-Leumbe et al., 2017). Liousse et al. (2014) showed the increase of the relative importance of particulate emissions from domestic fires and fossil fuel combustion in Africa. Uncertainty has been raised by the residents who live in urban areas as they have concerns about the health impacts of air quality. However, works on PE PM2.5 emitted from the typical anthropogenic sources in the emerging cities in Africa are still scarce.
The main anthropogenic emission sources of PM2.5 in sWA include domestic wood burning, fossil fuel combustion, unregulated traffic and industries, waste burning and road dust. An ongoing project, Dynamics–Aerosol–Chemistry–Cloud Interactions in West Africa (Africa-DACCIWA), aims to quantify the influences of anthropogenic and natural emissions on the atmospheric pollutant composition over southern West Africa and to assess their impacts on human health, ecosystems and agricultural productivity. The information will be gathered and discussed with policymakers, scientists, operational centers, students and the general public. The current work in the framework of the Work Package 2 “Air Pollution and Health” of DACCIWA aims to link emission sources, air pollution and health impacts over representative differentiated urban sources: domestic fires and waste burning in Abidjan (Côte d'Ivoire) and two-wheel vehicle emission in Cotonou (Benin) for different groups of the population.
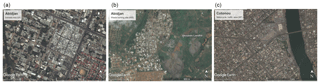
Figure 1Location of the sampling sites (white square) within the cities. (a) Domestic fire site (DF) at the Yopougon-Lubafrique market in Abidjan, (b) waste burning site (WB) at the landfill of Akeoudo in Abidjan and (c) motorcycle traffic site (MT) in the Dantokpa area in Cotonou.
Smoking meat (e.g., fish and pork) by biomass fuels (wood) is an important diet pattern for residents of coastal countries in the sWA area. Many female workers are engaged in roasting activities without any personal health protection. They are directly exposed to the high PM2.5 emissions from wood burning and smoking meat, which could cause serious health issues. In addition, urbanization has led to explosive population growth and rural depopulation in sWA, generating a huge amount of urban domestic waste. The biggest landfill in Abidjan involved in this study receives more than 1 000 000 t waste per year (Adjiri et al., 2015). Without any processing capacity or appropriate treatment methods, a large amount of air pollutants was thus emitted from the combustion and stacking of waste. Such a phenomenon damages the living environment and harms the residents' health (especially for children) in Abidjan (UNEP, 2015). Moreover, in most low-GDP countries, motorbike taxis are a major mode of local transportation (Assamoi and Liousse, 2010). In Benin, motorbike taxi drivers (mainly male) represented ∼2.5 % of the total population in 2002 (Lawin et al., 2016). Due to long daily working hours, the drivers are exposed to traffic-related PM2.5 emissions over years.
Major chemical components in PM2.5 like OC, EC and ions not only have a strong impact on PM2.5 physicochemical characteristics but also cause health risks. Typical trace toxic chemicals in PM2.5, such as heavy metals and polycyclic aromatic hydrocarbons (PAHs), cause various types of health damage to humans (Cao et al., 2012; WHO, 1998; Xu et al., 2015). For instance, Pb is a neurodevelopmental metal which affects children's health and mental development (U.S. EPA, 2012; Xu et al., 2017). Several PAHs are teratogenic and carcinogenic for humans (Tang et al., 2008). Up to now, only a few studies have investigated chemical compositions of PE PM2.5 samples, and little is known regarding the sources and health risks in the sWA region. This poses a challenge for the formulation of strategies to mitigate PM2.5 pollution and its health effects in this area.
Therefore, our study relies on the portative device sampling PM2.5 PE samples in the sWA area in 2016. Study objectives are (1) to characterize the PE PM2.5 from different typical local anthropogenic sources using chemical component and PM2.5 mass balance analysis, (2) to identify potential pollution sources to different exposed populations from fingerprints of organic markers and (3) to evaluate the PE PM2.5 health risks using the United States Environmental Protection Agency (U.S. EPA) health risk assessment model. This information offers scientific understanding of the PE PM2.5 in sWA and arouses the government's attention to protect residents' health from various anthropogenic sources.
2.1 Site description and participant selection
PE PM2.5) filter samples were collected using portative devices in unique source-dominated environments for different target groups of humans, including a domestic fire site (DF) for women and a waste burning site (WB) for students in Abidjan, Côte d'Ivoire, and a motorcycle traffic site (MT) for drivers in Cotonou, Benin (Fig. 1). Abidjan ( N, W) is the economic capital of Côte d'Ivoire, with 6.5 million inhabitants in 2016. It is characterized by the highest level of industrialization and urbanization in the sWA area. Cotonou ( N, W) is the largest city and economic center of Benin, with about 1.5 million inhabitants in 2016. Both the cities experience a tropical wet and dry mixed climate, with relatively constant ambient temperatures (in a range of 24–30 ∘C) and an average of relative humidity (RH) >80 % throughout a year.
The DF site in Abidjan is located in the market of Yopougon-Lubafrique ( N, W), which is a large courtyard with about 25 fireplaces (Fig. 2). The major fuel used is essentially hevea wood, which is a kind of local rubber tree. Several female adult workers are employed for grilling meat and/or roasting peanuts from 06:00 to 15:00 UTC (working hours) in the working day. In this study, we selected two healthy and nonsmoking female workers (an average age of 32.5 years old) to measure personal exposure to PM2.5 from domestic fires and related sources such as grilling (Fig. 2). The WB site in Abidjan is near the public landfill of Akouédo ( N, W), which has received all the waste collected from Abidjan for the past 50 years (Fig. 2). We selected two healthy and nonsmoking primary school students (an average age of 11 years old) who live and study next to the WB site (within 100 m straight-line distance) to determine the personal exposure features to PM2.5 from waste burning (spontaneous combustion at high ambient temperatures and irregular combustion by the landfill workers) emissions at landfill and other daily sources. Lastly, the MT site in Cotonou is located in the Dantokpa area ( N, E), one of the biggest markets in western Africa (Fig. 2). It is largely dominated by a mass of emissions from motorcycle traffic (two-wheeled vehicle powered by petrol, also named zemidjan in the local language) and a small quantity of other motor vehicles. We chose two healthy and nonsmoking male motorcycle drivers (an average age of 50 years old) to survey PM2.5 personal exposure from motorcycle emission and related sources (such as road dust).
Two women (woman A and B) involved in this study at DF were both in charge of cooking at home, using charcoal and butane gas as fuel (Fig. S1a, b, c in the Supplement), and daily household cleaning. One student participator (student A, boy, 8 years old) at WB was not involved in cooking activities at home (energy source for cooking is charcoal and liquefied petroleum gas, LPG) (Fig. S1a, c), but another student (student B, girl, 14 years old) is usually responsible for household cooking using solid fuels (wood) (Fig. S1d). Two motorcycle drivers (driver A and B) at MT work for a local motorcycle operation company whose working time is usually from 06:30 to 10:30, 12:00 to 17:00 and 18:30 to 21:00 UTC. They were on the road almost all the working hours and returned home for meals. They did not participate in any cooking at home (energy source for cooking is charcoal) (Fig. S1a).
2.2 Personal exposure to PM2.5 sample collection and QA ∕ QC
Twelve-hour time-integrated (daytime: 07:30 to 19:30 UTC; nighttime: 19:30 to 07:30 the next day UTC) PE PM2.5 samples were collected in two major southwestern African cities (Fig. 1) during the dry season (from 6 to 11 January) and wet season (from 5 to 10 July) in 2016. PE PM2.5 sampling was conducted for three consecutive days synchronously using the PEM (Personal Environmental Monitor) sampling devices with a SKC pump (SKC Inc., Fullerton, CA, USA) at a flow rate of 10 lpm (liters per minute). The PEM PM2.5 sampling heads were worn in the breathing zone (within 20 cm of the nose and mouth area) of participants in this study. PM2.5 was collected on 37 mm pre-baked quartz filters (800 ∘C, 3 h, QM/A®, Whatman Inc., UK). A total of 72 PE samples, including 24 samples (12 pairs of diurnal samples) for women at DF, 24 (12 pairs) for students at WB and 24 (12 pairs) for drivers at MT, were collected in this study. Moreover, 12 PE PM2.5 field blanks (one field blank for each participant in one season collected on the second day of the three consecutive sampling days) were obtained as well.
In order to verify the comparability of PE samples and data caused by nonidentical sampling devices, 10 pairs of PM2.5 samples were synchronously collected by two sets of actual PEMs with SKC pumps. The comparison results show a significant correlation between the PM2.5 mass concentrations obtained from two sampling devices (y=0.986x + 0.189, R2=0.974, P<0.0001). Identical membrane type (quartz fiber) and analytical treatments were applied in this study. After sampling, the filter samples were placed in Petri dishes, sealed with parafilm and stored in a freezer at −20 ∘C to prevent loss of mass through volatilization prior to analysis. Blank values from blank filter samples were used to account for any artifacts caused by gas absorption and subtract the background PM2.5 and chemical composition concentrations in this area.
Table 1Meteorological parameters of the studied two cities during the dry (December 2015 to March 2016) and wet (April to July 2016) seasons.
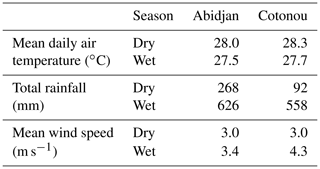
The meteorological observations during the dry (December 2015 to March 2016) and wet (April to July 2016) seasons at the sampling sites were shown in Table 1. The meteorological data were retrieved from the NOAA Global Surface Summary of the Day I (GSOD) at the airports of each cities, namely Félix Houphouët-Boigny Airport (Abidjan) and Cardinal Bernadin Gantin International Airport (Benin).
2.3 PM2.5 gravimetric and chemical analysis
PE PM2.5 filter samples were analyzed gravimetrically for mass concentrations with a high-precision electronic microbalance (Sartorius MC21S, Germany) at Laboratoire d'Aérologie (Toulouse, France) before and after sampling in the weighing room after equilibration at 20–23 ∘C and RH of 35 %–45 % for at least 24 h. The absolute errors between replicate weights were less than 0.015 mg for blank filters and 0.020 mg for sampled filters.
Total carbon (TC) was determined on 0.5 cm2 punch-out of the filters using a carbon analyzer (Ströhlein Coulomat 702C, Germany) at the Observatoire Midi-Pyrenees (OMP; Toulouse, France). The quartz filter samples were subjected to a thermal pretreatment step (kept at 60 ∘C for 20 min) in order to remove the volatile organic compounds (VOCs) and eliminate water vapor. Subsequently, the filters were combusted at 1200 ∘C under O2 and detected as CO2 in the carbon analyzer. EC was obtained using a two-step thermal method: step 1 consisted of a pre-combustion at 340 ∘C under O2 for 2 h in order to remove OC. Step 2 consisted of the oxidation of the remaining EC at 1200 ∘C under O2. The difference (TC − EC) yielded the OC concentration (Benchrif et al., 2018; Cachier et al., 2005).
