the Creative Commons Attribution 4.0 License.
the Creative Commons Attribution 4.0 License.
Large contribution of fossil fuel derived secondary organic carbon to water soluble organic aerosols in winter haze in China
Yan-Lin Zhang
Imad El-Haddad
Ru-Jin Huang
Kin-Fai Ho
Jun-Ji Cao
Yongming Han
Peter Zotter
Carlo Bozzetti
Kaspar R. Daellenbach
Jay G. Slowik
Gary Salazar
André S. H. Prévôt
Water-soluble organic carbon (WSOC) is a large fraction of organic aerosols (OA) globally and has significant impacts on climate and human health. The sources of WSOC remain very uncertain in polluted regions. Here we present a quantitative source apportionment of WSOC, isolated from aerosols in China using radiocarbon (14C) and offline high-resolution time-of-flight aerosol mass spectrometer measurements. Fossil emissions on average accounted for 32–47 % of WSOC. Secondary organic carbon (SOC) dominated both the non-fossil and fossil derived WSOC, highlighting the importance of secondary formation to WSOC in severe winter haze episodes. Contributions from fossil emissions to SOC were 61 ± 4 and 50 ± 9 % in Shanghai and Beijing, respectively, significantly larger than those in Guangzhou (36 ± 9 %) and Xi'an (26 ± 9 %). The most important primary sources were biomass burning emissions, contributing 17–26 % of WSOC. The remaining primary sources such as coal combustion, cooking and traffic were generally very small but not negligible contributors, as coal combustion contribution could exceed 10 %. Taken together with earlier 14C source apportionment studies in urban, rural, semi-urban and background regions in Asia, Europe and the USA, we demonstrated a dominant contribution of non-fossil emissions (i.e., 75 ± 11 %) to WSOC aerosols in the Northern Hemisphere; however, the fossil fraction is substantially larger in aerosols from East Asia and the eastern Asian pollution outflow, especially during winter, due to increasing coal combustion. Inclusion of our findings can improve a modelling of effects of WSOC aerosols on climate, atmospheric chemistry and public health.
Water-soluble organic carbon (WSOC) is a large fraction of atmospheric organic aerosols (OA), which contributes approximately 10 to 80 % of the total mass of organic carbon (OC) in aerosols from urban, rural and remote sites (Zappoli et al., 1999; Weber et al., 2007; Ruellan and Cachier, 2001; Wozniak et al., 2012; Mayol-Bracero et al., 2002). Only 10 to 20 % of total mass of WSOC has been resolved at a molecular level, and it consists of a large variety of chemical species such as mono- and di-carboxylic acids, carbohydrate derivatives, alcohols, aliphatic and aromatic acids and amino acids (Fu et al., 2015; Noziere et al., 2015). Recent studies suggest that the water-soluble fraction of humic like substances (HULIS) is a major component of WSOC, which exhibits light-absorbing properties (Limbeck et al., 2005; Andreae and Gelencser, 2006; Laskin et al., 2015). Therefore, WSOC has significant influences on the Earth's climate either directly by scattering and absorbing radiation or indirectly by altering the hygroscopic properties of aerosols and increasing cloud condensation nuclei (CCN) activity (Asa-Awuku et al., 2011; Cheng et al., 2011; Hecobian et al., 2010).
WSOC can be directly emitted as primary particles mainly from biomass burning emissions or produced from secondary organic aerosol (SOA) formation (Sannigrahi et al., 2006; Kondo et al., 2007; Weber et al., 2007; Bozzetti et al., 2017a, b). Ambient studies provide evidence that SOA formation through the oxidation of volatile organic compounds (VOCs) and gas to particle conversion processes may be a prevalent source of WSOC (Kondo et al., 2007; Weber et al., 2007; Miyazaki et al., 2006; Hecobian et al., 2010). WSOC is therefore thought to be a good proxy for secondary organic carbon (SOC) in the absence of biomass burning (Weber et al., 2007). By contrast, water insoluble OC (WIOC) is thought to be mainly from primary origins with a substantial contribution from fossil fuel emissions (Miyazaki et al., 2006; Zhang et al., 2014a).
Due to a large variety of sources and unresolved formation processes of WSOC, their relative fossil and non-fossil contributions are still poorly constrained. Radiocarbon (14C) analysis of sub-fractions of organic aerosols such as OC, WIOC and WSOC enable an unambiguous, precise and quantitative determination of their fossil and non-fossil sources (Zhang et al., 2012, 2014a, b; Zong et al., 2016; Cao et al., 2017). Meanwhile, the application of aerosol mass spectrometer measurement, positive matrix factorization and multi-linear engine 2 (ME-2) can quantitatively classify organic aerosols into two major types such as hydrocarbon-like OA (HOA) from primary fossil fuel combustion and oxygenated organic aerosol (OOA) from secondary origin (Zhang et al., 2007; Jimenez et al., 2009). Field campaigns with the aerosol mass spectrometer (AMS) have revealed a predominance of OOA in various atmospheric environments, although their sources remain poorly characterized (Zhang et al., 2007; Jimenez et al., 2009). Previous studies found OOA is strongly correlated with WSOC from urban aerosols in Tokyo, Japan, the Pearl River Delta (PRD) in South China and Helsinki, Finland, indicating similar chemical characteristics, sources and formation processes of OOA and WSOC (Kondo et al., 2007; Xiao et al., 2011; Timonen et al., 2013). Similarly, HOA is mostly water insoluble and the major portion of water insoluble OC (WIOC) can be assigned as HOA (Kondo et al., 2007; Daellenbach et al., 2016). Therefore, 14C measurement of WIOC and WSOC aerosols may provide new insights into sources and formation processes of primary and secondary OA, respectively, which also will elucidate the origin of HOA and OOA as measured by AMS (Zotter et al., 2014b; Zhang et al., 2017).
In this paper, we apply a newly developed method to measure 14C in WSOC of PM2.5 (particulate matter with an aerodynamic diameter of smaller than 2.5 µm) samples collected at four Chinese megacities during an extremely severe haze episode in winter 2013 (Y. L. Zhang et al., 2015; Huang et al., 2014). In conjunction with our previous dataset from the same campaign, we quantify fossil and non-fossil emissions from primary and secondary sources of WSOC and WIOC. The dataset is also complemented by previous 14C-based source apportionment studies conducted in urban, rural and remote regions in the Northern Hemisphere to gain an overall picture of the sources of WSOC aerosols.
2.1 Sampling
During January 2013, extremely high concentrations of 24 h PM2.5 (i.e. often > 100 µg m−3) were identified in several large cities in eastern China (Huang et al., 2014; Y. L. Zhang et al., 2015). To investigate sources and formation mechanisms of the haze particles, an intensive field campaign was carried out in four large cities, Beijing, Xi'an, Shanghai and Guangzhou, which are representative cities of the Beijing–Tianjin–Hebei region, central-northwestern region, Yangtze Delta Region, and Pearl River Delta Region, respectively. The sampling procedures have been previously described in detail elsewhere (Y. L. Zhang et al., 2015). Briefly, PM2.5 samples were collected on pre-baked (450 ∘C for 6 h) quartz filters using high-volume samplers for 24 h at a flow rate of ∼ 1.05 m3 min−1 from 5 to 25 January 2013. The sampling sites in each city were located at campuses of universities or at research institutes, at least 100 m away from major emission sources (e.g., roadways, industry and domestic sources). One field blank sample for each site was collected and analyzed. The results reported here were corrected for these field blanks (Zotter et al., 2014a; Cao et al., 2013). All samples were stored at −20 ∘C before analysis. The PM2.5 mass was gravimetrically measured with an analytical microbalance before and after sampling with the same conditions (∼ 12 h)
2.2 OC and EC mass determinations
A 1.0 cm2 filter punches were used for OC and EC mass determination with a OC ∕ EC analyzer (Model4L) using the EUSAAR_2 protocol (Cavalli et al., 2010). The replicate analysis (n = 6) showed analytical precision with relative standard deviations smaller than 5, 10, and 5 % for OC, EC and TC, respectively. The field blank of OC was on average 2.0 ± 1.0 µg cm−2 (equivalent to ∼ 0.5µg m−3), which was used for blank correction for OC. EC data were not corrected for field blank, because such a blank was not detectable.
