the Creative Commons Attribution 4.0 License.
the Creative Commons Attribution 4.0 License.
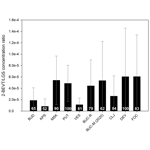
Assessment of the contribution of residential waste burning to ambient PM10 concentrations in Hungary and Romania
András Hoffer
Aida Meiramova
Ádám Tóth
Beatrix Jancsek-Turóczi
Gyula Kiss
Ágnes Rostási
Erika Andrea Levei
Luminita Marmureanu
Attila Machon
András Gelencsér
The illegal burning of solid waste in residential stoves is an existing practice, but until now it has been completely disregarded as an emission source of atmospheric pollutants in many developed countries, including those in eastern Europe. Various types of solid waste (plastics, treated wood, plyboards, tyre, rag) serve as an auxiliary fuel in many households, in particular during the heating season. In this work, for the first time ever in atmospheric pollution studies, specific tracer compounds identified previously in controlled test burnings of different waste types in the laboratory were detected and quantified in ambient PM10 samples collected in five Hungarian and four Romanian settlements. Using the identified tracers and their experimentally determined relative emission factors, the potential contribution of illegal waste burning emissions to ambient PM10 mass concentrations was assessed. Our findings implied that the burning of polyethylene terephthalate (PET)-containing waste (food and beverage packaging, clothes) was predominant at all the locations, especially in north-eastern Hungary and Romania. There is substantial evidence that the burning of scrap furniture is also common in big cities in Hungary and Romania. Back-of-the-envelope calculations based on the relative emission factors of individual tracers suggested that the contribution of solid waste burning particulate emissions to ambient PM10 mass concentrations may be as high as a few percent. This finding, when considering the extreme health hazards associated with particulate emissions from waste burning, is a matter of serious public health concern.
- Article
(2507 KB) - Full-text XML
-
Supplement
(818 KB) - BibTeX
- EndNote
Burning of solid fuels in households is a significant source of atmospheric particulate matter and gaseous pollutants worldwide. In most countries in Europe the predominant type of solid fuel in households is fuel wood. Using the tracer approach based on the cellulose pyrolysis product levoglucosan, Caseiro et al. (2009) estimated that the contributions of wood burning to the PM10 concentrations were 10 % and 20 % in Vienna and in rural settlements in Austria, respectively. In Budapest, up to 40 % of the carbon in PM10 was found to be emitted from wood burning in winter (Salma et al., 2017). The organic carbon emitted from biomass burning represented about 80 % of the PM1 carbon in winter near Bucharest, as determined by a compact time-of-flight aerosol mass spectrometer (Marmureanu et al., 2020).
In addition to biomass burning, the burning of different types of household waste is also an important emission source of particulate matter worldwide (Christian et al., 2010; Wiedinmyer et al., 2014; Kumar et al., 2015). Open waste burning is quite common in many countries, especially those in which organized waste collection systems are lacking or costly or in which collection services are infrequent. Furthermore, a lack of discipline and commitment within local governments and communities might also promote the habit of burning waste outdoors (Ramadan et al., 2022). Emissions from modern waste incinerator plants are incomparably lower than from open waste burning or from the burning of solid waste in household stoves (Lemieux et al., 2004; Jones and Harrison 2016). Based on the high concentrations of phthalic acid and bisphenol-A in urban particulates, Kanellopoulos et al. (2021) suggested that the burning of plastic waste is a non-negligible source of air pollution, especially in the autumn and winter season in the industrial district of Aspropyrgos, Greece. Wiedinmyer et al. (2014) estimated the amount of waste burned in households and at dump sites based on the general guidelines reported by the IPCC in 2006. For Hungary and Romania, they found that the amount of waste burned on an annual basis was about 2 % and 60 % of the generated waste of a given country, respectively. The estimated contributions of waste burning to the ambient PM10 were 4 % and 35 % in Hungary and Romania, respectively.
As an illegal and uncontrolled practice, the assessment of the magnitude of residential waste burning and its effect on air quality poses an extreme challenge to environmental authorities. Apart from the fact that the amount of solid waste produced in households can only be estimated with a high level of uncertainty, the fraction which is burned in residential stoves is practically unknown. Quite recently two independent surveys were conducted targeting waste burning practices in Hungary. The survey organized by Kantar Hoffmann Ltd. (2021) found that about 10 % of the pollees admitted to burning solid waste on a regular basis, mostly plastics, treated wood, and clothes. A telephone-based survey conducted by the Századvég Foundation in 2018 concluded that 4 % of the population burned household waste (treated wood, rags, paper, plastics) indoors, nearly half of it on a daily basis.
Hoffer et al. (2021) measured the emission factors of PM10 and particle-bound polycyclic aromatic hydrocarbons (PAHs) emitted upon burning of various types of solid waste in a residential stove in the laboratory. Potential tracer compounds specific to different waste types were also identified, and their emission factors were determined. The 1,3,5-triphenylbenzene is considered to be a non-specific tracer of low-temperature burning of several types of solid waste (polystyrene, PS; polyethylene terephthalate, PET; coated paper, furniture panels, acrylonitrile butadiene styrene polymer, ABS) and has been identified in atmospheric particulates (Simoneit et al., 2005; Kumar et al., 2015; Zhao et al., 2018; Furman et al., 2021). Although there are a few studies on the estimated mass contribution of solid waste burning (Islam et al., 2022) to ambient PM10 concentrations, to the best of our knowledge, our work is the very first study that attempts to quantitatively assess the magnitude of residential waste burning particulate emissions and their contribution to atmospheric PM10 concentrations in different settlements based on the laboratory measurements of the relative emission factors of highly specific tracers of waste burning. Our approach is analogous to the source apportionment of biomass burning in atmospheric aerosol using levoglucosan as a tracer.
2.1 Sampling
2.1.1 Sampling sites
Sampling was performed at five Hungarian and four Romanian settlements, and their geographic locations are shown in Fig. 1.