To extract the water-soluble inorganic ions from the quartz filters, a quarter of the filter was placed in separate 15 mL vials containing 10 mL distilled–deionized water (18.2 MΩ cm resistivity). The vials were placed in an ultrasonic water bath and shaken with a mechanical shaker for 45 min (15 min, three times) to extract the ions. The extracts were filtered through 0.45 µm pore size microporous membranes. After that, three anions (Cl−, and ) and five cations (Na+, , K+, Mg2+ and Ca2+) in aqueous extracts of the filters were determined using an ion chromatograph (IC) analyzer (Dionex-600, Dionex, Sunnyvale, CA, USA), which is equipped with an AS11-HC anion column and a CS12 cation column for separation. Details of the IC measurement method are described in Bahino et al. (2018) and Cachier et al. (2005).
One element of Fe (representing earth's crust emission) and 10 heavy metals (i.e., V, Cr, Mn, Co, Ni, Cu, Zn, Sb, Ba and Pb) in PE PM2.5 samples were determined using Energy Dispersive X-Ray Fluorescence (ED-XRF) spectrometry (the PANalytical Epsilon 5 ED-XRF analyzer, PANalytical B.V., the Netherlands) with a quarter of the filter. The relative errors for all measured elements were <6 % between the NIST Standard Reference Material (SRM) 2783 and our ED-XRF results, which is well within the required range of error, demonstrating the accuracy of method. Replicate analysis of one quartz-fiber filter sample (five times) yielded an analytical precision between 5.2 % and 13.9 %. Details of the ED-XRF measurements are shown in Brouwer (2003) and Xu et al. (2012).
Aliquot punches (0.1–1.0 cm2) from the quartz filters were used to quantify organic compounds, including PAHs, phthalate esters (PAEs) and hopanes (details of target organic species and their abbreviations are shown in Table 5) using an in-injection port thermal desorption gas chromatography–mass spectrometry (TD-GC/MS) method. The approach has the advantages of a shorter sample preparation time (<1 min), minimizing contamination from solvent impurities, and a higher sensitivity, compared with the traditional solvent extraction GC/MS method. The detailed analytical procedures have been reported in previous publications (Ho and Yu, 2004; Ho et al., 2008, 2011; Xu et al., 2013, 2016a). The results of the blank analyses showed only traces of contamination (<5.0 %) of PE PM2.5 sample concentrations.
2.4 Health risk assessment model
A number of heavy metals and toxic organic species are associated with negative PE health effects (Škrbic et al., 2016; Val et al., 2013; J. Z. Wang et al., 2017; Xu et al., 2018a). In this study, four heavy metals (Mn, Ni, Zn and Pb) and all measured PAH and PAE species in PE PM2.5 were selected to determine the PE inhalation health risks (Xu et al., 2018a). The heavy metal non-carcinogenic risks and toxic organics carcinogenic risks of PM2.5 via inhalation were calculated according to the U.S. EPA health risk assessment model (U.S. EPA, 2004, 2011). The average daily exposure dose (D) via inhalation was estimated to assess the risk using Eq. (1) as follows:
The definitions and recommended values of parameters are shown in Table 2.
A hazard quotient (HQ) for the non-cancer risk of heavy metals in PE PM2.5 samples can be obtained from Eq. (2):
The threshold value of RfD indicates whether there is an adverse health effect during a certain period. The hazard index (HI) can be obtained by summing up the individual HQs to estimate the total non-cancer risks. If HI < 1, then a non-carcinogenic effect is impossible; if HI ≥ 1, an adverse health effect is likely to appear (Hu et al., 2012).
The incremental lifetime cancer risk (ILCR) of PAHs and PAEs in PE PM2.5 samples can be calculated by multiplying the cancer slope factor (CSF) of PAHs and PAEs by D using Eq. (3):
For cancer risk, the value of is internationally accepted as the precautionary or threshold value above which the risk is unacceptable (Jedrychowski et al., 2015).
It is worth noting that, among the 19 PAHs, benzo[a]pyrene (BaP) has been used as an indicator of PAH carcinogenicity (Wang et al., 2006). The carcinogenic health risk of PAH species can be assessed by [BaP]eq instead (Yassaa et al., 2001) using Eq. (4):
Additionally, the carcinogenic risk for PAEs was assessed by bis(2-ethylhexyl) phthalate (DEHP), which is identified as possibly carcinogenic to humans by the International Agency for Research on Cancer (IARC, 1982; Li et al., 2016). The definitions and recommended values of the parameters in Eqs. (2)–(4) are also shown in Tables 2 and 3.
2.5 Questionnaire and time-activity diary
A questionnaire (Supplement A–C) and time-activity diary (Supplement D) were collected from each participant during the sampling period, respectively, to fully grasp the basic information, potential personal exposure sources and activities of participants. In the questionnaire, each participant was asked for personal information, family status, dermatological and asthma issues, medical history, current health status and so on. In addition, the questions for women include (1) living habits and environment (past and current living conditions, general living habits, cooking habits and domestic fuel type/usage); (2) work environment and travel habits (workplace, work nature, working hours and daily travel mode/time); and (3) whether they are affected by the burning of domestic solid fuels and roasting meat. The questions for students include (1) living habits and environment (past and current living conditions, general living habits, participation in household duties, family cooking habits and domestic fuel type/usage, distance from home to WB site); (2) school environment and travel habits (school location and related environment and daily travel mode/time); and (3) whether they are affected by the burning of waste and household air pollution sources. The questions for drivers include (1) living habits and environment (past and current living environments, general living habits, participation in household duties, family cooking habits and domestic fuel type/usage); (2) working environment and travel habits (motorcycle power type, driving conditions, working hours and daily travel mode/time); and (3) whether they are affected by the motorcycle emission and household air pollution sources.
The time-activity diaries requested the participants to record information on a half an hour basis (sleeping time excluded) to assess in detail what time is spent in each microenvironment on different activities.
3.1 Personal exposure to PM2.5 and its chemical compositions
3.1.1 PE PM2.5 mass concentration
The average PE PM2.5 mass concentrations were 331.7±190.7, 356.9±71.9 and 242.8±67.6 µg m−3 for women at the domestic fire site (DF), students at the waste burning site (WB) and drivers at the motorcycle traffic site (MT), respectively, in this study. Among the three types of subjects, the average concentrations of PE PM2.5 for women and students were quite similar, ∼40 % higher than that of the drivers. PE PM2.5 ranged from 106.2 µg m−3 (nighttime in the dry season, 7 January) to 1164.7 µg m−3 (daytime in the wet season, 5 July) for women at DF; from 37.8 µg m−3 (nighttime in the wet season, 8 July) to 1137.0 µg m−3 (daytime in the dry season, 11 January) for students at WB; and from 65.0 µg m−3 (nighttime in the wet season, 11 July) to 648.5 µg m−3 (daytime in the dry season, 15 January) for drivers at MT. The ranges and standard deviations of PE PM2.5 concentrations were extremely large, especially for women, because the direct combustion sources were close to the participants. The variations of physical activities and intensities of air pollution sources potentially led to a drastic fluctuation of PE PM2.5.
Table 4Statistical analysis (arithmetic mean ± standard deviation) of personal exposure to PM2.5 mass concentrations and the chemical compositions (units: µg m−3) during the sampling period in the sWA region.
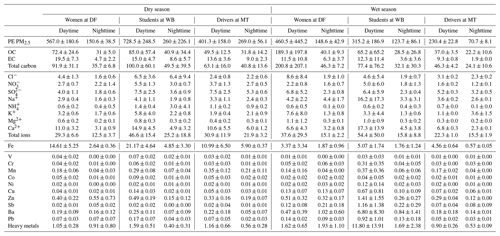
The average mass concentrations of PE PM2.5 were 358.8±100.5, 494.3±15.8 and 335.1±72.1 µg m−3 in the dry season (January) and 304.6±284.5, 219.5±71.3 and 150.6±10.4 µg m−3 in the wet season (July) for women at DF, students at WB and drivers at MT, respectively (Table 4). Compared to the dry season, the reduction rate of PE PM2.5 for women at DF in the wet season was approximately 15 %, while sharp reductions by more than 50 % were observed for students and drivers. PE PM2.5 concentrations reducing could be attributed to the occurrence of increased levels of rainfall in the wet season in sWA (Table 1), which cause a large reduction of road dust being exposed to drivers and limit the spontaneous combustion of garbage significantly around students. Moreover, the large-scale transport of mineral dust and combustion aerosols emitted by savannah wild fires contributed significantly to the aerosol load during the dry season (Djossou et al., 2018), which is more important at WB and MT than at DF (as women worked in a crowded community environment).
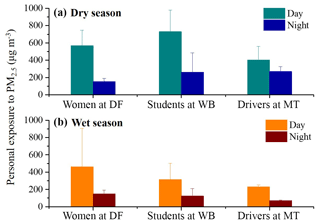
Figure 3Personal exposure to PM2.5 mass concentrations of women at DF, students at WB and drivers at MT in the dry season (January) and wet season (July) of 2016 in the sWA area.
The PE PM2.5 mass concentrations in the daytime were much higher than those at nighttime in dry or wet seasons (Table 4 and Fig. 3). The 12 h averaged PE PM2.5 concentrations showed day ∕ night (D ∕ N) ratios of 3.4 (3.8 in the dry season and 3.1 in the wet season), 2.7 (2.8 and 2.5) and 2.4 (1.5 and 3.3) for women at DF, students at WB and drivers at MT, respectively. Intensive human activities during the daytime, such as solid fuel combustion, waste combustion or motor vehicle emission influenced the different group subjects, elevating the exposure levels of PM2.5. In the same case, lower PE PM2.5 for students at WB in the nighttime can be explained by the fact that the participants usually spend most of their time indoors with limited physical activity, leading them to stay away and/or shelter from obvious emission sources (e.g., waste combustion) outdoors. Moreover, large fluctuations of D ∕ N ratios for drivers were observed, with a lower average in the dry season but a higher average in the wet season. Wet season high D ∕ N ratios are attributed to the increase in precipitation in Cotonou (Table 1), especially during nighttime (Sealy et al., 2003). This leads to the lower PE PM2.5 for drivers at night after aerosol scavenging. Shorter driving time in the wet season is another explanation for the phenomenon because of occasion unfavorable weather (e.g., rain and storms).
The 5 h PM2.5 average personal exposure concentration was 1574 µg m−3 (±287, n=3) for open wood fires in households in the Njombe district of Tanzania (Titcombe and Simcik, 2011) and was comparable to the highest 12 h exposure level to PM2.5 for women at the DF site in this study (1164.7 µg m−3, daytime in the wet season, 5 July). It was 4.7 times the daily average PE PM2.5 concentration in dry and wet seasons (331.7±190.7 µg m−3). Student (10–17 years old) PM2.5 exposures ranged from less than 10 µg m−3 to more than 150 µg m−3 (mean 56 µg m−3) in four neighborhoods in Accra, Ghana (Arku et al., 2015), much lower than that for students at the WB site (356.9±71.9 µg m−3). It can be seen that the high PM2.5 exposure of students in this study was likely related to waste burning emissions, while there was no obvious strong PM2.5 emission source in the study of Arku et al. (2015).