2.3 Offline-AMS measurement and PMF source apportionment
The water-soluble extracts from the same samples were analyzed by a high-resolution time of flight aerosol mass spectrometer (HR-ToF-AMS) and the resulting mass spectra were used as an inputs for positive matrix factorization (PMF) for the source apportionment of the WSOC, OC and PM2.5. The methodology applied and the AMS-PMF results obtained are detailed in Huang et al. (2014) and will be only briefly described in the following. Here, only data relative to WSOC are used.
Filter punches (the equivalent of ∼ 4 cm2) were sonicated in 10 mL ultrapure water (18.2 MΩ cm at 25 ∘C, TOC < 3 ppb) for 20 min at 30 ∘C. The water extracts were aerosolized and the resulting particles were dried with a silica gel diffusion dryer before analysis by the HR-ToF-AMS. For each measurement ten mass spectra were recorded (AMS V-mode, m∕z 12–500), with a collection time for each spectrum of 1 min.
Online AMS measurements provide quantitative mass spectra of submicron non-refractory aerosol species, including organic aerosol and ammonium nitrate and sulfate. However, the offline AMS measurements described herein cannot be directly related to ambient concentrations due to uncertainties in nebulization and AMS lens cut-off. Here, we have scaled the organic aerosol mass spectra to water soluble organic aerosol concentrations (WSOM), obtained as WSOC times OM ∕ OC ratios. The latter were determined by the high-resolution analysis of the organic aerosol mass spectra, acquired by the AMS.
The quantitative WSOM mass spectra are used together with other aerosol species (listed below), collectively referred to as “species” hereafter, as PMF inputs. PMF solves the bilinear matrix equation as follows:
by following a weighted least squares approach. In the equation, i represent the time index, j a species and k the factor number. Xij is the input matrix, Gi,k is the matrix of the factor time series, Fk,j is the matrix of the factor profiles and Ei,j the model residual matrix. PMF determines Gi,k and Fk,j such that the ratio of the Frobenius norm of Ei,j over the uncertainty matrix, si,j, used as model input is minimized.
The species considered as inputs include the quantitative WSOM mass spectra, organic markers (3 anhydrous sugars, 4 lignin breakdown products, 2 resin acids, 4 hopanes, 19 polycyclic aromatic hydrocarbons and their oxygenated derivatives), EC, major ions (Cl−, NO, SO, oxalate, methylsulfonic acid, Na+, K+, Mg2+, Ca2+, and NH) and residual PM. The latter is the difference between total PM2.5 mass and the measured species. It represents our best estimate of the particulate chemical species not measured here, most likely dominated by crustal material.
The Source Finder toolkit (SoFi v.4.9) (Canonaco et al., 2013) from the IGOR Pro software package (Wavemetrics, Inc., Portland, OR, USA) was used to run the PMF algorithm. The PMF was solved by the Multilinear Engine 2 (ME-2, Paatero, 1999), which allows the constraining of the Fk,j elements to vary within a certain range defined by the scalar α (0 ≤ α ≤ 1), such that the modelled equals the following:
The elements that were constrained in Fk,j matrix can be found in Huang et al. (2014). The factors extracted by ME-2 were interpreted to be related to primary emissions from traffic (TR), biomass burning (BB), coal burning (CC), cooking emissions (CI) and dust as well as from two secondary aerosol fractions. The contributions of the water soluble organic aerosol related to these different factors were determined by the multiplying their relative abundance in the factor profiles by the respective factor time series. The factors WSOM time series were then divided by the respective OM ∕ OCk calculated from the high-resolution analysis of the factor mass spectral profile to obtain the WSOCk time series related to each of the factors. The average OM ∕ OCk are: 1.25, 1.39, 1.49, 1.55, 2.25 and 2.4 for TR, CI, BB, CB, SOA and dust, respectively. In the following analysis, the mass of WSOCk related to coal burning and traffic were assigned to the fossil WSOC fraction, while the mass of WSOCk related to biomass burning and cooking emissions were assigned to the non-fossil WSOC fraction (see Sect. 2.5). Meanwhile, the remaining WSOC fractions are assigned to the secondary factors, which can be from both fossil and non-fossil origins. These were considered collectively and compared to the unassigned fossil and non-fossil WSOC, to retrieve the origins of this remaining fraction (see Sect. 2.5).
2.4 14C measurement of WSOC
14C content of micro-scale WSOC aerosol samples was measured with a newly developed method (Zhang et al., 2014c). Briefly, a 16 mm-diameter punch of each filter was extracted using 10 mL ultrapure water with low TOC impurity (less than 5 ppb). The water extracts were recovered in the 20 mL PFA vials and were then pre-frozen at −20 ∘C more than 5 h before completely dryness in a freeze dryer (Alpha 2-4 LSC, Christ, Germany) for about 24 to 36 h. The residue was re-dissolved in 50 µL of ultrapure water three times and transferred into 200 µL tin capsules (Elementar, Germany). The concentrated samples were heated in the oven at 55–60 ∘C until completely dry before the 14C measurements were taken.
WSOC extracts in tin capsules were then converted to CO2 by the oxidation of the carbon-containing samples using an Elemental Analyzer (EA, Model Vario Micro, Elementar, Germany) as a combustion unit (up to 1050 ∘C). The resulting CO2 was introduced continuously by a versatile gas inlet system into a gas ion source of the accelerator mass spectrometer MICADAS where 14C of CO2 was finally measured (Wacker et al., 2013; Salazar et al., 2015). The 14C content of OC and EC was measured in our previous study (Y. L. Zhang et al., 2015). 14C results were expressed as fraction of modern (fM), i.e., the fraction of the measured 14C ∕ 12C ratio related to the 14C ∕ 12C ratio of the reference year 1950 (Stuiver, 1977). To correct excess 14C from nuclear bomb tests in the 1950s and 1960s, fM values were converted to the fraction of non-fossil (fNF) (Zotter et al., 2014a; Zhang et al., 2012) as follows:
fM,ref is a reference value of fM for non-fossil carbon sources including biogenic and biomass burning emissions, which were estimated as 1.08 ± 0.05 (i.e., fM,ref = (0.5 × 1.10 + 0.5 × 1.05) (see details in Zhang et al., 2012) for WSOC samples collected in 2013 according to the contemporary atmospheric CO2 fM (Levin et al., 2010) and a tree growth model (Mohn et al., 2008).