The measurement station in Miskolc (MSK) was located in the city centre (Búza Square) near two busy roads and classified as a traffic-impacted site. Putnok (PUT) is a small town located in the Sajó Valley, which is reportedly the most polluted region in Hungary with respect to the number of exceedances of the health limit for PM10 mass concentrations. Here the sampling station was located inside the courtyard of the High School for Agriculture, and the sampling site is classified as rural. In the city of Budapest (BUD) the sampling was performed in the garden of the György Marczell Observatory of the Hungarian Meteorological Service located 14 km south-east of the city centre in the middle of a suburban residential area. K-Puszta (KPS) is a regional background air quality monitoring station located in a forest clearing 15 km from the city centre of Kecskemét. In Veszprém (VES) PM10 samples were collected in a residential area in a small river valley close to the city centre (Patak Square). In Deva (DEV) the sampling site was downtown in the yard of a company, in Dragos Voda Street, about 10 km from a coal- and natural-gas-fired power station. In Cluj-Napoca (CLJ) samples were collected in the yard of the Research Institute for Analytical Instrumentation in the western part of the city (Donath Street) near two busy roads in the immediate vicinity of the suburban air quality monitoring station of the Romanian Environmental Protection Agency. In Focsani (FOC) the sampling was performed in the backyard of a private house in a suburban residential area. In Bucharest PM10 samples were collected in the metropolitan area of Măgurele, which is located about 12 km from the city centre to the south-west. In Măgurele the PM10 samples were collected at two sampling stations: one was located in the yard of the National Institute for Research and Development for Optoelectronics, INOE 2000 (BUC-R), and the other station (BUC-M) was located 2 km away from the first station (Atmosferei Street) and was only used during the winter of 2020.
At all the stations, the PM10 samples were collected on quartz filters (Advantec QR-100) with Digitel DHA-80 high-volume samplers. The sampling time was 24 h, starting from midnight for each sample. Before and after the sampling the filters were conditioned in a weighting room at 20 ± 1 ∘C and at a relative humidity of 45 %–50 % for 3 d. The PM10 mass concentrations were determined according to the EN 12341:2014 Ambient air-Standard gravimetric measurement method.
2.1.2 PM10 filter samples
PM10 filter samples were collected during the heating seasons of 2018/2019 and 2019/2020 and also in the summer of 2019. Table 1 summarizes the sampling dates, the number of samples analysed, and the average PM10 concentrations obtained from the gravimetric analyses.
Table 1The sampling dates, number of samples analysed, and average PM10 concentrations with standard deviations (SDs).
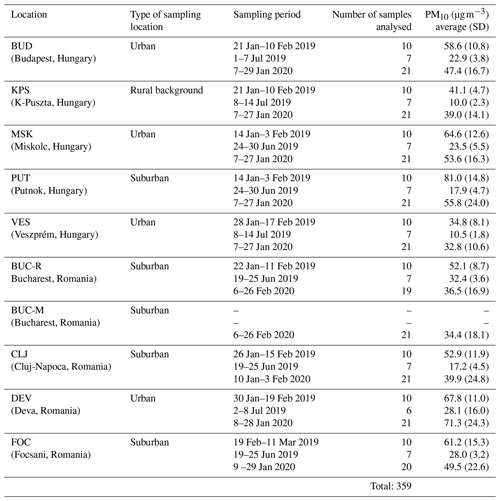
Among the samples collected in the winter of 2019, those with the 10 highest PM10 concentrations were selected for gas chromatography-mass spectrometry (GC-MS) analysis, whereas from 2021 nearly all the samples collected on consecutive days were analysed. This is also reflected in the PM10 mass concentrations reported in Table 1, as the average PM10 concentrations are typically higher for the winter of 2019 than for 2020. During the sampling periods, blank samples were collected at each sampling site and were also analysed.
2.2 GC-MS analysis of the samples for tracer compounds
The GC-MS method for the analysis of tracer compounds was adopted from Hoffer et al. (2021). From the ambient samples, 18.1 cm2 of the filters were extracted three times in dichloromethane-methanol 2 : 1 mixture. The extraction efficiency was followed by a recovery standard (p-terphenyl-d14) added to the samples before the extraction. During the first extraction step, 7.5 mL of dichloromethane : methanol (2 : 1) mixture was applied to the filter portion and the sample was shaken in a vortex agitator for 1 h. The volumes of the second and third extracts were 6 and 5 mL, respectively. The combined extracts were then filtered through a syringe filter (0.45 µm) and dried under an N2 stream. The redissolved (in dichloromethane : methanol 2 : 1 mixture) sample extracts were then directly analysed for the less polar tracer compounds, while the more polar compounds (levoglucosan, terephthalic acid, melamine) were measured after derivatization with BSTFA-TMCS (N,O-bis(trimethylsilyl)trifluoroacetamide-trimethylchlorosilane, 99 : 1, (Sigma-Aldrich) and pyridine (anhydrous, Merck) (1 : 1 volume ratio) at 80 ∘C for 1 h, with an Agilent 6890 gas chromatograph coupled to an Agilent 6973 mass spectrometer. The column type and the temperature programmes were the same as in Hoffer et al. (2021). In the samples the amounts of m-terphenyl (m-TPH), p-terphenyl (p-TPH), 1,3,5-triphenylbenzene (135-TPB), 1,2,4-triphenylbenzene (124-TPB), quaterphenyl isomers (m,p-QTPH, p-QTPH), 2-(benzoyloxy)ethyl vinyl terephthalate (2-BEVT), 5-hexene-1,3,5-triyltribenzene (styrene trimer, SSS), 2-methylene-4,6-diphenylhexanenitrile (ASS), 2-phenethyl-4-phenylpent-4-enenitrile (SAS), 4,6-diphenylhept-6-enenitrile (SSA), levoglucosan (LGS), melamine, and terephthalic acid (TPA) were measured. The identification of these compounds was based on the method reported in Hoffer et al. (2021). The calibration of the instrument was performed with standard solutions, but because of the lack of available individual standards the mass concentrations of some compounds were quantified based on calibration curves of other similar compounds: the terphenyls were expressed as p-TPH, 124-TPB, and quaterphenyls as well as the 2-BEVT as 135-TPB, the ASS, SSA, and SAS as SSS as detailed in Hoffer et al. (2021).
Blank samples were also analysed and the limit of quantification (LOQ) values were calculated as the average of the blanks +10 times the standard deviation of the blanks. The LOQ values were obtained separately for the 2019 and 2020 winter campaigns as well as for the summer campaign separately for the Hungarian and Romanian samples.