The average PE PM2.5 levels are compared to the weekly ambient PM2.5 concentrations (Djossou et al., 2018) in the same area during a similar sampling period. The average PE PM2.5 levels were 3.0 and 2.0 times the ambient values at DF and 6.1 and 8.8 times the ambient values at MT in dry and wet seasons, respectively. The highest PE PM2.5 to ambient (A) (PE ∕ A) ratios were found at WB, i.e., 10.3 in the dry and 10.5 in the wet season. Such large PE ∕ A ratios are probably due to the impact of waste combustion affecting the respiratory exposure of residents, especially children; on the other hand, high PE ∕ A ratios can be attributed to the fact that the WB site is located in the lowest living quality region of Abidjan, where the simplest stoves and nonqualified wood as fuel are used in the house (Fig. S1d). This leads to an extremely high PE PM2.5 indoors during the cooking time (especially for student B, who is in charge of cooking, recorded in the activity logging and questionnaire). Meanwhile, the ambient PM2.5 sampling equipment at WB was neither fixed very close to nor located at the downwind direction of the landfill (Djossou et al., 2018), which causes the differences between the ambient and PE PM2.5 concentrations.
Moreover, the daytime PE and ambient PM2.5 mass concentrations on the same sampling dates were also compared. The average women daytime PE PM2.5 levels were 3.7 and 1.2 times the ambient PM2.5 at DF in dry and wet seasons, respectively, consistent with the finding from the weekly comparison mentioned above. However, for the students at WB and drivers at MT, the PE ∕ A ratios were both much lower than those compared with the weekly ambient PM2.5, with averages of 5.1 and 7.0 for the students at WB and 1.9 and 3.3 for the drivers at MT in dry and wet seasons, respectively. The PE ∕ A ratios for students had the highest values, which is consistent with the results found earlier. The PE ∕ A ratios all above 1.0 and large variability of PM2.5 between PE and ambient concentrations imply that fixed-point sampling is likely to underestimate the PE PM2.5 and consequent human health hazards. The results further confirm the importance of portative PE PM2.5 sampling for health risk assessment.
3.1.2 PE PM2.5 chemical compositions
Table 4 summarizes the average PE PM2.5 chemical compositions, including carbon fractions (OC and EC), water-soluble inorganic ions and target heavy metals. TC was the highest composition in PE PM2.5, accounting for 24.4±4.5 %, 16.6±2.0 % and 17.8±4.9 % of PE PM2.5 for women, students and drivers, respectively. High OC values suggest the strong contribution of combustion sources to PE PM2.5 in sWA (Djossou et al., 2018; Ouafo-Leumbe et al., 2017). The average OC concentration (83.2 µg m−3) and composition (24.4 %) in women's PE PM2.5 samples were the highest among the three types of PE participants, due to their direct contact with the ignition and closeness to the solid fuel (wood in this study) burning/meat roasting at the workplace or their own residential units. However, the EC concentrations (8.4–10.5 µg m−3) and compositions (3.0 %–3.5 %) were very similar among the three different PE groups, showing that EC was less affected by human activities related to combustion sources in this study.
The OC to EC ratio (OC ∕ EC) has been used to determine emission and transformation characteristics of carbonaceous aerosols (Cao et al., 2008). The OC ∕ EC averaged 9.9±5.3 for women at DF, 6.1±0.7 for students at WB and 5.8±2.7 for drivers at MT. Previous studies (Cachier et al., 1989; Cao et al., 2005a, 2008; Li et al., 2009; Tian et al., 2017; Watson et al., 2001) characterized the average OC ∕ EC ratio of 1.1 as motor vehicle exhaust, 2.7 as coal combustion and 9.0 as biomass burning from their source samples (i.e., fresh emissions/plumes). In the present study, the OC ∕ EC suggests that biomass burning was the main contributor to PE carbonaceous aerosols for women at DF, while the mixed emissions of biomass and coal burning or/and motor vehicle exhaust were dominant for students at WB and drivers at MT. The OC ∕ EC was mostly higher in the wet season than the dry season, ascribed to the fact that the higher RH in the wet season favors the formation of secondary organic carbon (SOC) (Huang et al., 2014). The daytime OC ∕ EC for drivers' PE samples were relatively low (an average of 3.7) and constant between wet and dry seasons, promising that motor vehicle exhaust was the most dominant and stable pollution source in their working environment. The PE of women displays a higher (an average of 13.9) and more scattered OC ∕ EC ratio than those measured for students and drivers in the wet season (Fig. 4). This was induced by particularly high and dramatic changes in individual exposure to obvious carbonaceous aerosol sources (e.g., wood burning and grilling).
With the data shown in Djossou et al. (2018), the PE OC ∕ EC ratios for the participants were 1.2 and 2.5 times the ambient OC ∕ EC ratios in dry and wet seasons at DF, 1.7 and 2.8 times at WB and 1.1 and 2.0 times at MT. Such higher OC ∕ EC values in PE samples can be resulted from specific individuals' activities and potential contamination at microenvironments (Crist et al., 2008; Meng et al., 2009). In addition, the influences of precipitation and other meteorological factors on OC ∕ EC in ambient samples were less than those in PE samples (i.e., dry season OC ∕ EC was more comparable between the ambient and PE samples).
The average concentrations of total quantified water-soluble inorganic ions were 23.6±12.8, 35.5±18.3 and 22.7±5.0 µg m−3 for women at DF, students at WB and drivers at MT, accounting for 8.5±1.0 %, 12.1±2.7 % and 11.9±0.4 % of PE PM2.5 masses, respectively. Dissimilar to the compositions in heavy polluted cities in China (, and were the most abundant ions in ambient or PE PM2.5, accounting for 50 %–90 % of quantified ions and ∼30 % of PM2.5 masses) (Xu et al., 2016b, 2018b; Zhang et al., 2013), Ca2+, a marker of fugitive dust, was the most abundant ion, accounting for ∼28 % (in a range from 25.3 % to 29.3 %) of total quantified ions, followed by Cl−, and K+ for women at DF, Na+, and Cl− for students at WB and , Na+ and for drivers at MT. The profiles thus indicate that the particle resuspension by personal activities was the main contributor to the PE PM2.5 in sWA (Chen et al., 2017; Xu et al., 2015). The diurnal variations in the composition of Ca2+ to total ions (i.e., daytime = 30.6 % and nighttime = 22.8 %) also illustrate this conclusion. Moreover, forms primarily through the atmospheric oxidation of SO2 emitted mainly from coal and diesel combustion (Seinfeld and Pandis, 2006; Xu et al., 2016b). As the second most enriched ion, the average proportion of was 17.7 %, which implies that purification of raw coal and diesel (Wang et al., 2013) should be applied in this area to lower sulfur emissions and therefore decrease PE to in PM2.5. The exposure levels for the drivers were 33 % and 40 % higher than the women and students, respectively, indirectly indicating that the emission of SO2 might be higher in Cotonou or the participants are exposed to higher SO2 or from the diesel vehicle emissions.
Generally, Na+ and Cl− ranked the third and fourth abundant ions in the PE samples. The sampling sites in sWA cities in this study are all close to the sea and were affected by sea salt particles. It is also worth noting that biomass burning marker K+ (Kang et al., 2004; T. Zhang et al., 2014) displayed a high absolute average concentration of 3.4 µg m−3 and composition of 14.5 % in women's PE PM2.5 samples, confirming their particular exposure to biomass burning during roasting in the workplace. To the best of the authors' knowledge, derives from NOx emitted mainly from motor vehicle exhaust (especially gasoline vehicle), industry and power plants (Seinfeld and Pandis, 2006; Xu et al., 2016b). An additional consideration is that the industry is not well developed in this area (i.e., much less industry in Cotonou than Abidjan) and thus is not the main contributor to PM2.5 (Ouafo-Leumbe et al., 2017). In comparison with the findings from the other two sites, motor vehicle emission obviously contributed to drivers' PE concentrations, consistent with the conclusion for as discussed above.
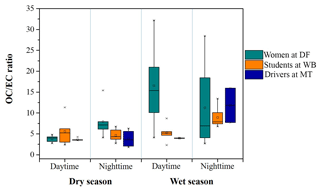
Figure 4Variations of OC ∕ EC ratios in personal exposure to PM2.5 samples for women at DF, students at WB and drivers at MT. (The box plots indicate the average concentration and the min, 1st, 25th, 50th, 75th, 99th and max percentiles).
The concentrations of 10 targeted heavy metals, including V, Cr, Mn, Co, Ni, Cu, Zn, Sb, Ba and Pb, are also shown in Table 4. The total concentrations were 1.4±0.3, 3.9±6.5 and 0.8±0.2 µg m−3 for women at DF, students at WB and drivers at MT, accounting for 0.7±0.4 %, 1.0±1.2 % and 0.4±0.1 % of the PE PM2.5, respectively. The PE to heavy metals for the students was 1.8 and 3.9 times those for the women and drivers, mainly due to the emissions from garbage combustion at landfill (Y. Wang et al., 2017). The D ∕ N ratios ranged from 0.8 to 2.1 for women and drivers but averaged 4.0 and 7.0 in dry and wet seasons, respectively, for students. This can be explained by two reasons: the first is that there were intense physical activities by the students and strong disturbances by landfill workers. Another reason is the spontaneous combustion of waste occurring frequently during the day due to less precipitation and higher ambient temperature in the daytime. Ba, Zn and Mn were found to be the dominant heavy metals, accounting for ∼73 % of the total quantified elemental concentration in all samples. Ba had a decisive advantage over other elements, having a contribution of >50 % for students. It is usually added to rubber and plastic products to improve acid and alkali resistance. Such products were main fractions of the garbage at landfill in this area (Feng et al., 2006). Zn and Mn ranked the first and second highest personal exposure elements for drivers at MT, which are mainly derived from motor oil additives, tyre wear and brake pad wear (Zhao and Hopke, 2006).
3.2 Mass balance of personal exposure to PM2.5
The calculation of the mass balance of the PE PM2.5 is an effective method to figure out the principal components in PM2.5 and distinguish the pollution sources (Gokhale et al., 2008). PE PM2.5 mass in this study can be classified into six parts: organic matter (OM), EC, water-soluble inorganic ions, geological material (GM), heavy metals and an unresolved fraction (Fig. 5). The first five main resolved fractions can explain 78.3 % to 90.6 % of total PE PM2.5 mass concentrations in this study. The unresolved fraction may include water and other undetected substances. For OM, since there are no full organic composition profiles for the PE PM2.5, a conversion factor of 1.4 (1.4 corrects the organic carbon mass for other constituents associated with the organic carbon molecule) is generally used (Turpin and Lim, 2001) to quantify OM using Eq. (5):
OM accounted for 34.1±6.3 %, 23.3±2.8 % and 24.9±6.9 % of the PE PM2.5 mass for women at DF, students at WB and drivers at MT, respectively. The results show that there are distinct sources of PE OC for women at DF. According to the information gathered from the questionnaires, the combustion sources, such as roasting meat/peanuts and burning wood, are the major contributors to PE OC for women in this study.