2.5 AMS2-based source apportionment of WSOC
To better understand the origin of WSOC observed at these sites, WSOC sources were apportioned into several major sources by a combination of 14C and PMF source apportionments (see Fig. 1). Here, two “AMS” (i.e., accelerator mass spectrometer and aerosol mass spectrometer), such a combined approach was named “AMS2-based source apportionment”.
WSOC concentration from non-fossil (WSOCNF) and fossil (WSOCF) sources were calculated as follows:
The mass concentration of WSOC was derived from the subtraction of TC mass measured from a water-extracted filter from that measured with an un-treated filter (Zhang et al., 2012):
Based on mass balance, WIOC concentrations from non-fossil (WIOCNF) and fossil (WIOCF) sources were calculated as follows:
where OC concentrations from non-fossil (OCNF) and fossil (OCF) sources were obtained by mass and 14C measurement of the OC fraction, which were reported previously (Y. L. Zhang et al., 2015).
The non-fossil and fossil fuel derived WSOC can be apportioned into primary and secondary OC:
WSOCPOC,NF can be sub-divided into the following three major primary emissions including cooking emission (WSOCCI) and biomass burning (WSOCBB).
Similarly, WSOCPOC,F can be sub-divided into the following two major primary emissions including traffic (WSOCTR) and coal combustion (WSOCCB).
where primary fractions such as WSOCCI, WSOCBB, WSOCTR and WSOCCB are previously estimated by the off line AMS–PMF approach (Huang et al., 2014; Daellenbach et al., 2016; Bozzetti et al., 2017a, b).
An uncertainty propagation scheme using a Latin-hypercube sampling (LHS) model was implemented to properly estimate overall uncertainties, including measurement uncertainties of the mass determinations of carbon species (i.e., OC, EC, TC, WSOC, WIOC) and 14C measurement, blank corrections from field blanks, and estimation of fM,ref (Y. L. Zhang et al., 2015).
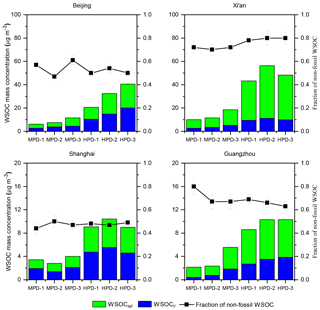
Figure 3Mass concentrations (µg m3) of WSOC from non-fossil and fossil fuel sources (WSOCNF and WSOCF, respectively) as well as non-fossil fractions of the WSOC aerosols from Beijing, Xi'an, Shanghai and Guangzhou during moderately polluted days (MPD) and heavily polluted days (HPD). Note the different scaling for different cities.
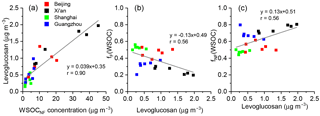
Figure 4Relationships of non-fossil derived WSOC (WSOCNF) and levoglucosan (a), levoglucosan and the fraction of fossil in WSOC (fF(WSOC)) (b) and levoglucosan and the fraction of non-fossil in WSOC (fNF(WSOC)) (c).
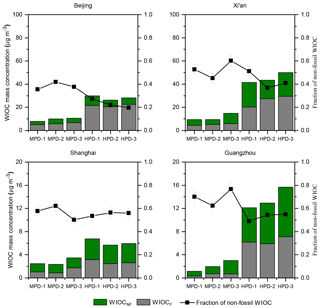
Figure 5Mass concentrations (µg m−3) of WIOC from non-fossil and fossil fuel sources (WIOCNF and WIOCF, respectively) as well as non-fossil fractions in the WIOC aerosols from Beijing, Xi'an, Shanghai and Guangzhou during moderately polluted days (MPD) and heavily polluted days (HPD). Note the different scaling for different cities.
3.1 Overall results
During the haze periods of January 2013, the highest daily average PM2.5 concentrations were found in Xi'an (345 µg m−3) followed by Beijing (158 µg m−3), Shanghai (90 µg m−3) and Guangzhou (68 µg m−3). These levels were much higher than the China's national ambient air quality standards (i.e., 35 µg m−3). Indeed, several studies have already reported the chemical composition, source and formation mechanism of PM2.5 in many large cities during the haze events of January 2013 in eastern China. For examples, Huang et al. (2014) revealed that the secondary aerosol formation contributed to 44–71 % of OA in Beijing, Xi'an, Shanghai, and Guangzhou during this extremely haze event in China (Huang et al., 2014). By 14C-based source appointment conducted in the same campaign, Zhang et al. (2015) have reported that carbonaceous aerosol pollution was driven to a large (often dominant) extent by SOA formation from both fossil and biomass-burning sources (Y. L. Zhang et al., 2015). For all four cities, the 24 h average levels of WSOC were significantly correlated with the levels of PM2.5 and OC (R=0.99, p < 0.01, Fig. 2), suggesting that WSOC and OA may have similar sources and formation processes and thus have important implications for OC loadings and associated environmental and health effects. However, the sources of WSOC remain poorly constrained. In this study, we measured the 14C content of WSOC aerosols in six samples (three with the highest, three with average PM mass) for each city to report on heavily and moderately polluted days (HPD and MPD, respectively) (Y. L. Zhang et al., 2015). The 14C contents of OC and EC of the same samples were reported previously (Y. L. Zhang et al., 2015).
Table 1Compilation of literature values of relative fossil fuel contributions (fossil %) to the WSOC aerosols in East/South Asia, USA and Europe.
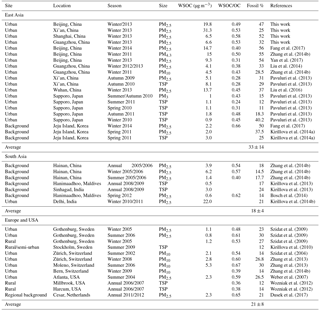
WSOC on average accounted for 53 ± 8.0 % (ranging from 40–65 %) of OC including all samples from the four sites, which was consistent with previous estimates. Based on these measurements, the concentrations of WSOC from non-fossil sources (WSOCNF) spanned from 1.41 to 45.3µg m−3 with a mean of 10.6 ± 12.1µg m−3, whereas the corresponding range for WSOC from fossil fuel emissions (WSOCF) was 0.44 to 20.1µg m−3 with a mean of 5.3 ± 4.9µg m−3 (Fig. 3). Similar to PM2.5 levels, the highest concentrations of WSOCNF and WSOCF were observed in northern China in Xi'an and Beijing (Xi'an > Beijing), followed by the two southern sites of Shanghai and Guangzhou. Non-fossil contributions (mean ± standard deviation) to total WSOC were 53 ± 5, 75 ± 4, 48 ± 2 and 68 ± 6 % in Beijing, Xi'an, Shanghai, and Guangzhou, respectively. Thus, fossil contributions were notably higher in Beijing and Shanghai than in Xi'an and Guangzhou. Such a trend was also observed for OC (Y. L. Zhang et al., 2015), suggesting relatively high contribution from fossil fuel emissions to OC and WSOC due to large coal usage. Despite these fossil emissions, non-fossil sources were important or even dominant contributors for all the studied sites, which may be associated with primary and secondary OA from regional and local biomass burning emissions. As shown in Fig. 4, non-fossil WSOC was significantly correlated with levoglucosan, indicating that a large fraction of non-fossil WSOC was indeed from biomass burning emissions. In addition, no significant (or only a negative) correlation (Fig. 4) was found between levoglucosan and fraction of fossil to WSOC, suggesting that fossil fuel source is very unlikely to be a major or important contributor of levoglucosan even in the regions (e.g., Xi'an and Beijing in this study) where coal combustion is important during the cold period (Y.-L. Zhang et al., 2015). It should also be noted that formation of SOA derived from biogenic VOCs may also have contributed to WSOCNF in Guangzhou, where temperatures during the sampling period were significantly higher (i.e., 5–18 ∘C) than those in other cities (i.e., −12 to +9 ∘C) (Bozzetti et al., 2017b). Although both fossil and non-fossil WSOC concentrations were dramatically enhanced during HPD compared to those during MPD, their relative contributions did not change significantly in Beijing and Shanghai, whereas a small increasing and decreasing trend in the non-fossil fraction was found in Xi'an and Guangzhou, respectively (Fig. 3). This suggests that the source pattern of WSOC in Beijing and Shanghai remained similar between HPD and MPD, but the increase in the WSOC concentrations was rather enhanced by additional fossil fuel and biogenic(or biomass) burning emissions in Guangzhou and Xi'an, respectively. It should be noted that the meteorological conditions played significant roles in the haze formation in eastern China during winter 2013, and has already been well documented (R. Zhang et al., 2014). However, the details sources of WSOC and WIOC were still unclear.