3.1 Burning of waste containing PET
The tracers of the emissions from the burning of PET-containing waste (usually PET bottles and textiles containing “polyester”) can be manifold, but recently we identified a highly specific 2-(benzoyloxy) ethyl vinyl terephthalate (2-BEVT) (Hoffer et al., 2021). Figure 2 shows the average 2-BEVT : levoglucosan (LGS) ratio obtained at the different sampling sites in Hungary and Romania for the winter samples. These mass concentration ratios may be used to weigh the relative ratio of PET burning emissions to firewood burning among the different settlements. The mass concentrations of 2-BEVT were in some samples below the quantification limit (LOQ). These values were considered zero in calculating the average. The numbers shown inside the bars represent the percentage of cases in which the 2-BEVT were determined quantitatively (the data coverage of the LGS concentrations was 100 %), and the error bars show the standard deviation of the data.
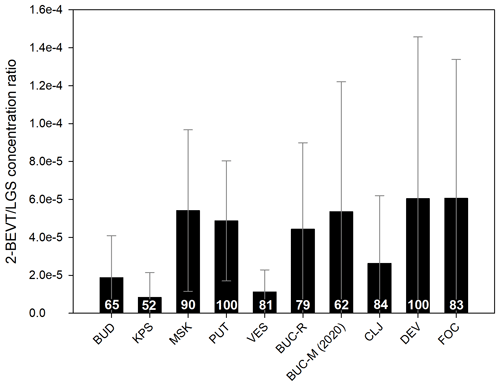
Figure 2The average mass concentration ratios of 2-BEVT to LGS in the PM10 samples collected at the different locations in the winters of 2019 and 2020. The numbers shown inside the bars represent the percentage of cases in which the concentrations of 2-BEVT were above the limit of quantification. The error bars represent the standard deviation of all the data.
The figure shows that in Hungary the relative contribution of PET burning is significantly higher in the north-eastern region (MSK and PUT) than elsewhere in the country. The difference may be up to a factor of 5 between the most and least affected settlements (MSK and VES). It should be noted that in terms of wintertime PM10 concentrations (see Table 1) the trends are identical, but the concentration ratios are much lower (only about a factor of 2 between MSK and VES). In Putnok 2-BEVT was quantified in all the analysed samples, whereas its concentration was above the limit of quantification in only half of the samples collected at the background station of K-Puszta. Since the mass concentrations of levoglucosan were highest in Putnok (Fig. S1 in the Supplement), the combination of these results showed that the highest absolute 2-BEVT concentrations were also measured there: on average, the mass concentrations of 2-BEVT found in Putnok was about a factor of 5 higher than that in Budapest and up to a factor of 15 higher than that in Veszprém. These findings are by far out of proportion to the differences in ambient PM10 concentrations. It should be noted that, since lignite is a frequently used solid fuel in Putnok and the emission factor of levoglucosan from lignite combustion may be higher than in the case of wood burning (Fabbri et al., 2009), this may lead to some bias in the observed 2-BEVT : levoglucosan ratios. In Veszprém, the relative importance of PET burning is found to be much lower even though its tracer was quantified in the vast majority (80 %) of the PM10 samples. In contrast, 2-BEVT was quantified in only 66 % of the PM10 samples in Budapest, yet the relative share of PET burning was found to be higher on average than that in Veszprém.
In the case of the PM10 samples collected in Romania, the relative share of PET burning on average was found to be largely comparable with those obtained at the most polluted Hungarian sites, with the exception of Cluj-Napoca. At the sampling locations in Bucharest (Măgurele), the 2-BEVT was quantified in about 70 % of the PM10 samples, and its concentration ratio to LGS was among the highest. This implies quite intensive PET-containing waste burning in the region. According to the measured 2-BEVT concentrations, the sites most heavily impacted by PET burning were Deva and Focsani in the study period. In Deva 2-BEVT was quantified in all the PM10 samples, and the corresponding ratios in Focsani and Cluj-Napoca were 83 % and 84 %, respectively. However, in the latter city, the relative share of PET burning was found to be relatively low (but higher than that obtained for Budapest). Although the numbers of the analysed samples were different in the two heating seasons, the concentration ratios of 2-BEVT to LGS at the majority of the sampling locations were largely comparable in the 2 years, with the exception of Cluj-Napoca and Focsani, at which waste burning was more intense in 2020 than in 2019. In the summertime samples the concentrations of 2-BEVT were below the limit of quantification in all but one of the samples.
It has been found recently that the burning of PET and PET-containing textiles releases significant amounts of m-terphenyl and p-terphenyl (Hoffer et al., 2021). While these compounds are not reported in particulates emitted by biomass burning and fossil fuel combustion, they were identified in lignite smoke (Fabbri et al., 2009). It has been shown that, besides the burning of PET-containing waste, the combustion of PS, ABS, and paper (the latter is for the meta-isomer) is also a significant source of terphenyls. In addition, the relative emission factors of the meta- and para-isomers are different for different types of waste. It was observed that p-terphenyl is emitted in higher quantities than m-terphenyl in the burning of PET-containing waste (e.g. PET flasks and clothes containing polyester), whereas for other waste types the ratio of the emission factor of the para-terphenyl to meta-terphenyl was lower than 1 (0.6 and 0.4 for PS and ABS, respectively). Figure 3 shows the average ratios of the mass concentrations of m-terphenyl and p-terphenyl at the different sampling sites.
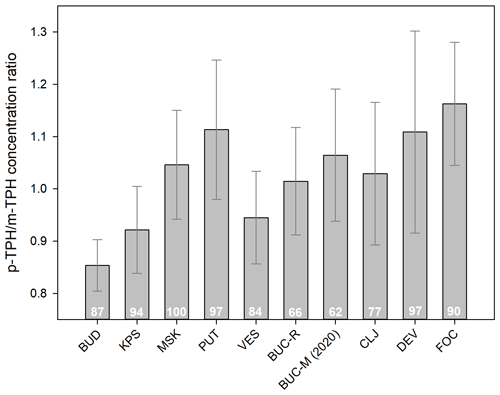
Figure 3The average mass concentration ratio of p-TPH and m-TPH in the PM10 samples collected at the different sampling sites. The numbers shown inside the bars represent the percentage of cases in which the concentrations of both isomers were above the limit of quantification. The error bars represent the standard deviation of all the data.