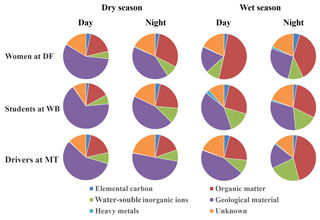
Figure 5Personal exposure to PM2.5 mass concentration closures for women at DF, students at WB and drivers at MT in different sampling seasons.
In addition, Fe has been widely used to estimate the upper limit of GM (Taylor and McLennan, 1985). Fe constitutes ∼4.0 % of dust of the earth's crust (Cao et al., 2005b; Hao et al., 2007; Kabata-Pendias and Mukherjee, 2007; Sun et al., 2014; Wu et al., 2012; Xu et al., 2016b). The amount of GM is calculated using Eq. (6):
It is found that GM contributed 35.8±2.1 %, 46.0±3.7 % and 42.4±4.7 % of PE PM2.5 mass concentrations for women at DF, students at WB and drivers at MT, respectively. Fugitive dusts, including road dust resuspension from the disturbance of motor vehicles and human activities, construction dust from uncovered construction sites and the dusts generated from burning, could be the dominant sources of PE PM2.5 in this study. OM and GM showed similar proportions (34.1 % and 35.8 %, respectively) of PE PM2.5 mass for women at DF. The fractions of GM in PE samples for students and drivers were approximately 10 % and 7 % higher than that for women. Therefore, the fugitive dust was the most important source of PE PM2.5 in this less developed area, shown by nearly 50 % contribution for students and drivers, attributable to human physical activities and a large amount of covered land. It is surprising to note that the secondary formed ions (i.e., , and ) and the total quantified water-soluble inorganic ions were shown to have exceedingly low proportions of PE PM2.5 for all groups. This reconfirms the limited contribution to PE PM2.5 from secondary ionic formation.
In Fig. 5, evident diurnal distinctions can be observed in the two major chemical compositions of OM and GM. GM exhibited a lower proportion at nighttime (35.3 %) than daytime (47.5 %), suggesting its close relationship with human activities. Higher GM was found for all groups in the dry season because of harmattan haze which introduced mineral dusts and the lack of precipitation which increased road dust resuspension. Moreover, OM showed equal or lower proportions in the daytime (25.0 %) than nighttime (30.0 %), relative to the meteorological parameters (i.e., factors that affected the formation of secondary organic carbonaceous aerosol) and diurnal changes of combustion sources around subjects. An exception is that OM proportion of women's PE PM2.5 in the daytime (50.8 %) was much higher than nighttime (38.2 %) in the wet season, due to influences of damp wood burning during working hours. Burning biomass fuel with high moisture often results in low combustion efficiency, a long smoldering period and high air pollutant emissions (Grandesso et al., 2011; Shen et al., 2012, 2013). The emission factor of OC usually increases with the fuel moisture content (Chen et al., 2010; Keita et al., 2018). Therefore, burning damp wood led to higher OC emission than dry wood, in line with the observation for women's PE results in this study.
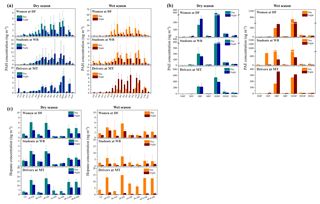
Figure 6Distributions of (a) PAHs, (b) PAEs and (c) hopanes in PM2.5 personal exposure samples for women at DF, students at WB and drivers at MT in dry and wet seasons of 2016.
Organic fingerprint markers can be used to indicate specific emission sources and further characterize the pollution that impacts different populations. The average PE concentrations of PM2.5-bound PAHs, PAEs and hopanes were 54.8±20.3, 986.8±82.2 and 27.9±1.0 ng m−3, respectively, representing high organic pollution in the sWA region (Table 5). Dissimilar to the trend in PM2.5 masses (students > women > drivers), the PE to target organic compounds of different groups were varied, with a descending order of women > students > drivers for PAHs, students > women > drivers for PAEs and drivers > women > students for hopanes (Table 5 and Fig. 6).
4.1 PAHs
Benzo[b]fluoranthene (BbF) was the most abundant PAH for women at DF, followed by benzo[a]pyrene (BaP) and indeno[1,2,3-cd]pyrene (IcdP). The average concentration of BbF (a marker of low temperature combustion, such as wood burning) was 11.6±19.2 ng m−3, accounting for approximately 15.0 % of the ΣPAHs for women (Wang et al., 2006) (Table 5). The most abundant PAH species for students at WB and drivers at MT were IcdP (6.4±4.5 ng m−3) and benzo[ghi]perylene (BghiP) (6.4±0.5 ng m−3), respectively, indicating the contributions from waste incineration and/or high temperature combustion of fuel (e.g., gasoline vehicle emission) (Baek et al., 1991; Wang et al., 2006). The average ΣPAHs of women at DF (125.4±54.8 ng m−3) and drivers at MT (44.6±10.8 ng m−3) in the wet season were 326 % and 52 % higher than those in the dry season (29.4±5.6 and 29.4±4.4 ng m−3, respectively), while ΣPAHs in the wet season (36.8±15.7 ng m−3) were 42 % lower than that dry season (62.9±45.0 ng m−3) for students at WB. The dramatic increase in the PE of women to PAHs is mainly due to an increase in moisture content in the wood used for grilling meat in the wet season, promoting more PAH emission from wood combustion processes (Shen et al., 2013). The restraint of waste combustion in the wet season is the main factor for the lower PE PM2.5-bound PAHs at landfill, in accordance with seasonal patterns of PE PM2.5 mass. Fanou et al. (2006) measured the PE PAH concentrations in Cotonou and found that the PAH level associated with particles ranged from 76.21 to 103.23 ng m−3 for 35 motorbike taxi drivers in March 2001. Our values for drivers at the MT site were 50 %–64 % lower than their values, suggesting that the exposure to PAHs for the motorbike drivers in this region has been improved.
In Fig. 6a, PE PAHs showed fluctuating diurnal variations for the three kinds of subjects. For the women at DF, the daytime concentrations in wet and dry seasons were both higher than those at nighttime due to the intensive roasting meat and burning wood during working hours. For the students at WB, PE PAHs at night were higher in the dry season but lower in the wet season. Both the PAH profiles showed high combustion markers of BbF and benzo[e]pyrene (BeP) and high gasoline vehicle emission markers of dibenzo[a,h]anthracene (DahA) and BghiP (Baek et al., 1991; Wang et al., 2006). For the drivers at MT, the average D ∕ N ratios in dry and wet seasons were 0.8 and 0.3, respectively. The higher PE ΣPAH concentrations at night and lower D ∕ N ratios for drivers may be explained by the potential combustion sources of PAHs close to the participants (e.g., sources nearby the drivers' homes) in Cotonou, Benin, especially in the wet season. This can be deduced by the combustion marker of BaP, which was the highest PAH species at night in the wet season, even though the drivers were exposed to traffic emissions during the night working hours (18:30 to 21:00 UTC). Further studies are thus required to confirm findings and figure out reasons for them.
In the study of Titcombe and Simcik (2011), the authors found that the 5 h average total PAH personal exposure concentration was 5040 ng m−3 (±909 ng m−3, n=3) for open wood fires in households in the Njombe district of Tanzania, which was much higher (∼65 times) than women's exposure to PAHs at the DF site in the current research. The highest 12 h exposure to PAHs for women at the DF site in this study was 469.7 ng m−3 (daytime in the wet season, 6 July), approximately one-tenth of the PAH concentrations from open wood fires in Tanzania mentioned above. The large PE PAH concentration difference between these two studies may be influenced by many factors such as wood type, combustion state, stove structure and sampling time.
Diagnostic ratios of PAHs have been widely used in source identification (Tobiszewski and Namiesnik, 2012; Yunker et al., 2002). In our study, the average values of BeP ∕ (BeP + BaP) and IcdP ∕ (IcdP + BghiP) were 0.47 and 0.52 for women at DF, 0.51 and 0.52 for students at WB and 0.64 and 0.34 for drivers at MT, respectively (Fig. 7), indicating the unique impacts on the PE PM2.5 from different atmospheric pollution sources. The average BeP ∕ (BeP + BaP) ratios ranged from 0.47 to 0.64, comparable with those reported in the Chinese megacities of Guangzhou (0.41–0.72) and Xi'an (0.59–0.73) (Li et al., 2005; Xu et al., 2018c), but lower than the value measured in Shanghai (>0.70) (Feng et al., 2006). This implies the low oxidability of the PAHs in the less-developed cities in sWA. PAHs in drivers' PE samples were more prone to aging (i.e., the average ratio was 1.3–1.4 times those for women and students) because of their re-suspension onto road dusts (i.e., longer residence lifetime) and longer outdoor activity time (i.e., exposure to more sunlight). Fine and ultra-fine particle-bound PAHs are emitted in high-temperature combustion from motor vehicular engine, which are more easily photochemically oxidized in the atmosphere (Baek et al., 1991; Lima et al., 2005). The differences of BeP ∕ (BeP + BaP) ratios between dry and wet seasons were not obvious, without a general pattern. However, the ratio exhibited a significant day–night variation, with an average of 0.59 and 0.49 in the daytime and nighttime, respectively. This shows that more favorable meteorological conditions (i.e., higher light intensity) and more physical activities (i.e., extended time for particulate resuspension) in the daytime are more conducive to the aging of PM2.5 and its bounded PAHs. Moreover, IcdP ∕ (BghiP + IcdP) ratios of <0.2, 0.2–0.5 and >0.5 were used to identify petrogenic, petroleum combustion and a mix of grass, wood and coal combustion, respectively (Yunker et al., 2002). The relatively low ratio for drivers at MT (0.34) demonstrates that the PAHs were mainly produced from motor vehicles, while grass, wood and coal combustion was more dominant for women at DF (0.52) and students at WB (0.52) (Fig. 7). The IcdP ∕ (IcdP + BghiP) ratio did not show significant seasonal variation.
4.2 Phthalate esters (PAEs)
Phthalate esters are widely used as plasticizers in materials and can be released into the air through matrix evaporation and combustion (Gu et al., 2010; J. Z. Wang et al., 2017). The PE levels of PAEs could be mainly attributed to the usage of household products, painting material, plastic waste incineration and municipal sewage release (L. B. Zhang et al., 2014). The total concentrations of six phthalate esters (the first six species of PAEs in Table 5) and one plasticizer (bis(2-ethylhexyl) adipate, DEHA) (abbreviated as ΣPAEs for the total seven species) were 882.0±193.3, 1380.4±335.2 and 698.1±192.4 ng m−3, respectively, for women at DF, students at WB and drivers at MT (Table 5). Bis(2-ethylhexyl) phthalate (DEHP) was the most dominant PAE species, followed by di-n-butyl phthalate (DBP) for all the three groups of participants. DEHP is mainly used as a plasticizer for the manufacture of polyvinyl chloride (PVC); and together with DBP, they are the most widely used PAEs globally (Meng et al., 2014). The average DEHP and DBP concentrations were 543.6 and 304.6 ng m−3, accounting for approximately 55.1 % and 30.9 % of the ΣPAEs, respectively (Fig. 6b). The elevated ΣPAEs for students can be ascribed to the combustion of plastic products at landfill nearby. Our results are similar to previous studies conducted in Xi'an and Tianjin, China (Kong et al., 2013; J. Z. Wang et al., 2017). The ΣPAEs ranged from 376.6 to 1074 ng m−3 outdoors and from 469.2 to 1537 ng m−3 in classrooms (J. Z. Wang et al., 2017), where DEHP and DBP were also the most abundant PAEs, with a sum of composition of 68 % and 73 % of the ΣPAEs outdoors and indoors, respectively.