3.2 WSOC versus WIOC
To compare sources of WSOC and WIOC aerosols, the mass concentrations and 14C contents of WIOC were also derived based on mass balance. The 14C-based source apportionment of WIOC and the relationship between fNF (WSOC) and fNF (WIOC) are presented in Figs. 5 and 6a, respectively. It shows that non-fossil contributions to WSOC were larger than those of WIOC for nearly all samples in Beijing, Xi'an and Guangzhou. On average, the majority (60–70 %) of the fossil OC was water insoluble at these three sites (see Fig. 6b), indicating that fossil-derived OA mostly consisted of hydrophobic components and thus is less water soluble than OA from non-fossil sources. This result is consistent with findings reported elsewhere such as at an urban or rural site in Switzerland (Zhang et al., 2013), a remote site on Hainan Island, southern China (Zhang et al., 2014a) and at two rural sites on the east coast of the United States (Wozniak et al., 2012). Meanwhile, the fossil OC in Shanghai, the dominant fraction of OC, was more water soluble (Fig. 6b), suggesting an enhanced SOA formation from fossil VOCs from vehicle emissions and/or coal burning in this city. As shown in Figure 6b, non-fossil OA was enriched in water-soluble fractions (i.e., 60 ± 8 %) for all cities, associated with the hydrophilic properties of biogenic-derived SOA and biomass-burning derived primary organic aerosol (POA) and SOA, which are composed of a large fraction of polar and highly oxygenated compounds (Mayol-Bracero et al., 2002; Sullivan et al., 2011; Noziere et al., 2015). Thus, non-fossil OC has more water-soluble components than fossil OC does. It should be noted that relative contributions of WSOCNF and WSOCF are similar in Beijing and Shanghai, whereas WSOCNF is much higher than WSOCF in Xi'an and Guangzhou. This suggests larger contribution of non-fossil sources to WSOC aerosols in Xi'an and Guangzhou than those in Beijing and Shanghai.
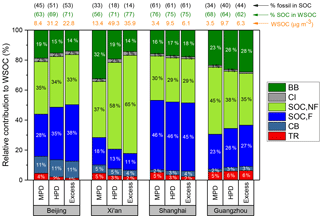
Figure 7Relative contributions (%) to WSOC from biomass burning, as well as secondary organic carbon (SOC) from fossil and non-fossil sources (WSOCSOC,F and WSOCSOC,NF, respectively) in different cities during moderately polluted days (MPD) and heavily polluted days (HPD), as well as their corresponding excess (Excess = HPD-MPD). The numbers above the bars refer to the average WSOC concentrations and the SOC fractions (%) of WSOC.
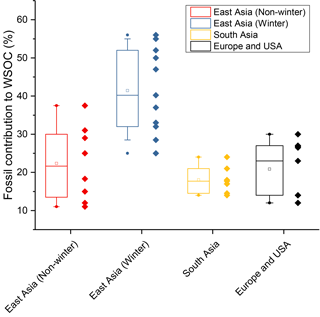
Figure 8Box-plot of the fossil contribution (%) to the WSOC aerosols in East Asia, South Asia, the USA and Europe. The box represents the 25th (lower line), 50th (middle line) and 75th (top line) percentiles; the empty square within the box represent the mean values; the end lines of the vertical bars represent the 10th (below the box) and 90th (above the box) percentiles; the solid dots represent the maximum and minimum values; the solid diamonds represent the individual data (Table 1). The data from East Asia is grouped by winter and non-winter seasons.
3.3 High contribution of secondary formation to WSOC
WSOC was further apportioned into fossil sources such as coal burning (CB), traffic (TR) and SOC (SOC,F) as well as non-fossil sources such as biomass burning (BB), cooking (CI) and SOC (SOC,NF) using a AMS2 based source apportionment (see Sect. 2.5 and Fig. 1). SOC dominated WSOC during both the HPD and MPD with a mean contribution of 67 ± 9 %, highlighting the importance of SOC formation to the WSOC aerosols in winter pollution events. This is consistent with our previous findings for total PM2.5 mass and bulk carbonaceous aerosols (i.e., total carbon, sum of OC and EC) (Huang et al., 2014; Y. L. Zhang et al., 2015). The increase in SOC contribution to WSOC during HPD compared to MPD can be largely due to fossil contribution in Beijing but non-fossil emissions in Xi'an. In Shanghai and Guangzhou, the source pattern of WSOC was not significantly different between MPD and HPD. Fossil contributions to WSOCSOC were 50 ± 9 % in Beijing, 61 ± 4 % in Shanghai, associated with SOA from local and transported fossil fuel derived precursors at these sites (Guo et al., 2014). This contribution drops to 36 ± 9 and 26 ± 9 % in Guangzhou and Xi'an, respectively, due to higher biomass-burning contribution to SOC. Despite of the general importance of fossil SOC, formation of non-fossil WSOCSOC becomes especially relevant during HPD especially in Xi'an (Fig. 7), which may be explained by competing effects in SOC formation from fossil versus non-fossil precursors. It can be hypothesized for extremely polluted episodes that more hydrophilic volatile compounds that were emitted from biomass burning precursors preferentially form SOC compounds via heterogeneous reaction or processing of dust particles, compared to highly hydrophobic precursors from fossil sources, a point that should be subjected to future laboratory and field experiments. The most important primary sources of WSOC were biomass burning emissions, and their contributions were higher in Xi'an (26 ± 7 %) and Guangzhou (25 ± 6 %) than those found in Beijing (17 ± 6 %) and Shanghai (17 ± 5 %). The remaining primary sources such as coal combustion, cooking and traffic were generally very small contributors of WSOC due to lower water solubility, although coal combustion could exceed 10 % in Beijing. It should be noted that WSOC was dominated by SOC formation with a mean contribution of 61± 10 and 72 ± 12 % (average for all four cities) to non-fossil and fossil fuel derived WSOC, respectively.