In spite of the fact that there is no statistically significant correlation between the 2-BEVT : LGS and p-TPH : m-TPH concentration ratios obtained for the PM10 samples collected at a given sampling site, it can be clearly seen in Fig. 2 that at the sampling locations implying a higher incidence of PET-waste burning (MSK, PUT, BUC-M, DEV, FOC), the ratios of the two terphenyl isomers are all higher than 1. Although there might be other emission sources of the terphenyl isomers (such as lignite burning), these findings also confirm that PET burning may indeed be a non-negligible emission source of particulates in the above settlements. This conclusion is also supported by the very high emission factor of the terphenyls from PET burning (Hoffer et al., 2021), but obviously, since the p-terphenly : m-terphenyl ratio is lower than that typical of PET burning emissions (1.1–1.7), the contribution of other sources to the terphenyl concentration should also be considered.
3.2 Burning of fibreboard, PS, and paper
The SSS is emitted mainly during the incomplete burning of polystyrene, but its relative emission factor () was also considerable in the case of LDF burning (coated low-density fibreboard used as furniture material) as well as during paper burning (printed and coated, waxy paper burning) (Hoffer et al., 2021). On a mass basis, about 2 orders of magnitude more SSS is emitted from PS than from LDF or PAP, but since the latter two are burned in much more significant quantities than PS, their contributions to its atmospheric concentration are at least comparable. Figure 4 shows the concentration ratio of the styrene trimer to the LGS measured in the ambient PM10 samples collected in Hungary and Romania.
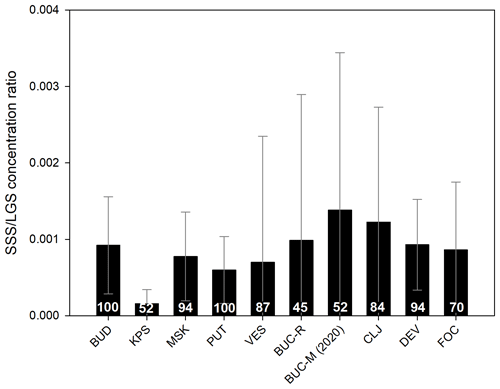
Figure 4The average relative mass concentration ratio of styrene trimer (SSS) to levoglucosan in the PM10 samples at the different sampling sites. The numbers shown inside the bars represent the percentage of cases in which the concentrations of SSS were above the limit of quantification. The error bars represent the standard deviation of all the data.
The average relative concentration of SSS was lowest at the Hungarian background station KPS, and SSS was quantified in only half of the samples collected at this station. By contrast, SSS was measured in all of the PM10 samples from Budapest and Putnok and in most of the samples collected in Miskolc and Veszprém. The concentration ratios of SSS and LGS were within a factor of 2 at all the sampling sites in Hungary except for KPS. The average SSS : LGS ratio was slightly higher in most of the PM10 samples in Romania (except for FOC). In the latter town there was a large year-to-year variability of the SSS concentration, as in 2019 its concentration was above the LOQ in only 20 % of the samples, whereas in 2020 the same ratio was 95 %. It is important to note that in the samples collected near Bucharest, SSS was only identified in about half of the samples (in BUC-R 60 % and 37 % in 2019 and 2020, respectively), but the average SSS : LGS ratio was among the highest, which may indicate that waste burning may either be highly episodic or is related to some specific wind sectors only. It is interesting to see that the SSS : LGS ratio is higher in towns with larger populations than in smaller settlements (BUD > MSK > VES and BUC, CLJ > DEV, FOC). This might indicate that the types of waste yielding SSS upon burning are more readily accessible in larger cities per unit number of wood-burning households. The concentrations of the SSS were below the LOQ in the PM10 samples collected during summer in both countries.
Hoffer et al. (2021) identified 135-TPB in most of the PM10 samples collected during the burning of different types of solid waste in the laboratory. This compound has been considered a universal tracer of waste burning (Simoneit et al., 2005). The 1,3,5-triphenylbenzene was quantified in almost all (> 93 %) the PM10 samples during winter. In the study period the average atmospheric concentrations of 135-TPB were highest in Bucharest and Cluj-Napoca (1.3 and 1.6 ng m−3, respectively). These concentrations are at the lower end of those obtained for Beijing during the summer season of 2008 (1.58–4.58 ng m−3, Li and Fang 2009) but were markedly higher than that measured in southern Poland in the heating season of 2017 (0.8 ng m−3, Furman et al., 2021). In Hungary the average atmospheric concentrations of 135-TPB varied between 0.25 and 0.42 ng m−3 at the different sampling stations.
Unlike the 2-BEVT and SSS, the relative concentration of the compounds containing four aromatic rings (the quaterphenyls m,p-QTPH and p-QTPH and the triphenyl-benzene isomers 135-TPB and 124-TPB) also show relatively high relative concentration values (see Fig. 5) at the background station (KPS), indicating that these compounds likely have longer residence times in the atmosphere (as follows from the absence of the double bond which is present in both the 2-BEVT and SSS). In Hungary the relative concentrations of quaterphenyls in LGS were highest in MSK, where PET burning was also found to be significant. Similarly, in Romania this ratio was highest in Bucharest, where the relative concentrations of both the SSS and the 2-BEVT were also highest. Although the relative concentrations of the quaterphenyls in LGS are highly variable at different stations, their ratio is only slightly higher (by 20 %–50 %) in the PM10 samples collected in Romanian settlements than those in Hungary. On the other hand, the relative amounts of the triphenylbenzenes in the LGS show much larger differences between the two countries: their relative concentrations are larger in the PM10 samples collected in Romania on average by a factor of 2–3 than those in Hungary. Exceptionally high concentrations of the triphenylbenzenes relative to LGS were obtained near Bucharest and in Cluj-Napoca. It is important to note that the large sample-to-sample variability (the large standard deviation of the measured ratio; see Fig. 5) at these stations may indicate the presence of strong and intermittent emission source(s) in the vicinity of the sampling sites. Li and Fang speculated that in Beijing the emission sources of 135-TPB might also be waste incineration plants and fossil fuel combustion; in that case 135-TPB may not be a reliable tracer of household waste burning. On the other hand, if the large variation in the atmospheric concentration of triphenylbenzenes can be attributed to the substantial changes in the types of solid waste that are burned (e.g. if the emission factor of triphenylbenzenes for PS burning is very large), it may still be a generic tracer of waste burning.