The average concentrations of the ΣPAEs for women at DF, students at WB and drivers at MT were comparable in the dry season. However, the average concentrations were 927.2±154.9, 1929.8±340.4 and 594.6±16.6 ng m−3 in the wet season, 1.1, 2.3 and 0.7 times the ΣPAEs in the dry season (Fig. 6b). A significant increase in PE ΣPAEs for students at WB can be attributed to the enhanced PAE emission in the daytime with high RH (3173.6±1028.3 ng m−3), consistent with the findings on PE PM2.5. Dry and wet seasons had similar PAE profiles with different diurnal variations (Fig. 6b). The average D ∕ N ratios of the ΣPAEs in the dry season demonstrate constant concentrations, with an average of 1.0, 1.0 and 1.3, respectively, for women, students and drivers, while much larger variations of 1.1, 4.6 and 0.7 were found for the wet season. Noticeably different diurnal D ∕ N ratios for students at WB are interrelated with human activities (especially the emissions from plastic materials) and the decreased waste combustion influenced by meteorological conditions (i.e., more precipitation at night in the wet season), which was mentioned in Sect. 3.1.1.
4.3 Hopanes
Hopanes are markers for fossil fuel (e.g., petroleum) combustion (Simoneit, 1999; Wang et al., 2009). The average PE to the sum of eight quantified hopanes (Σhopanes) for the drivers was 50.9±7.9 ng m−3, 2.0 and 2.3 times higher than the women (17.1±6.4 ng m−3) and students (15.6±6.1 ng m−3), respectively (Table 5). The results indicate an extremely high personal respiratory exposure contribution from the motor vehicle emissions (e.g., gasoline combustion) for the drivers. It is important to note that number of automobiles is rapidly increasing in sWA cities, further exacerbating the air pollution and consequential health issues. The Σhopanes showed unobvious seasonal variations for three types of PE participants. The Σhopanes in the dry season were 0.9, 1.8 and 0.7 times those in the wet season. Even though the Σhopanes varied among the three sites, their profiles on individual species were similar. 17α(H)-21β(H),30-norhopane (αβ-NH) and 17α(H)-21β(H)-hopane (αβ-HH) were the two most abundant hopanes in all PE PM2.5 samples, with average concentrations of 6.0 and 6.5 ng m−3 and compositions of 21.4 % and 23.3 % of the Σhopanes, respectively (Table 5 and Fig. 6c).
Compared with D ∕ N ratios of ΣPAHs and ΣPAEs, Σhopanes exhibited a more stable diurnal trend, higher in the daytime due to heavier traffic emissions. For women at DF, the D ∕ N ratio was both 2.0 in dry and wet seasons, with Σhopanes of 24.0±11.1 and 12.2±5.0 ng m−3 for daytime and nighttime in the dry season and 21.4±17.5 and 10.9±3.6 ng m−3 in the wet season. The D ∕ N ratio of Σhopane for drivers at MT had the highest value of 11.5, with 78.0±19.1 and 44.9±16.4 ng m−3 for daytime and nighttime in the dry season and 74.2±16.3 and 6.5±1.7 ng m−3 in the wet season. It is notable that the daytime concentrations for drivers were comparable between the two seasons, while the nighttime hopanes in the wet season were mostly washed away by rainfall, resulting in a very large decline in its concentrations.
Even though these organic groups are not major fractions in PE PM2.5, their fingerprints can more accurately illustrate the contributions of air pollution sources to PM2.5. PAHs, PAEs and hopanes are source markers for combustion activities, plastics emissions and fossil fuel emissions (e.g., from gasoline vehicles), respectively, well matching the potential air pollution sources that impact the PE PM2.5 for participants in this study. Our results not only indicate that the PM2.5 respiratory exposure can be strongly contributed from environmental pollution sources and individual activities but also prove the study to be a reliable application of organic tracers for the characterization of personal exposure.
Non-cancer risks of four heavy metals (i.e., Mn, Ni, Zn and Pb) and cancer risks of PAHs and PAEs via inhalation exposure for women at DF, students at WB and drivers at MT are shown in Table 6. In general, the non-carcinogenic risks of Mn and Pb were relatively higher than those of Ni and Zn but still well below the international threshold value of 1.0. Among those four metals, the hazard quotient (HQ) of Pb in the wet season for students at WB was the highest (), which suggests that the Pb non-carcinogenic risk to children is more severe in that area compared with other participants and metals. There was no consistent difference in the risks between dry and wet seasons, except Ni, which showed a much greater value in the wet than the dry season for all participants. Counting the total of four toxic heavy metals, hazard index (HI) values for participants are also shown in Table 6. The dry ∕ wet season ratios of HI were 0.9, 0.5 and 2.3 for women, students and drivers, respectively, suggesting that the non-cancer risk of PE to metals in PM2.5 for drivers was significantly higher in dry than wet seasons, owing to a mass of fugitive dust on the road at low RH. Moreover, the average HI levels were , and for the women, students and drivers, respectively. The highest non-cancer health risks of the heavy metals in PE PM2.5 for students were 5.1 and 4.8 times those for women and drivers. Overall, Mn, Zn, Ni, Pb and HI were all below the safety limit for the populations involved in this study, representing negligible non-cancer risks of heavy metals in PE PM2.5 in the sWA region.
As shown in Table 6, the ILCRs of PAHs all exceeded the international acceptable level of , both in dry and wet seasons. Meanwhile, the ILCRs of PAEs were all below , well within the safety limits of cancer risk. For all target participants, higher cancer risks of PE PM2.5-bound PAHs and PAEs were found in the wet season. The seasonal variations such as an increase of RH could lead to an increase in cancer risks of toxic organics in PE PM2.5. In the dry season, the average ILCR values of PAHs for women and drivers were comparable, both ∼50 % lower than those for students, implying the high toxicity originated from the waste burning and the high sensitivity of young people. In the wet season, PAHs exhibited the highest ILCR for women at DF, 2.5 and 2.7 times those for students and drivers, respectively. The domestic wood burning and meat grilling can trigger nearly 10 times the safety limit for PAHs. The cancer risks of PAEs showed similar trends in dry and wet seasons (Yang et al., 2011), with the descending order of students > women> drivers. The carcinogenic risks of PAEs for the drivers were the lowest, much lower (45 % and 76 % for dry and wet seasons) than those for students who live close to waste incineration. In a word, the ILCRs of PAHs exceeded the threshold value of for all the participants, indicating that the carcinogenic PAHs are a threat to individuals' health and subsequently alert to the need for effective emission control in sWA. Even though PAEs had low carcinogenic risks, the effects from waste burning on students should not be ignored, and proper control measures for both PM2.5-bound heavy metals and toxic organic must be established.
In addition, it should be noted that both non-cancer and cancer risks could be potentially underestimated since many toxic chemical components were not involved in this study. Based on the current study, there are a variety of emission sources that impact population groups in the sWA region to different degrees. Attention should be paid to the health risks of chemicals via inhalation, especially Pb and Mn for students at the WB site as well as PAHs for women at DF in the wet season.
This work can be regarded as a first attempt at the assessment of personal exposure to fine particulate matter originating from main sources of combustion aerosols in representative cities of southern West Africa. We targeted different groups of people exposed to domestic fires, traffic and waste burning in this study. Even though there are few drawbacks such as a relatively short sampling period and a limited number of participants, our findings provide a new insight into the health risks due to PM2.5 exposure in areas with scarce observations. Developing countries of southern West Africa are facing a great challenge regarding air pollution mitigation strategies, and more investigations on personal exposure and related potential health effects using a cohort method will be considered in the future. In the short term, developing and implementing appropriate preventive and control measures on anthropogenic combustion sources downtown, such as improving waste treatment equipment at landfill or making smoking equipment for domestic use more efficient, are appropriate actions.
Requests for data sets and materials should be addressed to Hongmei Xu (xuhongmei@xjtu.edu.cn).
The supplement related to this article is available online at: https://doi.org/10.5194/acp-19-6637-2019-supplement.
HX and CL conceived and designed the study. HX, J-FL, CL and BG contributed to the literature search, data analysis and interpretation and writing of the paper. J-FL, CL, BG, VY, AA, KH, SH, ZS and JC contributed to revision of the paper. HX, J-FL, EG, JA and LL conducted the particulate sample collection and chemical experiments and analyzed the experimental data.
The authors declare that they have no conflict of interest.
This article is part of the special issue “Results of the project “Dynamics–aerosol–chemistry–cloud interactions in West Africa” (DACCIWA) (ACP/AMT inter-journal SI)”. It is not associated with a conference.
The authors thank all the personal exposure sampling participants who helped a lot in this study.
This research has been supported by the Natural Science Foundation of China (grant no. 41503096 and 41877376), the European Union Seventh Framework Program (FP7/2007-2013) under grant agreement no. 603502 (EU project DACCIWA: Dynamics–aerosol–chemistry–cloud interactions in West Africa), the open fund of the Jiangsu Key Laboratory of Atmospheric Environment Monitoring and Pollution Control (grant no. KHK1712), a project funded by the Priority Academic Program Development of Jiangsu Higher Education Institutions (PAPD) and the open fund of the State Key Laboratory of Loess and Quaternary Geology, Institute of Earth Environment, CAS (grant no. SKLLQG1722).
This paper was edited by Dominick Spracklen and reviewed by three anonymous referees.
Adjiri, O. A., Mafou, C. K., and Konan, P. K.: Impact of Akouedo landfill (Abidjan-Côte d'Ivoire) on the populations: socio-economic and environmental study, available at: http://www.ijias.issr-journals.org/abstract.php?article=IJIAS-15-235-01 (last access: 24 May 2017), 2015.
Arku, R. E., Dionisio, K. L., Hughes, A. F., Vallarino, J., Spengler, J. D., Castro, M. C., Agyei-Mensah, S., and Ezzati, M.: Personal particulate matter exposures and locations of students in four neighborhoods in Accra, Ghana. J. Expo. Sci. Environ. Epidemiol., 25, 557–566, 2015.
Assamoi, E. and Liousse, C.: Focus on the impact of two wheel vehicles on African combustion aerosols emissions, Atmos. Environ., 44, 3985–3996, 2010.
Baek, S. O., Fleld, R. A., Goldstone, M. E., Kirk, P. W., Lester, J. N., and Perry, R.: A review of atmospheric polycyclic aromatic hydrocarbons: sources, fate and behavior, Water Air Soil Poll., 60, 279–300, 1991.