Our study demonstrates that non-fossil emissions are generally a dominant contributor of WSOC aerosols during extreme haze events in representative major cities of China, which is in agreement with WSOC source information identified in aerosols with different size fractions (e.g., TSP, PM10 and PM2.5) observed in the Northern Hemisphere at urban, rural, semi-urban, and background sites in East/South Asia, Europe and USA (Table 1). The 14C-based source apportionment database shows a mean non-fossil fraction of 73 ± 11 % across all sites. This overwhelming non-fossil contribution to WSOC is consistently observed throughout the year, which is associated with seasonally dependent biomass burning emissions and/or biogenic-derived SOC formation. Our study provides evidence that the presence of oxidized OA, which is to a large extent water soluble, in the Northern Hemisphere (Zhang et al., 2007) is mainly derived from biogenic derived SOA and/or biomass burning sources. The overall importance of non-fossil emissions to the WSOC aerosols results from large contributions of SOC formation from biogenic precursors (e.g., most likely during summer) and relatively high water-solubility of primary biomass burning particles (e.g., most likely during winter) compared to those emitted from fossil fuel emissions such as coal combustion and vehicle exhaust. Despite the importance of non-fossil sources, a significant fossil fraction is also observed in the WSOC aerosols from polluted regions in East Asia and sites influenced by East Asian continental outflow (Table 1, Fig. 8). This fossil contribution is apparently higher than in this region than in the USA and Europe, which is due to large industrial and residential coal usage as well as vehicle emissions. From our observation, the increases in the fossil fractions of WSOC were mostly from SOC formation. Since WSOC has hygroscopic properties, our findings suggest that SOC formation from non-fossil emissions have significant implications on aerosol-induced climate effects. In addition, fossil-derived SOC formation may also become important in polluted regions with large amounts of fossil fuel emissions, such as in China and other emerging countries. Low combustion efficiencies and consequently high emission factors in most of the combustion processes in China may further be responsible for increased concentrations of fossil precursors which may be oxidized to form water-soluble SOA in the atmosphere and contribute substantially to the WSOC aerosols. The enhanced WSOC levels may also originate from aging of fossil POA during the long-range transport of aerosols (Kirillova et al., 2014a). It is also interesting to note that fossil contribution during winter in East Asia is generally higher than those in the rest of the year, although a relatively large fossil fraction could be occasionally found as well. Such seasonal dependence was not observed in other regions, suggesting the importance of fossil contribution to WSOC due to increasing coal combustions during winter in China. This study provides a more detailed source apportionment of WSOC, which could improve modelling of climate and health effects as well as the understanding of atmospheric chemistry of WSOC in the polluted atmosphere such as China and provide a scientific basis for policy decisions on air pollution emissions mitigation.
All data needed to evaluate the conclusions in the paper are present in the paper. Additional data related to this paper may be requested from the authors (Yan-Lin Zhang, zhangyanlin@nuist.edu.cn).
YLZ, SS, RJH, JJC and ASHP designed the study. YLZ and GS perform 14C measurement. YLZ and SS interpreted the 14C data. RJH, IEH, CB and KD performed the offline AMS analysis and interpret the data. YLZ and IEH perform 14C-AMS-PMF source apportionments. YLZ wrote the paper. All authors reviewed and commented on the paper.
The authors declare that they have no conflict of interest.
This article is part of the special issue “Multiphase chemistry of secondary aerosol formation under severe haze”. It does not belong to a conference.
This work was supported by The National Key Research and Development Program
of China (Grant No. 2017YFC0212704), the National Natural Science
Foundation of China (Grant Nos. 41603104 and 91644103) and the Priority
Academic Program Development of Jiangsu Higher Education Institutions (grant
PAPD).Edited by: Daniel Knopf
Reviewed by: two
anonymous referees
Andreae, M. O. and Gelencsér, A.: Black carbon or brown carbon? The nature of light-absorbing carbonaceous aerosols, Atmos. Chem. Phys., 6, 3131–3148, https://doi.org/10.5194/acp-6-3131-2006, 2006.
Asa-Awuku, A., Moore, R. H., Nenes, A., Bahreini, R., Holloway, J. S., Brock, C. A., Middlebrook, A. M., Ryerson, T. B., Jimenez, J. L., DeCarlo, P. F., Hecobian, A., Weber, R. J., Stickel, R., Tanner, D. J., and Huey, L. G.: Airborne cloud condensation nuclei measurements during the 2006 Texas Air Quality Study, J. Geophys. Res., 116, D11201, https://doi.org/10.1029/2010jd014874, 2011.
Bosch, C., Andersson, A., Kirillova, E. N., Budhavant, K., Tiwari, S., Praveen, P. S., Russell, L. M., Beres, N. D., Ramanathan, V., and Gustafsson, O.: Source-diagnostic dual-isotope composition and optical properties of water-soluble organic carbon and elemental carbon in the South Asian outflow intercepted over the Indian Ocean, J. Geophys. Res., 119, 11743–11759, https://doi.org/10.1002/2014JD022127, 2014.
Bozzetti, C., El Haddad, I., Salameh, D., Daellenbach, K. R., Fermo, P., Gonzalez, R., Minguillón, M. C., Iinuma, Y., Poulain, L., Elser, M., Müller, E., Slowik, J. G., Jaffrezo, J. L., Baltensperger, U., Marchand, N., and Prévôt, A. S. H.: Organic aerosol source apportionment by offline-AMS over a full year in Marseille, Atmos. Chem. Phys., 17, 8247–8268, https://doi.org/10.5194/acp-17-8247-2017, 2017a.
Bozzetti, C., Sosedova, Y., Xiao, M., Daellenbach, K. R., Ulevicius, V., Dudoitis, V., Mordas, G., Byčenkienė, S., Plauškaitė, K., Vlachou, A., Golly, B., Chazeau, B., Besombes, J. L., Baltensperger, U., Jaffrezo, J. L., Slowik, J. G., El Haddad, I., and Prévôt, A. S. H.: Argon offline-AMS source apportionment of organic aerosol over yearly cycles for an urban, rural, and marine site in northern Europe, Atmos. Chem. Phys., 17, 117–141, https://doi.org/10.5194/acp-17-117-2017, 2017b.
Canonaco, F., Crippa, M., Slowik, J. G., Baltensperger, U., and Prévôt, A. S. H.: SoFi, an IGOR-based interface for the efficient use of the generalized multilinear engine (ME-2) for the source apportionment: ME-2 application to aerosol mass spectrometer data, Atmos. Meas. Tech., 6, 3649–3661, https://doi.org/10.5194/amt-6-3649-2013, 2013.
Cao, F., Zhang, Y.-L., Szidat, S., Zapf, A., Wacker, L., and Schwikowski, M.: Microgram-level radiocarbon determination of carbonaceous particles in firn and ice samples: pretreatment and OC/EC separation, Radiocarbon, 55, 383–390, 2013.
Cao, F., Zhang, Y., Ren, L., Liu, J., Li, J., Zhang, G., Liu, D., Sun, Y., Wang, Z., Shi, Z., and Fu, P.: New insights into the sources and formation of carbonaceous aerosols in China: potential applications of dual-carbon isotopes, Nat. Sci. Rev., 4, 804–806, https://doi.org/10.1093/nsr/nwx097, 2017.
Cavalli, F., Viana, M., Yttri, K. E., Genberg, J., and Putaud, J.-P.: Toward a standardised thermal-optical protocol for measuring atmospheric organic and elemental carbon: the EUSAAR protocol, Atmos. Meas. Tech., 3, 79–89, https://doi.org/10.5194/amt-3-79-2010, 2010.