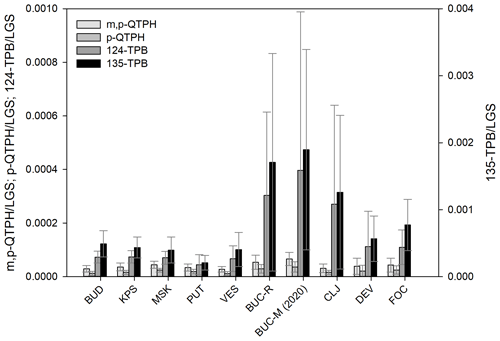
Figure 5The average relative mass concentration ratio of quaterphenyls and triphenylbenzenes to levoglucosan in the PM10 samples at the different sampling sites. The error bars represent the standard deviation of all the data. The percentage of cases in which the concentrations of the presented compounds were above the limit of quantification was larger than 87 %.
Unlike other more specific waste burning tracers, both quaterphenyls and triphenylbenzenes were also quantified in PM10 samples collected during summer (Fig. S2 in the Supplement). This might imply that there are other industrial or traffic-related emission sources. Furman et al. (2021) found that, outside the heating season in southern Poland, the relative concentration of 135-TPB in PM10 was still about 60 % of that found during winter. Here we also note that the p-quaterphenyl is emitted from aircraft engines, and it was suggested as a tracer of PAH pollution from aircraft (Krahl et al., 1998).
The ratio of the triphenylbenzenes and quaterphenyls showed large variation during the burning of different plastic types in the laboratory, and the concentration of the triphenylbenzenes was much larger during the burning of waste specimens containing styrene and/or also emitting SSS (PS, LDF, PAP, ABS) than in the case of PET and RAG burning (Hoffer et al., 2021). The average m,p-QTPH : 124-TPB ratios were < 0.3 and 1.3–2.3 for the styrene-containing waste (LDF, ABS, PS, and PAP) and for the PET-containing waste, respectively.
Figure 6 shows that this ratio is largest in MSK and PUT, implying that a large amount of PET-containing waste is burned in the region. In Romania, lower m,p-QTPH : 124-TPB ratios were found at all the stations, which, together with the elevated concentrations of SSS, suggested that large quantities of styrene-containing waste (PS, LDF, PAP) were burned in the vicinity of the sampling sites. The higher m,p-QTPH : 124-TPB concentration ratios found at DEV and FOC support enhanced rates of PET burning (2-BEVT : LGS ratio) at these locations. It should be noted that in Hungary and Romania the concentration ratio m,p-QTPH : 124-TPB is below 1, which implies that the concentration of these components might be determined by the burning of LDF, PS, and PAP in both countries.
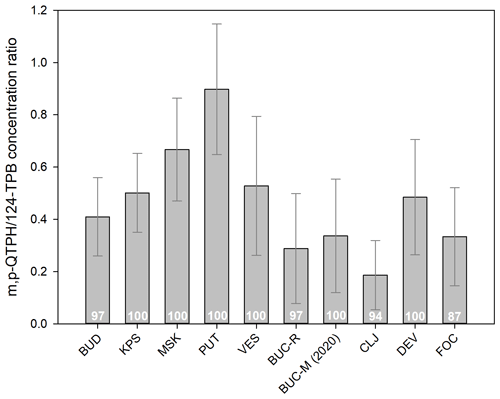
Figure 6The average relative concentration ratio of m,p-QTPH to 124-TPH at the different sampling locations. The numbers shown inside the bars represent the percentage of cases in which the concentrations of both isomers were above the limit of quantification. The error bars represent the standard deviations of all the data.
While SSS can be emitted from various sources, melamine is more specific to the burning of materials containing melamine–formaldehyde resins, e.g. coated fibreboards used as furniture parts. Based on the melamine : LGS ratio (Fig. 7), the extent of the burning of furniture panels can be compared at the different sampling sites. Here we note that melamine concentrations were below the LOQ (except for one sample from Putnok) in the PM10 samples collected in the winter of 2019 in Hungary and were quantified as being only about half (43 %–57 %) of the samples from 2020. In the PM10 samples collected in Romania, this compound was quantified in the majority (50 %–100 %) of the samples in both years, indicating that the burning of furniture panels is likely more common in Romania than in Hungary (Fig. 7). It should also be noted that in both countries the burning of furniture panels is more common in the capitals than in other settlements, possibly due to the much wider availability of scrap furniture in the high-income regions.
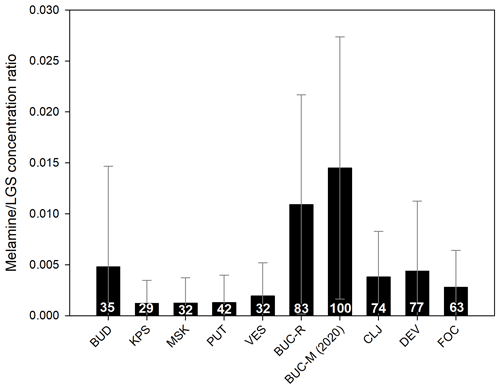
Figure 7The relative concentration ratio of melamine to levoglucosan in PM10 samples from the heating periods. The numbers shown inside the bars represent the percentage of cases in which the concentrations of melamine were above the limit of quantification. The error bars represent the standard deviations of all the data.
Melamine was also sporadically identified in some Hungarian and Romanian samples collected during summer, but its relative contribution to PM10 concentrations was much lower than during winter. There might be some sporadic burning of furniture panels in summer or emissions from local furniture plants (e.g. manufacturing and tailoring of furniture panels).
In the samples collected during the heating season, the pyrolysis products of the ABS (ASS, SSA, SAS) were also identified (Fig. 8). These compounds are released into the atmosphere not only from the burning of scrap electronic devices, but also from the burning of furniture panels as widely used as edge banding tapes for chipboards, medium-density fibreboard (MDF), or high-density fibreboard (HDF). Similarly to melamine, the relative concentrations of the different ABS pyrolysis products in the LGS were highest in the samples collected in Budapest and Bucharest, also supporting scrap electronic devices and furniture being more readily available for burning in large cities.