Bahino, J., Yoboué, V., Galy-Lacaux, C., Adon, M., Akpo, A., Keita, S., Liousse, C., Gardrat, E., Chiron, C., Ossohou, M., Gnamien, S., and Djossou, J.: A pilot study of gaseous pollutants' measurement (NO2, O2, NH3, HNO3 and O3) in Abidjan, Côte d'Ivoire: contribution to an overview of gaseous pollution in African cities, Atmos. Chem. Phys., 18, 5173–5198, https://doi.org/10.5194/acp-18-5173-2018, 2018.
Benchrif, A., Guinot, B., Bounakhla, M., Cachier, H., Damnati, B., and Baghdad, B.: Aerosols in Northern Morocco: Input pathways and their chemical fingerprint, Atmos. Environ., 174, 140–147, 2018.
Brouwer, P.: Theory of XRF: Getting acquainted with the principles, PANanalytical BV, the Netherlands, 58 pp., 2003.
Bruce, N., Perez-Padilla, R., and Albalak, R.: Indoor air pollution in developing countries: a major environmental and public health challenge. Special Theme-Environment and Health, B. World Health Organ., 78, 1078–1092, 2000.
Cachier, H., Bremond, M. P., and Buat-Menard, P.: Carbonaceous aerosols from different tropical biomass burning sources, Nature, 340, 371–373, 1989.
Cachier, H., Aulagnier, F., Sarda, R., Gautier, F., Masclet, P., Besombes, J.-L., Marchand, N., Despiau, S., Croci, D., Mallet, M., Laj, P., Marinoni, A., Deveau, P.-A., Roger, J.-C., Putaud, J.-P., Dingenen, R. V., Dell'Acqua, A., Viidanoja, J., Santos, S. M.-D., Liousse, C., Cousin, F., Rosset, R., Gardrat, E., and Galy-Lacaux, C.: Aerosol studies during the ESCOMPTE experiment: an overview, Atmos. Res., 74, 547–563, 2005.
Cao, G., Zhang, X., Gong, S., and Zheng, F.: Investigation on emission factors of particulate matter and gaseous pollutants from crop residue burning, J. Envrion. Sci., 20, 50–55, 2008.
Cao, J. J., Xu, H. M., Xu, Q., Chen, B. H., and Kan, H. D.: Fine particulate matter constituents and cardiopulmonary mortality in a heavily polluted Chinese city, Environ. Health Persp., 120, 373–378, 2012.
Cao, J. J., Wu, F., Chow, J. C., Lee, S. C., Li, Y., Chen, S. W., An, Z. S., Fung, K. K., Watson, J. G., Zhu, C. S., and Liu, S. X.: Characterization and source apportionment of atmospheric organic and elemental carbon during fall and winter of 2003 in Xi'an, China, Atmos. Chem. Phys., 5, 3127–3137, https://doi.org/10.5194/acp-5-3127-2005, 2005a.
Cao, J. J., Rong, B., Lee, S. C., Chow, J. C., Ho, K. F., Liu, S. X., and Zhu, C. S.: Composition of indoor aerosols at emperor Qin's terra-cotta museum, Xi'an, China, during summer, 2004, China Part., 3, 170–175, 2005b.
Capes, G., Johnson, B., McFiggans, G., Williams, P. I., Haywood, J., and Coe, H.: Aging of biomass burning aerosols over West Africa: Aircraft measurements of chemical composition, microphysical properties, and emission ratios, J. Geophy. Res.-Atmos., 113, D23, https://doi.org/10.1029/2008JD009845, 2008.
Chen, L.-W. A., Verburg, P., Shackelford, A., Zhu, D., Susfalk, R., Chow, J. C., and Watson, J. G.: Moisture effects on carbon and nitrogen emission from burning of wildland biomass, Atmos. Chem. Phys., 10, 6617–6625, https://doi.org/10.5194/acp-10-6617-2010, 2010.
Chen, R. J., Zhao, Z. H., and Kan, H. D.: Heavy smog and hospital visits in Beijing, China, Am. J. Resp. Crit. Care., 188, 1170–1171, 2013.
Chen, X. C., Jahn, H. J., Engling, G., Ward, T. J., Kraemer, A., Ho, K. F., Yim, S. H. L., and Chan, C. Y.: Chemical characterization and sources of personal exposure to fine particulate matter (PM2.5) in the megacity of Guangzhou, China, Environ. Pollut., 231, 871–881, 2017.
Crist, K. C., Liu, B., Kim, M., Deshpande, S. R., and John, K.: Characterization of fine particulate matter in Ohio: Indoor, outdoor, and personal exposures, Environ. Res., 106, 62–71, 2008.
Delmas, R. A., Druilhet, A., Cros, B., Durand, P., Delon, C., Lacaux, J. P., Brustet, J. M., Serca, D., Affre, C., and Guenther, A.: Experiment for regional sources and sinks of oxidants (EXPRESSO): an overview, J. Geophys. Res.-Atmos., 104, 30609–30624, 1999.
Djossou, J., Léon, J.-F., Akpo, A. B., Liousse, C., Yoboué, V., Bedou, M., Bodjrenou, M., Chiron, C., Galy-Lacaux, C., Gardrat, E., Abbey, M., Keita, S., Bahino, J., Touré N'Datchoh, E., Ossohou, M., and Awanou, C. N.: Mass concentration, optical depth and carbon composition of particulate matter in the major southern West African cities of Cotonou (Benin) and Abidjan (Côte d'Ivoire), Atmos. Chem. Phys., 18, 6275–6291, https://doi.org/10.5194/acp-18-6275-2018, 2018.
Fanou, L. A., Mobio, T. A., Creppy, E. E., Fayomi, B., Fustoni, S., Møller, P., Kyrtopoulos, S., Georgiades, P., Loft, S., Sanni, A., Skov, H., Øvrebø, S., and Autrup, H.: Survey of air pollution in Cotonou, Benin-air monitoring and biomarkers, Sci. Total Environ., 358, 85–96, 2006.
Feng, S. L., Wang, X. M., Wei, G. J., and Peng, P. A.: The leaching behavior and genotoxic assessment of heavy metals in the municipal solid waste incineration bottom ashes from Macao, Asian J. Ecotoxicol., 4, 330–335, 2006.
Gaudichet, A., Echalar, F., Chatenet, B., Quiseft, J. P., Malingre, G., Cachier, H., Buat-Menard, P., Artaxo, P., and Maenhaut, W.: Trace elements in tropical African Savanna biomass burning aerosols, J. Atmos. Chem., 22, 19–39, 1995.
Gokhale, S., Kohajda, T., and Schlink, U.: Source apportionment of human personal exposure to volatile organic compounds in homes, offices and outdoors by chemical mass balance and genetic algorithm receptor models, Sci. Total Environ., 407, 122–138, 2008.
Grandesso, E., Gullett, B., Touati, A., and Tabor, D.: Effect of moisture, charge size, and chlorine concentration on PCDD/F emissions from simulated open burning of forest biomass, Environ. Sci. Technol., 45, 3887–3894, 2011.
Gu, Z. P., Feng, J. L., Han, W. L., Wu, M. H., Fu, J. M., and Sheng, G. Y.: Characteristics of organic matter in PM2.5 from an e-waste dismantling area in Taizhou, China, Chemosphere, 80, 800–806, 2010.
Hao, Y. C., Guo, Z. G., Yang, Z. S., Fang, M., and Feng, J. L.: Seasonal variations and sources of various elements in the atmospheric aerosols in Qingdao, China, Atmos. Res., 85, 27–37, 2007.
Ho, S. S. H. and Yu, J. Z.: In-injection port thermal desorption and subsequent gas chromatography-mass spectrometric analysis of polycyclic aromatic hydrocarbons and n-alkanes in atmospheric aerosol samples, J. Chromatogr. A, 1059, 121–129, 2004.
Ho, S. S. H., Yu, J. Z., Chow, J. C., Zielinska, B., Watson, J. G., Sit, E. H., and Schauer, J. J.: Evaluation of an in-injection port thermal desorption-gas chromatography/mass spectrometry method for analysis of non-polar organic compounds in ambient aerosol samples, J. Chromatogr. A, 1200, 217–227, 2008.
Ho, S. S. H., Chow, J. C., Watson, J. G., Ng, L. P. T., Kwok, Y., and Ho, K. F.: Precautions for in-injection port thermal desorption-gas chromatography/mass spectrometry (TD-GC/MS) as applied to aerosol filter samples, Atmos. Environ., 45, 1491–1496, 2011.
Hu, X., Zhang, Y., Ding, Z. H., Wang, T. J., Lian, H. Z., Sun, Y. Y., and Wu, J. C.: Bioaccessibility and health risk of arsenic and heavy metals (Cd, Co, Cr, Cu, Ni, Pb, Zn and Mn) in TSP and PM2.5 in Nanjing, China, Atmos. Environ., 57, 146–152, 2012.
Huang, R.-J., Zhang, Y., Bozzetti, C., Ho, K., Cao, J., Han, Y., Daellenbach, K., Slowik, J., Platt, S., Canonaco, F., Zotter, P., Wolf, R., Pieber, S., Bruns, E., Crippa, M., Ciarelli, G., Piazzalunga, A., Schwikowski, M., Abbaszade, G., Schnelle-Kreis, J., Zimmermann, R., An, Z., Szidat, S., Baltensperger, U., El Haddad, I., and Prévôt, A.: High secondary aerosol contribution to particulate pollution during haze events in China, Nature, 514, 218–222, 2014.
IARC: Di (2-ethylhexyl) phthalate, International Agency for Research on Cancer (IARC) Monographs on the Evaluation of Carcinogenic Risks to Humans, 29, 269–294, 1982.
IMF: World Economic Outlook Update, A Firming Recovery, available at: https://www.imf.org/en/Publications/WEO/Issues/2017/07/07/world-economic-outlook-update-july-2017, 2017.
Jedrychowski, W. A., Perera, F. P., Majewska, R., Mrozek-Budzyn, D., Mroz, E., Roen, E. L., Sowa, A., and Jacek, R.: Depressed height gain of children associated with intrauterine exposure to polycyclic aromatic hydrocarbons (PAH) and heavy metals: The cohort prospective study, Environ. Res., 136, 141–147, 2015.
Kabata-Pendias, A. and Mukherjee, A. B.: Trace Elements from Soil to Human, Springer-Verlag, Berlin Heidelberg, Germany, 381–393, 2007.
Kang, C. M., Lee, H. S., Kang, B. W., Lee, S. K., and Sunwoo, Y.: Chemical characteristics of acidic gas pollutants and PM2.5 species during hazy episodes in Seoul, South Korea, Atmos. Environ., 38, 4749–4760, 2004.
Keita, S., Liousse, C., Yoboué, V., Dominutti, P., Guinot, B., Assamoi, E.-M., Borbon, A., Haslett, S. L., Bouvier, L., Colomb, A., Coe, H., Akpo, A., Adon, J., Bahino, J., Doumbia, M., Djossou, J., Galy-Lacaux, C., Gardrat, E., Gnamien, S., Léon, J. F., Ossohou, M., N'Datchoh, E. T., and Roblou, L.: Particle and VOC emission factor measurements for anthropogenic sources in West Africa, Atmos. Chem. Phys., 18, 7691–7708, https://doi.org/10.5194/acp-18-7691-2018, 2018.
