the Creative Commons Attribution 4.0 License.
the Creative Commons Attribution 4.0 License.
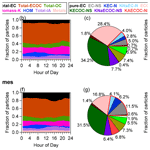
Mixing state and effective density of aerosol particles during the Beijing 2022 Olympic Winter Games
Aodong Du
Jiaxing Sun
Weiqi Xu
Yuting Zhang
Xubing Du
Yan Li
Xiaole Pan
Zifa Wang
Mixing state and density are two key parameters of aerosol particles affecting their impacts on radiative forcing and human health. Here a single-particle aerosol mass spectrometer in tandem with a differential mobility analyzer and an aerodynamic aerosol classifier was deployed during the Beijing 2022 Olympic Winter Games (OWG) to investigate the impacts of emission controls on particle mixing state and density. Our results show the dominance of carbonaceous particles comprising mainly total elemental carbon (Total-EC, 13.4 %), total organic carbon (Total-OC, 10.5 %) and Total-ECOC (47.1 %). Particularly, the particles containing organic carbon and sulfate were enhanced significantly during OWG, although those from primary emissions decreased. The composition of carbonaceous particles also changed significantly which was characterized by the decreases in EC mixed with nitrate and sulfate (EC-NS), EC mixed with potassium nitrate (KEC-N), and amine-containing particles and increase in ECOC mixed with nitrate and sulfate (ECOC-NS). This result indicates that emission controls during OWG reduced the mixing of EC with inorganic aerosol species and amines yet increased the mixing of EC with organic aerosol. The average effective density (ρeff) of aerosol particles (150–300 nm) was 1.15 g cm−3 during the non-Olympic Winter Games (nOWG), with higher values during OWG (1.26 g cm−3) due to the increase in secondary particle contribution. In addition, the ρeff of most particles increased with the increases in pollution levels and relative humidity, yet they varied differently for different types of particles, highlighting the impacts of aging and formation processes on the changes of particle density and mixing state.
- Article
(6630 KB) - Full-text XML
-
Supplement
(1183 KB) - BibTeX
- EndNote
Atmospheric aerosols from both natural and anthropogenic sources exert strong influences on radiative forcing and human health (Buseck and Posfai, 1999; Anderson et al., 2003; Ramanathan et al., 2001; Prather, 2009), and the impacts depend strongly on their chemical and physical properties, e.g., composition, mixing state, and density. Beijing, experiencing severe pollution with high concentrations of fine particulate matter over the past decade (Huang et al., 2014; Guo et al., 2014; Sun et al., 2016), has had great success in air pollution control (Lei et al., 2021a; Cheng et al., 2019), and the annual average concentration of PM2.5 reached the Chinese National Ambient Air Quality Standard for the first time in 2021 (33 µg m−3). However, polluted events still occurred occasionally, particularly during wintertime with stagnant meteorological conditions and high anthropogenic emissions (Lei et al., 2021b; Xu et al., 2022; Feng et al., 2022; Zhou et al., 2023). Short-term emission controls can improve air quality by reducing anthropogenic emissions temporarily, for example, the Beijing 2008 Summer Olympics (Wang et al., 2010; Okuda et al., 2011; Zhou et al., 2010), the 2014 Asia-Pacific Economic Cooperation (APEC) summit (Han et al., 2015; Gao et al., 2017; Ren et al., 2018), and the 2020 lockdown due to the corona virus disease (COVID-19) (Sun et al., 2020; Rajesh and Ramachandran, 2022; Zhang et al., 2022a). Similarly, the Chinese government imposed strict emission controls in Beijing and surrounding regions during the Olympic Winter Games (OWG) in Beijing in 2022. These measures included shutting down factories with high emissions, limiting the number of vehicles, stopping construction activities, and forbidding fireworks (The People's Government of Beijing Municipality, 2022). As a result, PM2.5 decreased significantly during OWG (Liu et al., 2022). Although the effects of emission controls on chemical composition and formation mechanisms of fine particles have been extensively studied, their impacts on chemical and physical properties of single particles, e.g., mixing state and effective density (ρeff), are poorly understood.
The single-particle aerosol mass spectrometer (SPAMS) has been widely used to characterize mixing state, chemical compositions and formation mechanisms of aerosol particles (Bhave et al., 2002; Giorio et al., 2015; Li et al., 2014; Bi et al., 2011; Chen et al., 2017; Zhang et al., 2018). Previous single-particle studies in China were mainly conducted in polluted winters. Chen et al. (2020) found that carbonaceous particles associated with coal combustion were the main contributors of fine particles during wintertime in urban and rural areas of Beijing. Zhang et al. (2022b) found that the proportion of elemental carbon particles mixed with organics gradually increased in winter from 2016 to 2019 in Chengdu. Similarly, elemental carbon particles tended to be more mixed with organics in winter in Beijing compared to summer (Xie et al., 2020). However, measurements of critical parameters of aerosol particles, e.g., effective density (ρeff) with different mixing states, are still limited (Buseck and Posfai, 1999; Pitz et al., 2003).