3.3 Assessment of the potential contribution of household waste burning to ambient PM10 mass concentrations
The contribution of particulate emissions from household waste burning to ambient PM10 mass concentrations at the Hungarian and Romanian sampling sites was assessed incrementally for the first time from the measured atmospheric concentrations of quaterphenyls, 2-BEVT, SSS, ASS, SAS, SSA, and melamine using estimated waste-component-specific EFs based on the data reported by Hoffer et al. (2021). The component-specific EFs were weighted averages for waste mixtures based on their estimated abundance in household waste, as some compounds are emitted from multiple sources. For this estimation, furniture panels, paper, rags, and household waste containing a mixture of different plastics were treated separately. Taking into account the plastic composition of municipal waste (Bodzay and Bánhegyi, 2016), it was assumed that the plastic-type household waste consisted of 42 % PE, 28 % PET, 14 % PP and PS, and 0.7 % PVC and ABS. Furthermore, EFs were calculated for waste mixtures in which the mass of furniture boards varied between 10 % and 91.7 % and that of the rags, paper, and mixed plastics from household waste varied between 1.8 % and 65.2 %. Table 2 shows the relative EFs of specific tracers as well as the absolute PM10 emission factors for the burning of individual waste types (taken from Hoffer et al., 2020) and summarizes the range of EFs as well as the average for the prescribed real-life waste mixtures whose compositional ranges are shown in the first column of the table.
Table 2The estimated emission factors for different tracer compounds for the burning of waste mixtures.
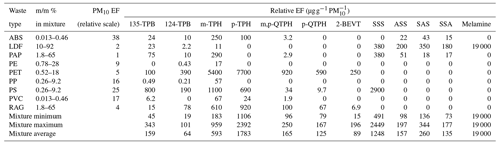
Table 3Estimated mass contributions of waste burning particulate emissions to ambient PM10 concentrations at the different sampling sites in Hungary and Romania based on the measured atmospheric concentrations of the different tracer compounds as well as their relative EFs taken from laboratory measurements. The data are in percentages (m/m %) of the measured PM10 concentrations.
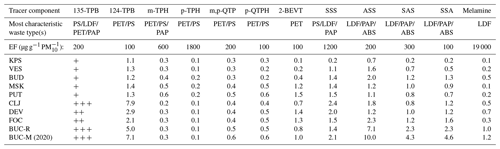
It should be noted that the assessments of the contribution of particulate emission from residential waste burning to the ambient PM10 concentration are loaded with very high uncertainties emerging from the large variations of the EFs (which depend on the burning conditions and the type of waste burned) and also from the fact that most tracer compounds are emitted upon the burning of several waste types (e.g. SSS from PS but also from paper and LDF), with vastly different emission factors. In addition, all waste burning tracers are considered refractory in the atmosphere. That is, they do not decompose during atmospheric transport. Because of all of these inherent uncertainties, the assessment should only be treated as a first-order approximation. Table 3 summarizes the estimated contributions of waste burning particulate emissions to ambient PM10 concentrations based on the measured atmospheric concentrations of the different tracer compounds as well as their relative EFs taken from laboratory measurements.
Table 3 demonstrates that the estimated contribution of PM10 emitted from the burning of different waste types in households to the atmospheric PM10 concentration is of the order of a few percent based on the measured ambient concentrations and relative emission factors of waste burning tracers. Since there may be additional sources of 135-TPB (its relative contributions to ambient PM10 were also significant in summer at some sampling sites) and the very large uncertainty in its EF, in the column based on its ambient concentrations only the magnitude of the estimated contribution of waste burning is indicated in the table. Although the data calculated from the individual tracers should not be added due to the considerable overlap between the sources, the PET-, PS-, LDF-, PAP-, and ABS-related sets of data may be, since they represent distinctly different types of waste burned. By doing so, the estimated contributions of waste burning particulate emissions to ambient PM10 were found to be lowest for the regional background station KPS and for Veszprém. In Budapest there is a strong indication that scrap furniture was burned in significant quantities in household stoves and possibly in repair shops, whereas in MSK and PUT the burning of common household waste (PET and/or RAG) was more typical. The results also imply that in Bucharest and Cluj the burning of scrap furniture was also quite common but was accompanied by higher contributions from PET and other household waste burning compared to those found for Budapest. In Deva and Focsani the contribution of PET and/or RAG burning emissions was predominant, similar to that found in PUT and MSK, with some additional contributions from the burning of other waste types (e.g. PS or LDF).
3.4 Assessment of the quantities of household waste burned in households and repair shops
Using the absolute emission factors of the different tracer compounds determined upon controlled waste burning in the laboratory as well as the reported emission factors for levoglucosan from wood burning, the quantities of solid waste burned in households may be estimated relative to the amount of firewood for which statistical data are available. This assessment can only be considered back-of-the-envelope calculations because of the vast uncertainties associated with emission measurements, variable burning conditions, degree of co-firing, atmospheric stability of tracers, and all other underlying assumptions. Table 3 summarizes the obtained results along with the applied EFs, which were estimated similarly to the case of the relative EFs (Table 2) using the reported data from Hoffer et al. (2021). For the calculations, we assumed that levoglucosan is emitted solely from wood burning at a rate of 200 mg kg−1 (Jimenez et al., 2017).
Table 4The estimated mass of solid waste burned relative to firewood (in percentage) at the different locations based on the measured ambient concentrations of different tracer compounds. The first row shows the absolute emission factors of waste burning tracers as determined experimentally in Hoffer et al. (2021).