Kong, S., Ji, Y., Liu, L., Chen, L., Zhao, X., Wang, J., Bai, Z., and Sun, Z.: Spatial and temporal variation of phthalic acid esters (PAEs) in atmospheric PM10 and PM2.5 and the influence of ambient temperature in Tianjin, China, Atmos. Environ., 74, 199–208, 2013.
Lacaux, J. P., Brustet, J. M., Delmas, R., Menaut, J. C., Abbadie, L., Bonsang, B., Cachier, H., Baudet, J., Andreae, M. O., and Helas, G.: Biomass burning in the tropical savannas of Ivory Coast: an overview of the field experiment Fire of Savannas (FOS/DECAFE 91), J. Atmos. Chem., 22, 195–216, 1995.
Laurent, B., Marticorena, B., Bergametti, G., Léon, J. F., and Mahowald, N. M.: Modeling mineral dust emissions from the Sahara Desert using new surface properties and soil database, J. Geophys. Res.-Atmos., 113, D14218, https://doi.org/10.1029/2007JD009484, 2008.
Lawin, H., Agodokpessi, G., Ayelo, P., Kagima, J., Sonoukon, R., Ngahane, B. H. M., Awopeju, O., Vollmer, W. M., Nemery, B., Burney, P., and Fayomi, B.: A cross-sectional study with an improved methodology to assess occupational air pollution exposure and respiratory health in motorcycle taxi driving, Sci. Total Environ., 550, 1–5, 2016.
Léon, J.-F., Derimian, Y., Chiapello, I., Tanré, D., Podvin, T., Chatenet, B., Diallo, A., and Deroo, C.: Aerosol vertical distribution and optical properties over M'Bour (16.96∘ W; 14.39∘ N), Senegal from 2006 to 2008, Atmos. Chem. Phys., 9, 9249–9261, https://doi.org/10.5194/acp-9-9249-2009, 2009.
Li, C. L., Fu, J. M., Sheng G. Y., Bi, X. H., Hao, Y. M., Wang, X. M., and Mai, B. X.: Vertical distribution of PAHs in the indoor and outdoor PM2.5 in Guangzhou, China, Build. Environ., 40, 329–341, 2005.
Li, X., Wang, S., Duan, L., Hao, J., and Nie, Y.: Carbonaceous aerosol emissions from household biofuel combustion in China, Environ. Sci. Technol., 43, 6076–6081, 2009.
Li, H. L., Song, W. W., Zhang, Z. F., Ma, W. L., Gao, C. J., Li, J., Huo, C. Y., Mohammed, M. O. A., Liu, L. Y., Kannan, K., and Li, Y. F.: Phthalates in dormitory and house dust of northern Chinese cities: Occurrence, human exposure, and risk assessment, Sci. Total Environ., 565, 496–502, 2016.
Lima, A. L. C., Farrington, J. W., and Reddy, C. M.: Combustion-derived polycyclic aromatic hydrocarbons in the environment – A review, Environ. Forensics, 6, 109–131, 2005.
Lindesay, J. A., Andreae, M. O., Goldammer, J. G., Harris, G. W., Annegarn, H. J., Garstang, M., Scholes, R. J., and van Wilgen, B. W.: The IGBP/IGAC SAFARI-92 field experiment: background and overview, J. Geophys. Res., 101, 521–530, 1996.
Liousse, C., Guillaume, B., Grégoire, J. M., Mallet, M., Galy, C., Pont, V., Akpo, A., Bedou, M., Castéra, P., Dungall, L., Gardrat, E., Granier, C., Konaré, A., Malavelle, F., Mariscal, A., Mieville, A., Rosset, R., Serça, D., Solmon, F., Tummon, F., Assamoi, E., Yoboué, V., and Van Velthoven, P.: Updated African biomass burning emission inventories in the framework of the AMMA-IDAF program, with an evaluation of combustion aerosols, Atmos. Chem. Phys., 10, 9631–9646, https://doi.org/10.5194/acp-10-9631-2010, 2010.
Liousse, C., Assamoi, E., Criqui, P., Granier, C., and Rosset, R.: African combustion emission explosive growth from 2005 to 2030, Environ. Res. Lett., 9, 156–166, 2014.
Liu, X. T., Zhai, Y. B., Zhu, Y., Liu, Y. N., Chen, H. M., Li, P., Peng, C., Xu, B. B., Li, C. T., and Zeng, G. M.: Mass concentration and health risk assessment of heavy metals in size-segregated airborne particulate matter in Changsha, Sci. Total Environ., 517, 215–221, 2015.
Marticorena, B., Chatenet, B., Rajot, J. L., Traoré, S., Coulibaly, M., Diallo, A., Koné, I., Maman, A., NDiaye, T., and Zakou, A.: Temporal variability of mineral dust concentrations over West Africa: analyses of a pluriannual monitoring from the AMMA Sahelian Dust Transect, Atmos. Chem. Phys., 10, 8899–8915, https://doi.org/10.5194/acp-10-8899-2010, 2010.
Meng, Q. Y., Spector, D., Colome, S., and Turpin, B.: Determinants of indoor and personal exposure to PM2.5 of indoor and outdoor origin during the RIOPA study, Atmos. Environ., 43, 5750–5758, 2009.
Meng, X. Z., Wang, Y., Xiang, N., Chen, L., Liu, Z. G., Wu, B., Dai, X. H., Zhang, Y. H., Xie, Z. Y., and Ebinghaus, R.: Flow of sewage sludge-borne phthalate esters (PAEs) from human release to human intake: implication for risk assessment of sludge applied to soil, Sci. Total Environ., 476, 242–249, 2014.
Nisbet, I. C. T. and Lagoy, P. K.: Toxic equivalency factors (TEFs) for polycyclic aromatic hydrocarbons (PAHs), Regul. Toxicol. Pharm., 16, 290–300, 1992.
Norman, R., Barnes, B., Mathee, A., and Bradshaw, D.: Estimating the burden of disease attributable to indoor air pollution from household use of solid fuels in South Africa in 2000, S. Afr. Med. J., 97, 764–771, 2007.
Ouafo-Leumbe, M.-R., Galy-Lacaux, C., Liousse, C., Pont, V., Akpo, A., Doumbia, T., Gardrat, E., Zouiten, C., Sigha-Nkamdjou, L., and Ekodeck, G. E.: Chemical composition and sources of atmospheric aerosols at Djougou (Benin), Meteorol. Atmos. Phys., 1–19, https://doi.org/10.1007/s00703-017-0538-5, 2017.
Owili, P. O., Lien, W.-H., Muga, M. A., and Lin, T. H.: The associations between types of ambient PM2.5 and under-five and maternal mortality in Africa, Inter. J. Env. Res. Pub. Heal., 14, 359, 1–20, 2017.
Reeves, C. E., Formenti, P., Afif, C., Ancellet, G., Attié, J.-L., Bechara, J., Borbon, A., Cairo, F., Coe, H., Crumeyrolle, S., Fierli, F., Flamant, C., Gomes, L., Hamburger, T., Jambert, C., Law, K. S., Mari, C., Jones, R. L., Matsuki, A., Mead, M. I., Methven, J., Mills, G. P., Minikin, A., Murphy, J. G., Nielsen, J. K., Oram, D. E., Parker, D. J., Richter, A., Schlager, H., Schwarzenboeck, A., and Thouret, V.: Chemical and aerosol characterisation of the troposphere over West Africa during the monsoon period as part of AMMA, Atmos. Chem. Phys., 10, 7575–7601, https://doi.org/10.5194/acp-10-7575-2010, 2010.
Ruellan, S., Cachier, H., Gaudichet, A., Masclet, P., and Lacaux, J. P.: Airborne aerosols over central Africa during the experiment for regional sources and sinks of oxidants (EXPRESSO), J. Geophys. Res.-Atmos., 104, 673–690, 1999.
Sealy, A., Jenkins, G. S., and Walford, S. C.: Seasonal/regional comparisons of rain rates and rain characteristics in West Africa using TRMM observations, J. Geophys. Res.-Atmos., 108, 4306, https://doi.org/10.1029/2002JD002667, 2003.
Seinfeld, J. H. and Pandis, S. N.: Atmospheric chemistry and physics: from air pollution to climate change, John Wiley & Sons, p. 1225, 2006.
Shen, G., Wei, S., Wei, W., Zhang, Y., Min, Y., Wang, B., Wang, R., Li, W., Shen, H., Huang, Y., Yang, Y., Wang, W., Wang, X., Wang, X., and Tao, S.: Emission factors, size distributions, and emission inventories of carbonaceous particulate matter from residential wood combustion in rural China, Environ. Sci. Technol., 46, 4207–4214, 2012.
Shen, G., Xue, M., Wei, S., Chen, Y., Wang, B., Wang, R., Lv, Y., Shen, H., Li, W., Zhang, Y., Huang, Y., Chen, H., Wei, W., Zhao, Q., Li, B., Wu, H. and TAO, S.: The influence of fuel moisture, charge size, burning rate and air ventilation conditions on emissions of PM, OC, EC, Parent PAHs, and their derivatives from residential wood combustion, J. Environ. Sci., 25, 1808–1816, 2013.
Simoneit, B. R.: A Review of biomarker compounds as source indicators and tracers for air pollution, Environ. Sci. Pollut. R., 6, 159–169, 1999.
Škrbic, B. D., Ji, Y. Q., Durisic-Mladenovic, N., and Zhao, J.: Occurence of the phthalate esters in soil and street dust samples from the Novi Sad city area, Serbia, and the influence on the children's and adults' exposure, J. Hazard. Mater., 312, 272–279, 2016.
Sun, Y. Y., Hu, X., Wu, J. C., Lian, H. Z., and Chen, Y. J.: Fractionation and health risks of atmospheric particle-bound As and heavy metals in summer and winter, Sci. Total Environ., 493, 487–494, 2014.
Swap, R. J., Annegarn, H. J., and Otter, L.: Southern African regional science initiative (SAFARI 2000) summary of science plan, S. Afr. J. Sci., 98, 119-124, 2002.
Tang, D. L., Li, T.-Y., Liu, J. J., Zhou, Z. J., Yuan, T., Chen, Y.-H., Rauh, V. A., Xie, J., and Perera, F.: Effects of prenatal exposure to coal-burning pollutants on children's development in China, Environ. Health Persp., 116, 674–679, 2008.
Taylor, S. R. and McLennan, S. M.: The continental crust: Its composition and evolution, Phys. Earth Planet. In., 42, 196–197, 1985.
Tian, J., Ni, H. Y., Cao, J. J., Han, Y. M., Wang, Q. Y., Wang, X. L., Chen, L. W. A., Chow, J. C., Watson, J. G., Wei, C., Sun, J., Zhang, T., and Huang, R. J.: Characteristics of carbonaceous particles from residential coal combustion and agricultural biomass burning in China, Atmos. Pollut. Res., 8, 521–527, 2017.
Titcombe, M. E. and Simcik, M.: Personal and indoor exposure to PM2.5 and polycyclic aromatic hydrocarbons in the southern highlands of Tanzania: a pilot-scale study, Environ. Monit. Assess., 180, 461–476, 2011.