Cheng, Y., He, K. B., Zheng, M., Duan, F. K., Du, Z. Y., Ma, Y. L., Tan, J. H., Yang, F. M., Liu, J. M., Zhang, X. L., Weber, R. J., Bergin, M. H., and Russell, A. G.: Mass absorption efficiency of elemental carbon and water-soluble organic carbon in Beijing, China, Atmos. Chem. Phys., 11, 11497–11510, https://doi.org/10.5194/acp-11-11497-2011, 2011.
Daellenbach, K. R., Bozzetti, C., Krepelova, A. K., Canonaco, F., Wolf, R., Zotter, P., Fermo, P., Crippa, M., Slowik, J. G., Sosedova, Y., Zhang, Y., Huang, R. J., Poulain, L., Szidat, S., Baltensperger, U., El Haddad, I., and Prevot, A. S. H.: Characterization and source apportionment of organic aerosol using offline aerosol mass spectrometry, Atmos. Meas. Tech., 9, 23–39, https://doi.org/10.5194/amt-9-23-2016, 2016.
Dusek, U., Hitzenberger, R., Kasper-Giebl, A., Kistler, M., Meijer, H. A. J., Szidat, S., Wacker, L., Holzinger, R., and Röckmann, T.: Sources and formation mechanisms of carbonaceous aerosol at a regional background site in the Netherlands: insights from a year-long radiocarbon study, Atmos. Chem. Phys., 17, 3233–3251, https://doi.org/10.5194/acp-17-3233-2017, 2017.
Fang, W., Andersson, A., Zheng, M., Lee, M., Holmstrand, H., Kim, S.-W., Du, K., and Gustafsson, Ö.: Divergent Evolution of Carbonaceous Aerosols during Dispersal of East Asian Haze, Scientific Reports, 7, 10422, https://doi.org/10.1038/s41598-017-10766-4, 2017.
Fu, P., Kawamura, K., Chen, J., Qin, M., Ren, L., Sun, Y., Wang, Z., Barrie, L. A., Tachibana, E., Ding, A., and Yamashita, Y.: Fluorescent water-soluble organic aerosols in the High Arctic atmosphere, Sci. Rep., 5, 9845, https://doi.org/10.1038/srep09845, 2015.
Guo, S., Hu, M., Zamora, M. L., Peng, J., Shang, D., Zheng, J., Du, Z., Wu, Z., Shao, M., Zeng, L., Molina, M. J., and Zhang, R.: Elucidating severe urban haze formation in China, P. Natl. Acad. Sci. USA, 111, 17373–17378, https://doi.org/10.1073/pnas.1419604111, 2014.
Hecobian, A., Zhang, X., Zheng, M., Frank, N., Edgerton, E. S., and Weber, R. J.: Water-Soluble Organic Aerosol material and the light-absorption characteristics of aqueous extracts measured over the Southeastern United States, Atmos. Chem. Phys., 10, 5965–5977, https://doi.org/10.5194/acp-10-5965-2010, 2010.
Huang, R. J., Zhang, Y., Bozzetti, C., Ho, K. F., Cao, J. J., Han, Y., Daellenbach, K. R., Slowik, J. G., Platt, S. M., Canonaco, F., Zotter, P., Wolf, R., Pieber, S. M., Bruns, E. A., Crippa, M., Ciarelli, G., Piazzalunga, A., Schwikowski, M., Abbaszade, G., Schnelle-Kreis, J., Zimmermann, R., An, Z., Szidat, S., Baltensperger, U., El Haddad, I., and Prevot, A. S.: High secondary aerosol contribution to particulate pollution during haze events in China, Nature, 514, 218–222, https://doi.org/10.1038/nature13774, 2014.
Jimenez, J. L., Canagaratna, M. R., Donahue, N. M., Prevot, A. S. H., Zhang, Q., Kroll, J. H., DeCarlo, P. F., Allan, J. D., Coe, H., Ng, N. L., Aiken, A. C., Docherty, K. S., Ulbrich, I. M., Grieshop, A. P., Robinson, A. L., Duplissy, J., Smith, J. D., Wilson, K. R., Lanz, V. A., Hueglin, C., Sun, Y. L., Tian, J., Laaksonen, A., Raatikainen, T., Rautiainen, J., Vaattovaara, P., Ehn, M., Kulmala, M., Tomlinson, J. M., Collins, D. R., Cubison, M. J., Dunlea, E. J., Huffman, J. A., Onasch, T. B., Alfarra, M. R., Williams, P. I., Bower, K., Kondo, Y., Schneider, J., Drewnick, F., Borrmann, S., Weimer, S., Demerjian, K., Salcedo, D., Cottrell, L., Griffin, R., Takami, A., Miyoshi, T., Hatakeyama, S., Shimono, A., Sun, J. Y., Zhang, Y. M., Dzepina, K., Kimmel, J. R., Sueper, D., Jayne, J. T., Herndon, S. C., Trimborn, A. M., Williams, L. R., Wood, E. C., Middlebrook, A. M., Kolb, C. E., Baltensperger, U., and Worsnop, D. R.: Evolution of organic aerosols in the atmosphere, Science, 326, 1525–1529, https://doi.org/10.1126/science.1180353, 2009.
Kirillova, E. N., Sheesley, R. J., Andersson, A., and Gustafsson, O.: Natural abundance 13C and 14C analysis of water-soluble organic carbon in atmospheric aerosols, Anal. Chem., 82, 7973–7978, https://doi.org/10.1021/Ac1014436, 2010.
Kirillova, E. N., Andersson, A., Sheesley, R. J., Kruså, M., Praveen, P. S., Budhavant, K., Safai, P. D., Rao, P. S. P., and Gustafsson, Ö.: 13C and 14C-based study of sources and atmospheric processing of water-soluble organic carbon (WSOC) in South Asian aerosols, J. Geophys. Res., 118, 614–626, https://doi.org/10.1002/jgrd.50130, 2013.
Kirillova, E. N., Andersson, A., Han, J., Lee, M., and Gustafsson, O.: Sources and light absorption of water-soluble organic carbon aerosols in the outflow from northern China, Atmos. Chem. Phys., 14, 1413–1422, https://doi.org/10.5194/acp-14-1413-2014, 2014a.
Kirillova, E. N., Andersson, A., Tiwari, S., Srivastava, A. K., Bisht, D. S., and Gustafsson, O.: Water-soluble organic carbon aerosols during a full New Delhi winter: Isotope-based source apportionment and optical properties, J. Geophys. Res., 119, 3476–3485, https://doi.org/10.1002/2013jd020041, 2014b.
Kondo, Y., Miyazaki, Y., Takegawa, N., Miyakawa, T., Weber, R. J., Jimenez, J. L., Zhang, Q., and Worsnop, D. R.: Oxygenated and water-soluble organic aerosols in Tokyo, J. Geophys. Res., 112, D01203, https://doi.org/10.1029/2006jd007056, 2007.
Laskin, A., Laskin, J., and Nizkorodov, S. A.: Chemistry of Atmospheric Brown Carbon, Chem. Rev. (Washington, DC, U. S.), 115, 4335–4382, https://doi.org/10.1021/cr5006167, 2015.
Levin, I., Naegler, T., Kromer, B., Diehl, M., Francey, R. J., Gomez-Pelaez, A. J., Steele, L. P., Wagenbach, D., Weller, R., and Worthy, D. E.: Observations and modelling of the global distribution and long-term trend of atmospheric 14CO2, Tellus B, 62, 26–46, https://doi.org/10.1111/j.1600-0889.2009.00446.x, 2010.