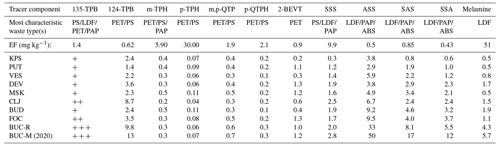
The results summarized in Table 4 indicate that the mass of household waste burned relative to that of firewood is of the order of a few percent (up to 5 %), but at some sites (BUD, BUC, DEV, and FOC) in Romania it is somewhat larger, primarily as a consequence of excess LDF, PAP, or ABS burning. Note that different tracers used for the same types of waste burned yield largely similar orders of magnitudes in the estimations. It can be seen that, on a mass basis, LDF, PAP, and ABS dominate the types of waste burned, most likely from the burning of scrap furniture. By assuming that on a mass basis the burned waste is 3 %–5 % of that of the firewood in both countries and taking into account that the total masses of firewood annually consumed in Hungary and Romania in the year 2019 were 7.4 and 12.2 million tonnes, respectively (Clean Air Action Group, Romanian Statistical Office), the calculations reveal that 8 %–13 % and 9 %–15 % of all household waste produced in Hungary and Romania, respectively, ended up being burned in household stoves. According to EUROSTAT (https://ec.europa.eu/eurostat/databrowser/view/ten00106/default/table?lang=en, last access: 2 February 2024) data, the amounts of household waste produced in Hungary and Romania were 2.7 and 4.1 million tonnes per year, respectively. These are alarming numbers given that such activities are prohibited in both countries and all over the EU.
The tracer compounds identified by Hoffer et al. (2021) for the burning of different waste types were quantified in atmospheric PM10 samples collected in different settlements in Hungary and Romania in the two consecutive heating seasons of 2019/2020 and 2020/2021. As a reference, PM10 samples were also collected in summer at all the locations. In spite of the fact that some tracers contain double bonds and therefore their long-term atmospheric stability is questionable, they were quantified in PM10 samples collected in winter but were missing from the summer samples. The relative contributions of PET burning emissions to those of wood burning were followed by the concentration ratio of 2-BEVT and LGS. The results showed that, in Hungary, large quantities of PET-containing waste are burned in the north-eastern part of the country. Although they can be emitted from other sources as well, the burning of PET was supported by the higher concentration ratios of the p-terphenyl and m-terphenyl isomers at those sites. Implications for the excessive burning of PET were also found in Romanian cities, where the burning of PET-containing waste was found to be on a similar scale to that in the most polluted north-eastern regions of Hungary. The styrene trimer (SSS) can be used as a tracer of the burning of furniture panels, household waste containing PS, and printed advertising and waxy papers, whereas melamine was used as a tracer of the burning of furniture panels and/or waste containing melamine–formaldehyde resin. Larger SSS emissions were observed in the capitals, possibly due to the burning of large quantities of scrap furniture panels as well as other types of styrene-containing household waste (such as printed and waxy papers of advertising leaflets). The presence of ASS, SSA, and SAS also implied the burning of furniture panels, as these compounds are emitted from the burning of furniture panel edge bands. The presence of quaterphenyls and triphenylbenzenes indicated burning of PET, RAG, and to a lesser extent PS, ABS, and furniture panels.
The estimated contributions of waste burning emissions to the mass concentration of ambient PM10 were of the order of a few percent estimated by taking into account the relative emission factors of waste burning tracers determined in controlled laboratory experiments. It is very important to emphasize that, considering the extremely high emission factors of PAHs and their toxicity equivalent from waste burning (Hoffer et al., 2020), this large mass contribution poses disproportionally higher health risks to the urban population. Using firewood consumption statistics and emission factors of levoglucosan (a tracer of wood burning) and the mass concentrations of waste burning tracers found in atmospheric PM10 samples implied that some 10 % of all household waste produced ended up being burned in household stoves during the heating season. While these estimates are loaded with very high uncertainties and can be regarded as back-of-the-envelope calculations only, it is crucial to underline that they prove beyond any doubt that the illegal burning of solid waste in household stoves is a common practice in both countries. Our findings call for the need for immediate and effective legislative measures against these activities posing extreme health risks to the population in both countries and all over Europe.
Data used in this study are available from the first author upon request (hoffer.andras@mk.uni-pannon.hu).
The supplement related to this article is available online at: https://doi.org/10.5194/acp-24-1659-2024-supplement.
EAL, LM, and AMa collected the aerosol samples and performed the gravimetric measurements. AH, AT, BJT, AMe, and GK performed and/or coordinated the analytical measurements and data evaluation. All the authors were involved in the scientific interpretation and discussion of the results as well as in the manuscript preparation. All the co-authors commented on the paper.
The contact author has declared that none of the authors has any competing interests.
Publisher's note: Copernicus Publications remains neutral with regard to jurisdictional claims made in the text, published maps, institutional affiliations, or any other geographical representation in this paper. While Copernicus Publications makes every effort to include appropriate place names, the final responsibility lies with the authors.
The work was also supported by the János Bolyai Research Scholarship of the Hungarian Academy of Sciences. The authors would like to thank the Institute for Environment and Energy, Technology and Analytics e.V. (IUTA) for providing the high-volume samplers used for the PM10 measurements in Romania.
This research has been supported by “Analysing the effect of residential solid waste burning on ambient air quality in central and eastern Europe and potential mitigation measures” (grant no. 07.027737/2018/788206/SER/ENV.C.3) and by the National Multidisciplinary Laboratory for Climate Change (project RRF-2.3.1-21-2022-00014).
This paper was edited by Ivan Kourtchev and reviewed by two anonymous referees.
Bodzay, B. and Bánhegyi, G.: Polymer waste: controlled breakdown or recycling?, Int. J. Des. Sci. Technol., 22, 109–138, 2016..
Caseiro, A., Bauer, H., Schmidl, C., Pio, C. A., and Puxbaum, H.: Wood burning impact on PM10 in three Austrian regions, Atmos. Environ., 43, 2186–2195, https://doi.org/10.1016/j.atmosenv.2009.01.012, 2009.
Christian, T. J., Yokelson, R. J., Cárdenas, B., Molina, L. T., Engling, G., and Hsu, S.-C.: Trace gas and particle emissions from domestic and industrial biofuel use and garbage burning in central Mexico, Atmos. Chem. Phys., 10, 565–584, https://doi.org/10.5194/acp-10-565-2010, 2010.
Fabbri, D., Torri, C., Simonei, B. R. T., Marynowski L., Rushdi A. I., and Fabianska M. J.: Levoglucosan and other cellulose and lignin markers in emissions from burning of Miocene lignites, Atmos. Environ., 43, 2286–2295, 2009.
Furman, P., Styszko, K., Skiba, A., Zieba, D., Zimnoch, M., Kistler, M., Kasper-Giebl, A., and Gilardoni, S.: Seasonal Variability of PM10 Chemical Composition Including 1,3,5-triphenylbenzene, Marker of Plastic Combustion and Toxicity in Wadowice, South Poland, Aerosol Air Qual. Res., 21, 200223, https://doi.org/10.4209/aaqr.2020.05.0223, 2021.