Tobiszewski, M. and Namiesnik, J.: Review: PAH diagnostic ratios for the identification of pollution emission sources, Environ. Pollut., 162, 110–119, 2012.
Turpin, B. J. and Lim, H. J.: Species contributions to PM2.5 mass concentrations: Revisiting common assumptions for estimating organic mass, Aerosol Sci. Tech., 35, 602–610, 2001.
UNEP: available at: https://environmentlive.unep.org/publication/country/CC3B4te20d'Ivoire/unep (last access: 23 April 2018), 2015.
U.S. EPA, Exposure factors handbook, National Center for Environmental Assessment, Washington, DC; EPA/600/R-09/052F, National Technical Information Service, Springfield, VA, p. 1436, 2011.
U.S. EPA, Integrated Science Assessment (ISA) for Lead (Third External Review Draft, Nov. 2012), p. 1762, 2012.
U.S. EPA, Risk Assessment Guidance for Superfund Volume I: Human Health Evaluation Manual (Part E, Supplemental Guidance for Dermal Risk Assessment), Office of Superfund Remediation and Technology Innovation U.S. Environmental Protection Agency Washington, DC, p. 156, 2004.
U.S. EPA, Integrated risk information system, Di(2-ethyhexyl)phthalate (DEHP) (CASRN 117-81-7), http://www.epa.gov/iris/subst/0014.htm (last access: 15 May 2018), 1997.
Val, S., Liousse, C., Doumbia, E. H. T., Galy-Lacaux, C., Cachier, H., Marchand, N., Badel, A., Gardrat, E., Sylvestre, A., and Baeza-Squiban, A.: Physico-chemical characterization of African urban aerosols (Bamako in Mali and Dakar in Senegal) and their toxic effects in human bronchial epithelial cells: description of a worrying situation, Part. Fibre Toxicol., 10, 10, https://doi.org/10.1186/1743-8977-10-10, 2013.
Wang, G. H., Kawamura, K., Lee, S. C., Ho, K. F., and Cao, J. J.: Molecular, seasonal, and spatial distributions of organic aerosols from fourteen Chinese cities, Environ. Sci. Technol., 40, 4619–4625, 2006.
Wang, G. H., Kawamura, K., Xie, M., Hu, S., Cao, J., An, Z., Waston, J. G., and Chow, J. C.: Organic Molecular Compositions and Size Distributions of Chinese Summer and Autumn Aerosols from Nanjing: Characteristic Haze Event Caused by Wheat Straw Burning, Environ. Sci. Technol., 43, 6493–6499, 2009.
Wang, J. Z., Guinot, B., Dong, Z. B., Li, X. P., Xu, H. M., Xiao, S., Ho, S. S. H., Liu, S. X., and Cao, J. J.: PM2.5-bound polycyclic aromatic hydrocarbons (PAHs), oxygenated-PAHs and phthalate esters (PAEs) inside and outside middle school classrooms in Xi'an, China: Concentration, characteristics and health risk assessment, Aerosol Air Qual. Res., 17, 1811–1824, 2017.
Wang, Y., Cheng, K., Wu, W. D., Tian, H. Z., Yi, P., Zhi, G. R., Fan, J., and Liu, S. H.: Atmospheric emissions of typical toxic heavy metals from open burning of municipal solid waste in China, Atmos. Environ., 152, 6–15, 2017.
Wang, Y., Zhang, Q. Q., He, K., Zhang, Q., and Chai, L.: Sulfate-nitrate-ammonium aerosols over China: Response to 2000–2015 emission changes of sulfur dioxide, nitrogen oxides, and ammonia, Atmos. Chem. Phys., 13, 2635–2652, 2013.
Watson, J. G., Chow, J. C., and Houck, J. E.: PM2.5 chemical source profiles for vehicle exhaust, vegetative burning, geological material, and coal burning in northwestern Colorado during 1995, Chemosphere, 43, 1141–1151, 2001.
WHO: Environmental Health Criteria 202: Selected non-heterocyclic polycyclic aromatic hydrocarbons, World Health Organization, Geneva, 1998.
Wu, F., Zhang, D. Z., Cao, J. J., Xu, H. M., and An, Z.S: Soil-derived sulfate in atmospheric dust particles at Taklimakan desert, Geophy. Res. Lett., 39, L24803, https://doi.org/10.1029/2012GL054406, 2012.
Xu, H. M., Cao, J. J., Ho, K. F., Ding, H., Han, Y. M., Wang, G. H., Chow, J. C., Watson, J. G., Khol, S. D., Qiang, J., and Li, W. T.: Lead concentrations in fine particulate matter after the phasing out of leaded gasoline in Xi'an, China, Atmos. Environ., 46, 217–224, 2012.
Xu, H. M., Tao, J., Ho, S. S. H., Ho, K. F., Cao, J. J., Li, N., Chow, J. C., Wang, G. H., Han, Y. M., Zhang, R. J., Watson, J. G., and Zhang, J. Q.: Characteristics of fine particulate non-polar organic compounds in Guangzhou during the 16th Asian Games: effectiveness of air pollution controls, Atmos. Environ., 76, 94–101, 2013.
Xu, H. M., Guinot, B., Niu, X. Y., Cao, J. J., Ho, K. F., Zhao, Z. H., Ho, S. S. H., and Liu, S. X.: Concentrations, particle-size distributions, and indoor/outdoor differences of polycyclic aromatic hydrocarbons (PAHs) in a middle school classroom in Xi'an, China, Environ. Geochem. Hlth., 37, 861–873, 2015.
Xu, H. M., Ho, S. S. H., Gao, M. L., Cao, J. J., Guinot, B., Ho, K. F., Long, X., Wang, J. Z., Shen, Z. X., Liu, S. X., Zheng, C. L., and Zhang Q.: Microscale spatial distribution and health assessment of PM2.5-bound polycyclic aromatic hydrocarbons (PAHs) at nine communities in Xi'an, China, Environ. Pollut., 218, 1065–1073, 2016a.
Xu, H. M., Cao, J. J., Chow, J. C., Huang, R.-J., Shen, Z. X., Chen, L. W. A., Ho, K. F., and Watson, J. G.: Inter-annual variability of wintertime PM2.5 chemical composition in Xi'an, China: evidences of changing source emissions, Sci. Total Environ., 545, 546–555, https://doi.org/10.1016/j.scitotenv.2015.12.070, 2016b.
Xu, H. M., Sonke, J. E., Guinot, B., Fu, X. W., Sun, R. Y., Lanzanova, A., Candaudap, F., Shen, Z. X., and Cao, J. J.: Seasonal and annual variations in atmospheric Hg and Pb isotopes in Xi'an, China, Environ. Sci. Technol., 51, 3759–3766, 2017.
Xu, H. M., Guinot, B., Cao, J. J., Li, Y. Q., Niu, X. Y., Ho, K. F., Shen, Z. X., Liu, S. X., Zhang, T., Lei, Y. L., Zhang, Q., Sun, J., and Gao, J. J.: Source, health risk and composition impact of outdoor very fine particles (VFPs) to school indoor environment in Xi'an, Northwestern China, Sci. Total Environ., 612, 238–246, 2018a.
Xu, H. M., Li, Y. Q., Guinot, B., Wang, J. H., He, K. L., Ho, K. F., Cao, J. J., Shen, Z. X., Sun, J., Lei, Y. L., Gong, X. S., and Zhang, T.: Personal exposure of PM2.5 emitted from solid fuels combustion for household heating and cooking in rural Guanzhong Plain, northwestern China, Atmos. Environ., 185, 196–206, 2018b.
Xu, H. M., Guinot, B., Ho, S. S. H., Li, Y. Q., Cao, J. J., Shen, Z. Z., Niu, X. Y., Zhao, Z. H., Liu, S. X., Lei, Y. L., Zhang, Q., and Sun, J.: Evaluation on exposures to particulate matter in a junior secondary school: First comprehensive study on health risks and effective inflammatory responses in Northwestern China, Environ. Geochem. Hlth., 40, 849–863, 2018c.
Yang, F. X., Jin, S. W., Xu, Y., and Lu, Y. N.: Comparisons of IL-8, ROS and p53 responses in human lung epithelial cells exposed to two extracts of PM2.5 collected from an e-waste recycling area, China, Environ. Res. Lett., 6, 1–6, 2011.
Yassaa, N., Meklati, B. Y., Cecinato, A., and Marino, F.: Particulate n-alkanes, nalkanoic acids and polycyclic aromatic hydrocarbons in the atmosphere of Algiers City area, Atmos. Environ., 35, 1843–1851, 2001.
Yunker, M. B., Macdonald, R. W., Vingarzan, R., Mitchell, R. H., Goyette, D., and Sylvestre, S.: PAHs in the Fraser River basin: a critical appraisal of PAH ratios as indicators of PAH source and composition, Org. Geochem., 33, 489–515, 2002.
Zhang, R., Jing, J., Tao, J., Hsu, S.-C., Wang, G., Cao, J., Lee, C. S. L., Zhu, L., Chen, Z., Zhao, Y., and Shen, Z.: Chemical characterization and source apportionment of PM2.5 in Beijing: seasonal perspective, Atmos. Chem. Phys., 13, 7053–7074, https://doi.org/10.5194/acp-13-7053-2013, 2013.
Zhang, L. B., Wang, F. M., Ji, Y. Q., Jiao, J., Zou, D. K., Liu, L. L., Shan, C. Y., Bai, Z. P., and Sun, Z. R.: Phthalate esters (PAEs) in indoor PM10/PM2.5 and human exposure to PAEs via inhalation of indoor air in Tianjin, China, Atmos. Environ., 85, 139–146, 2014.
Zhang, T., Cao, J. J., Chow, J. C., Shen, Z. X., Ho, K. F., Ho, S. S. H., Liu, S. X., Han, Y. M., Watson, J. G., Wang, G. H., and Huang, R. J.: Characterization and seasonal variations of levoglucosan in fine particulate matter in Xi'an. China, J. Air Waste Manag., 64, 1317–1327, 2014.
Zhao, W. X. and Hopke, P. K.: Source investigation for ambient PM2.5 in Indianapolis, IN, Aerosol Sci. Tech., 40, 898–909, 2006.
Zhou, P., Guo, J., Zhou, X. Y., Zhang, W., Liu, L. L., Liu, Y. C., and Lin, K. F.: PM2.5, PM10 and health risk assessment of heavy metals in a typical printed circuit noards manufacturing workshop, J. Environ. Sci., 26, 2018–2026, 2014.
- Abstract
- Introduction
- Materials and methods
- Results and discussion
- Organic species fingerprints of personal exposure to PM2.5
- Health risk assessment of personal exposure to PM2.5
- Conclusions
- Data availability
- Author contributions
- Competing interests
- Special issue statement
- Acknowledgements
- Financial support
- Review statement
- References
- Supplement
- Abstract
- Introduction
- Materials and methods
- Results and discussion
- Organic species fingerprints of personal exposure to PM2.5
- Health risk assessment of personal exposure to PM2.5
- Conclusions
- Data availability
- Author contributions
- Competing interests
- Special issue statement
- Acknowledgements
- Financial support
- Review statement
- References
- Supplement