Limbeck, A., Handler, M., Neuberger, B., Klatzer, B., and Puxbaum, H.: Carbon-specific analysis of humic-like substances in atmospheric aerosol and precipitation samples, Anal. Chem., 77, 7288–7293, https://doi.org/10.1021/ac050953l, 2005.
Liu, J., Li, J., Vonwiller, M., Liu, D., Cheng, H., Shen, K., Salazar, G., Agrios, K., Zhang, Y., He, Q., Ding, X., Zhong, G., Wang, X., Szidat, S., and Zhang, G.: The importance of non-fossil sources in carbonaceous aerosols in a megacity of central China during the 2013 winter haze episode: A source apportionment constrained by radiocarbon and organic tracers, Atmos. Environ., 144, 60–68, https://doi.org/10.1016/j.atmosenv.2016.08.068, 2016.
Liu, J. W., Li, J., Zhang, Y. L., Liu, D., Ding, P., Shen, C. D., Shen, K. J., He, Q. F., Ding, X., Wang, X. M., Chen, D. H., Szidat, S., and Zhang, G.: Source Apportionment Using Radiocarbon and Organic Tracers for PM2.5 Carbonaceous Aerosols in Guangzhou, South China: Contrasting Local- and Regional-Scale Haze Events, Environ. Sci. Technol., 48, 12002–12011, https://doi.org/10.1021/Es503102w, 2014.
Mayol-Bracero, O. L., Guyon, P., Graham, B., Roberts, G., Andreae, M. O., Decesari, S., Facchini, M. C., Fuzzi, S., and Artaxo, P.: Water-soluble organic compounds in biomass burning aerosols over Amazonia – 2. Apportionment of the chemical composition and importance of the polyacidic fraction, J. Geophys. Res., 107, D8091, https://doi.org/10.1029/2001jd000522, 2002.
Miyazaki, Y., Kondo, Y., Takegawa, N., Komazaki, Y., Fukuda, M., Kawamura, K., Mochida, M., Okuzawa, K., and Weber, R. J.: Time-resolved measurements of water-soluble organic carbon in Tokyo, J. Geophys. Res., 111, D23206, https://doi.org/10.1029/2006JD007125, 2006.
Mohn, J., Szidat, S., Fellner, J., Rechberger, H., Quartier, R., Buchmann, B., and Emmenegger, L.: Determination of biogenic and fossil CO2 emitted by waste incineration based on 14CO2 and mass balances, Bioresour. Technol., 99, 6471–6479, https://doi.org/10.1016/j.biortech.2007.11.042, 2008.
Noziere, B., Kalberer, M., Claeys, M., Allan, J., D'Anna, B., Decesari, S., Finessi, E., Glasius, M., Grgic, I., Hamilton, J. F., Hoffmann, T., Iinuma, Y., Jaoui, M., Kahnt, A., Kampf, C. J., Kourtchev, I., Maenhaut, W., Marsden, N., Saarikoski, S., Schnelle-Kreis, J., Surratt, J. D., Szidat, S., Szmigielski, R., and Wisthaler, A.: The molecular identification of organic compounds in the atmosphere: state of the art and challenges, Chem. Rev., 115, 3919–3983, https://doi.org/10.1021/cr5003485, 2015.
Pavuluri, C. M., Kawamura, K., Uchida, M., Kondo, M., and Fu, P. Q.: Enhanced modern carbon and biogenic organic tracers in Northeast Asian aerosols during spring/summer, J. Geophys. Res., 118, 2362–2371, https://doi.org/10.1002/Jgrd.50244, 2013.
Ruellan, S. and Cachier, H.: Characterisation of fresh particulate vehicular exhausts near a Paris high flow road, Atmos. Environ., 35, 453–468, https://doi.org/10.1016/S1352-2310(00)00110-2, 2001.
Salazar, G., Zhang, Y. L., Agrios, K., and Szidat, S.: Development of a method for fast and automatic radiocarbon measurement of aerosol samples by online coupling of an elemental analyzer with a MICADAS AMS, Nucl. Instr. Meth. Phys. Res. B., 361, 163–167, https://doi.org/10.1016/j.nimb.2015.03.051, 2015.
Sannigrahi, P., Sullivan, A. P., Weber, R. J., and Ingall, E. D.: Characterization of water-soluble organic carbon in urban atmospheric aerosols using solid-state C-13 NMR spectroscopy, Environ. Sci. Technol., 40, 666–672, https://doi.org/10.1021/Es051150i, 2006.
Stuiver, M.: Discussion: Reporting of 14C data, Radiocarbon, 19, 355–363, 1977.
Sullivan, A. P., Frank, N., Kenski, D. M., and Collett, J. L.: Application of high-performance anion-exchange chromatography-pulsed amperometric detection for measuring carbohydrates in routine daily filter samples collected by a national network: 2. Examination of sugar alcohols/polyols, sugars, and anhydrosugars in the upper Midwest, J. Geophys. Res., 116, D08303, https://doi.org/10.1029/2010jd014169, 2011.
Szidat, S., Jenk, T. M., Gäggeler, H. W., Synal, H. A., Fisseha, R., Baltensperger, U., Kalberer, M., Samburova, V., Wacker, L., Saurer, M., Schwikowski, M., and Hajdas, I.: Source apportionment of aerosols by 14C measurements in different carbonaceous particle fractions, Radiocarbon, 46, 475–484, 2004.
Szidat, S., Ruff, M., Perron, N., Wacker, L., Synal, H.-A., Hallquist, M., Shannigrahi, A. S., Yttri, K. E., Dye, C., and Simpson, D.: Fossil and non-fossil sources of organic carbon (OC) and elemental carbon (EC) in Göteborg, Sweden, Atmos. Chem. Phys., 9, 1521–1535, https://doi.org/10.5194/acp-9-1521-2009, 2009.
Timonen, H., Carbone, S., Aurela, M., Saarnio, K., Saarikoski, S., Ng, N. L., Canagaratna, M. R., Kulmala, M., Kerminen, V. M., Worsnop, D. R., and Hillamo, R.: Characteristics, sources and water-solubility of ambient submicron organic aerosol in springtime in Helsinki, Finland, J. Aerosol Sci., 56, 61–77, https://doi.org/10.1016/j.jaerosci.2012.06.005, 2013.
Wacker, L., Fahrni, S. M., Hajdas, I., Molnar, M., Synal, H. A., Szidat, S., and Zhang, Y. L.: A versatile gas interface for routine radiocarbon analysis with a gas ion source, Nucl. Instrum. Meth. B, 294, 315–319, https://doi.org/10.1016/j.nimb.2012.02.009, 2013.
Weber, R. J., Sullivan, A. P., Peltier, R. E., Russell, A., Yan, B., Zheng, M., de Gouw, J., Warneke, C., Brock, C., Holloway, J. S., Atlas, E. L., and Edgerton, E.: A study of secondary organic aerosol formation in the anthropogenic-influenced southeastern United States, J. Geophys. Res., 112, D13302, https://doi.org/10.1029/2007jd008408, 2007.
Wozniak, A. S., Bauer, J. E., and Dickhut, R. M.: Characteristics of water-soluble organic carbon associated with aerosol particles in the eastern United States, Atmos. Environ., 46, 181–188, https://doi.org/10.1016/j.atmosenv.2011.10.001, 2012.