Hoffer, A., Jancsek-Turóczi, B., Tóth, Á., Kiss, G., Naghiu, A., Levei, E. A., Marmureanu, L., Machon, A., and Gelencsér, A.: Emission factors for PM10 and polycyclic aromatic hydrocarbons (PAHs) from illegal burning of different types of municipal waste in households, Atmos. Chem. Phys., 20, 16135–16144, https://doi.org/10.5194/acp-20-16135-2020, 2020.
Hoffer, A., Tóth, Á., Jancsek-Turóczi, B., Machon, A., Meiramova, A., Nagy, A., Marmureanu, L., and Gelencsér, A.: Potential new tracers and their mass fraction in the emitted PM10 from the burning of household waste in stoves, Atmos. Chem. Phys., 21, 17855–17864, https://doi.org/10.5194/acp-21-17855-2021, 2021.
Islam, M. R., Li, T., Mahata, K., Khanal, N., Werden, B., Giordano, M. R., Puppala, S. P., Dhital, N. B., Gurung, A., Saikawa, E., Panday, A. K., Yokelson, R. J., DeCarlo, P. F., and Stone, E. A.: Wintertime Air Quality across the Kathmandu Valley, Nepal: Concentration, Composition, and Sources of Fine and Coarse Particulate Matter, ACS Earth Sp. Chem., 6, 2955–2971, https://doi.org/10.1021/acsearthspacechem.2c00243, 2022.
Kantar Hoffmann Ltd.: Household waste burning habits in Hungary, https://www.levego.hu/sites/default/files/Kantar_Hoffmann_Levego_MCS_Hulladekegetes_2017dec.pdf last access: 20 February 2021 (in Hungarian).
Jimenez, J., Farias, O., Quiroz, R., and Yanez, J.: Emission factors of particulate matter, polycyclic aromatic hydrocarbons, and levoglucosan from wood combustion in south-central Chile, J. Air Waste Manag. Assoc., 67, 806–813, https://doi.org/10.1080/10962247.2017.1295114, 2017.
Jones, A. M. and Harrison, R. M.: Emission of ultrafine particles from the incineration of municipal solid waste: A review, Atmos. Environ., 140, 519–528, https://doi.org/10.1016/j.atmosenv.2016.06.005, 2016.
Kanellopoulos, P. G., Verouti, E., Chrysochou, E., Koukoulakis, K., and Bakeas, E.: Primary and secondary organic aerosol in an urban/industrial site: Sources, health implications and the role of plastic enriched waste burning, J. Environ. Sci., 99, 222–238, https://doi.org/10.1016/j.jes.2020.06.012, 2021.
Krahl, J., Seidel, H., Jeberien, H. E., Ruckert, M., and Bahadir, M.: Pilot study: PAH fingerprints of aircraft exhaust in comparison with diesel engine exhaust, Fresen. J. Anal. Chem., 360, 693–696, 1998.
Kumar, S., Aggarwal, S. G., Gupta, P. K., and Kawamura, K.: Investigation of the tracers for plastic-enriched waste burning aerosols, Atmos. Environ., 108, 49–58, https://doi.org/10.1016/j.atmosenv.2015.02.066, 2015.
Lemieux, P. M., Lutes, C. C., and Santoianni, D. A.: Emissions of organic air toxics from open burning: a comprehensive review, Prog. Energ. Combust., 30, 1–32, https://doi.org/10.1016/j.pecs.2003.08.001, 2004.
Li, B. and Fang, J.: The Detection and Environmental Significance of 5'Phenyl1, 1': 3',1”Terphenyl from the Atmosphere in Beijing, 3rd International Conference on Bioinformatics and Biomedical Engineering, iCBBE 2009, 11–16 June 2009, Beijing, China, https://doi.org/10.1109/ICBBE.2009.5163587, 2009.
Marmureanu, L., Vasilescu, J., Slowik, J., Prevot, A. S. H., Marin, C. A., Antonescu, B., Vlachou, A., Nemuc, A., Dandocsi, A., and Szidat, S.: Online Chemical Characterization and Source Identification of Summer and Winter Aerosols in Măgurele, Romania, Atmosphere, 11, 385, https://doi.org/10.3390/atmos11040385, 2020.
Ramadan, B. S., Rachman, I., Ikhlas, N., Kurniawan, S. B., Miftahadi, M. F., and Matsumoto, T.: A comprehensive review of domestic-open waste burning: recent trends, methodology comparison, and factors assessment, J. Mater. Cycles Waste, 24, 1633–1647, https://doi.org/10.1007/s10163-022-01430-9, 2022.
Salma, I., Németh, Z., Weidinger, T., Maenhaut, W., Claeys, M., Molnár, M., Major, I., Ajtai, T., Utry, N., and Bozóki, Z.: Source apportionment of carbonaceous chemical species to fossil fuel combustion, biomass burning and biogenic emissions by a coupled radiocarbon–levoglucosan marker method, Atmos. Chem. Phys., 17, 13767–13781, https://doi.org/10.5194/acp-17-13767-2017, 2017.
Simoneit, B. R. T., Medeiros, P. M., and Didyk, B. M.: Combustion products of plastics as indicators for refuse burning in the atmosphere, Environ. Sci. Technol., 39, 6961–6970, https://doi.org/10.1021/es050767x, 2005.
Wiedinmyer, C., Yokelson, R. J., and Gullett, B. K.: Global Emissions of Trace Gases, Particulate Matter, and Hazardous Air Pollutants from Open Burning of Domestic Waste, Environ. Sci. Technol., 48, 9523–9530, https://doi.org/10.1021/es502250z, 2014.
Zhao, W., Kawamura, K., Yue, S., Wei, L., Ren, H., Yan, Y., Kang, M., Li, L., Ren, L., Lai, S., Li, J., Sun, Y., Wang, Z., and Fu, P.: Molecular distribution and compound-specific stable carbon isotopic composition of dicarboxylic acids, oxocarboxylic acids and α-dicarbonyls in PM2.5 from Beijing, China, Atmos. Chem. Phys., 18, 2749–2767, https://doi.org/10.5194/acp-18-2749-2018, 2018.