Xiao, R., Takegawa, N., Zheng, M., Kondo, Y., Miyazaki, Y., Miyakawa, T., Hu, M., Shao, M., Zeng, L., Gong, Y., Lu, K., Deng, Z., Zhao, Y., and Zhang, Y. H.: Characterization and source apportionment of submicron aerosol with aerosol mass spectrometer during the PRIDE-PRD 2006 campaign, Atmos. Chem. Phys., 11, 6911–6929, https://doi.org/10.5194/acp-11-6911-2011, 2011.
Yan, C., Zheng, M., Bosch, C., Andersson, A., Desyaterik, Y., Sullivan, A. P., Collett, J. L., Zhao, B., Wang, S., He, K., and Gustafsson, O.: Important fossil source contribution to brown carbon in Beijing during winter, Sci. Rep., 7, 43182, https://doi.org/10.1038/srep43182, 2017.
Zappoli, S., Andracchio, A., Fuzzi, S., Facchini, M. C., Gelencser, A., Kiss, G., Krivacsy, Z., Molnar, A., Meszaros, E., Hansson, H. C., Rosman, K., and Zebuhr, Y.: Inorganic, organic and macromolecular components of fine aerosol in different areas of Europe in relation to their water solubility, Atmos. Environ., 33, 2733–2743, https://doi.org/10.1016/S1352-2310(98)00362-8, 1999.
Zhang, Q., Jimenez, J. L., Canagaratna, M. R., Allan, J. D., Coe, H., Ulbrich, I., Alfarra, M. R., Takami, A., Middlebrook, A. M., Sun, Y. L., Dzepina, K., Dunlea, E., Docherty, K., DeCarlo, P. F., Salcedo, D., Onasch, T., Jayne, J. T., Miyoshi, T., Shimono, A., Hatakeyama, S., Takegawa, N., Kondo, Y., Schneider, J., Drewnick, F., Borrmann, S., Weimer, S., Demerjian, K., Williams, P., Bower, K., Bahreini, R., Cottrell, L., Griffin, R. J., Rautiainen, J., Sun, J. Y., Zhang, Y. M., and Worsnop, D. R.: Ubiquity and dominance of oxygenated species in organic aerosols in anthropogenically-influenced Northern Hemisphere midlatitudes, Geophys. Res. Lett., 34, L13801, https://doi.org/10.1029/2007gl029979, 2007.
Zhang, R., Li, Q., and Zhang, R.: Meteorological conditions for the persistent severe fog and haze event over eastern China in January 2013, Sci. China Earth Sci., 57, 26–35, https://doi.org/10.1007/s11430-013-4774-3, 2014.
Zhang, Y., Ren, H., Sun, Y., Cao, F., Chang, Y., Liu, S., Lee, X., Agrios, K., Kawamura, K., Liu, D., Ren, L., Du, W., Wang, Z., Prevot, A. S. H., Szidat, S., and Fu, P.: High Contribution of Nonfossil Sources to Submicrometer Organic Aerosols in Beijing, China, Environ. Sci. Technol., 51, 7842–7852, https://doi.org/10.1021/acs.est.7b01517, 2017.
Zhang, Y. L., Perron, N., Ciobanu, V. G., Zotter, P., Minguillón, M. C., Wacker, L., Prévôt, A. S. H., Baltensperger, U., and Szidat, S.: On the isolation of OC and EC and the optimal strategy of radiocarbon-based source apportionment of carbonaceous aerosols, Atmos. Chem. Phys., 12, 10841–10856, https://doi.org/10.5194/acp-12-10841-2012, 2012.
Zhang, Y. L., Zotter, P., Perron, N., Prévôt, A. S. H., Wacker, L., and Szidat, S.: Fossil and non-fossil sources of different carbonaceous fractions in fine and coarse particles by radiocarbon measurement, Radiocarbon, 55, 1510–1520, 2013.
Zhang, Y. L., Huang, R. J., El Haddad, I., Ho, K. F., Cao, J. J., Han, Y., Zotter, P., Bozzetti, C., Daellenbach, K. R., Canonaco, F., Slowik, J. G., Salazar, G., Schwikowski, M., Schnelle-Kreis, J., Abbaszade, G., Zimmermann, R., Baltensperger, U., Prévôt, A. S. H., and Szidat, S.: Fossil vs. non-fossil sources of fine carbonaceous aerosols in four Chinese cities during the extreme winter haze episode of 2013, Atmos. Chem. Phys., 15, 1299–1312, https://doi.org/10.5194/acp-15-1299-2015, 2015.
Zhang, Y.-L., Li, J., Zhang, G., Zotter, P., Huang, R.-J., Tang, J.-H., Wacker, L., Prévôt, A. S. H., and Szidat, S.: Radiocarbon-based source apportionment of carbonaceous aerosols at a regional background site on hainan Island, South China, Environ. Sci. Technol., 48, 2651–2659, https://doi.org/10.1021/es4050852, 2014a.
Zhang, Y.-L., Liu, J.-W., Salazar, G. A., Li, J., Zotter, P., Zhang, G., Shen, R.-r., Schäfer, K., Schnelle-Kreis, J., Prévôt, A. S. H., and Szidat, S.: Micro-scale (mug) radiocarbon analysis of water-soluble organic carbon in aerosol samples, Atmos. Environ., 97, 1–5, https://doi.org/10.1016/j.atmosenv.2014.07.059, 2014b.
Zhang, Y.-L., Schnelle-Kreis, J. r., Abbaszade, G. l., Zimmermann, R., Zotter, P., Shen, R.-R., Schafer, K., Shao, L., Prévôt, A. S. H., and Szidat, S. N.: Source apportionment of elemental carbon in Beijing, China: Insights from radiocarbon and organic marker measurements, Environ. Sci. Technol., 49, 8408–8415, 2015.
Zong, Z., Wang, X., Tian, C., Chen, Y., Han, G., Li, J., and Zhang, G.: Source and formation characteristics of water-soluble organic carbon in the anthropogenic-influenced Yellow River Delta, North China, Atmos. Environ., 144, 124–132, https://doi.org/10.1016/j.atmosenv.2016.08.078, 2016.
Zotter, P., Ciobanu, V. G., Zhang, Y. L., El-Haddad, I., Macchia, M., Daellenbach, K. R., Salazar, G. A., Huang, R. J., Wacker, L., Hueglin, C., Piazzalunga, A., Fermo, P., Schwikowski, M., Baltensperger, U., Szidat, S., and Prévôt, A. S. H.: Radiocarbon analysis of elemental and organic carbon in Switzerland during winter-smog episodes from 2008 to 2012 – Part 1: Source apportionment and spatial variability, Atmos. Chem. Phys., 14, 13551–13570, https://doi.org/10.5194/acp-14-13551-2014, 2014a.
Zotter, P., El-Haddad, I., Zhang, Y., Hayes, P. L., Zhang, X., Lin, Y.-H., Wacker, L., Schnelle-Kreis, J., Abbaszade, G., Zimmermann, R., Surratt, J. D., Weber, R., Jimenez, J. L., Szidat, S., Baltensperger, U., and Prévôt, A. S. H.: Diurnal cycle of fossil and nonfossil carbon using radiocarbon analyses during CalNex, J. Geophys. Res., 119, 6818–6835, https://doi.org/10.1002/2013jd021114, 2014b.