ρeff reflecting the average density and morphological characteristics of particles depends strongly on mixing state, sources, and aging levels (DeCarlo et al., 2004). To obtain ρeff, SPAMS is often deployed along with other instruments that can measure particle sizes as well. Spencer et al. (2007) deployed a differential mobility analyzer (DMA) and a SPAMS simultaneously and determined the ρeff of aerosol particles in the range of ∼1 to 1.5 g cm−3 in California in summer. Using the same system, Zhai et al. (2017) found that the ρeff of biomass combustion particles ranged from ∼1.16 to 1.51 g cm−3. Recently, an aerodynamic aerosol classifier (AAC) tandem SPAMS system was established by Peng et al. (2021) to determine the volume equivalent diameter (Dve) and ρeff of different types of aerosol particles. This system was then used to analyze the characteristics of particles emitted from diesel vehicle engines with different mixing states (Su et al., 2021). The results showed largely different ρeff for aerosol particles emitted under launching and idling conditions (0.66 vs. 0.34 g cm−3). Till now, few studies have characterized the ρeff of ambient aerosol particles at different mixing states using similar tandem systems, and the evaluation of the impacts of emission controls is rare as well.
In this study, a high-resolution SPAMS coupled with a DMA and an AAC, respectively, was deployed during OWG in Beijing to characterize the chemical and physical properties of aerosol particles. The chemical composition, mixing state, and ρeff are determined, and the differences between OWG and non-Olympic Winter Games (nOWG) are explored. Particularly, the influences of emission controls on fine particle characteristics and mixing state are elucidated.
2.1 Sampling site and measurements
All instruments were deployed at the Institute of Atmospheric Physics (IAP), Chinese Academy of Sciences (39∘58′28′′ N, 116∘22′16′′ E), an urban site influenced by both residential and traffic emissions. To measure aerosol particles according to density, two tandem systems by coupling a differential mobility analyzer (DMA; model 3085A, TSI Inc.) and a single-particle aerosol mass spectrometer (SPAMS; Hexin Analytical Instrument Co., Ltd.) and an aerodynamic aerosol classifier (AAC; Cambustion Ltd.) and a SPAMS, were operated from 21 January to 10 February and from 10 February to 1 March, respectively (Fig. 1). Aerosol particles were first filtered with a PM2.5 cyclone placed in front of the sampling line. After being dried with a diffusion dryer, the particles with mobility diameters (Dm) of 150, 200, 250, and 300 nm were selected by the DMA, and those with an aerodynamic diameter (Da) of 300 nm were selected by the AAC, and then the highly monodispersed particles were measured by the SPAMS. A detailed description of the AAC is given elsewhere (Liu et al., 2019, 2020; Yu et al., 2022). Before the campaign, the size calibration of the SPAMS was performed using polystyrene latex spheres (PSLs) with known sizes (0.23, 0.32, 0.51, 0.74, 0.96, 1.4, and 2 µm).
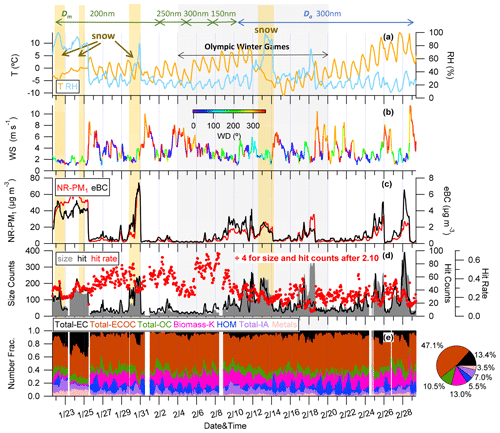
Figure 3Time series of (a) temperature (T) and relative humidity (RH), (b) wind speed (WS) colored by wind direction (WD; measured in degrees clockwise from due north), (c) mass concentrations of eBC and NR-PM1, (d) number of sized particles, hit particles, and the average hit rate of SPAMS per minute (both size and hit counts after 2.10 have been divided by 4 in order to show the temporal trend of the DMA-SPAMS period better), and (e) number fraction of seven major classes of particles including Total-EC, Total-ECOC, Total-OC, Total-IA, Biomass-K, HOM, and Metals. The pie chart shows the average number fraction of particles for the entire period. The green and blue arrows represent the sizes selected by DMA or AAC, respectively, while the yellow and gray shading corresponds to the snowfall and Olympic Winter Games periods, respectively.
A seven-wavelength (370, 470, 520, 590, 660, 880, and 950 nm) aethalometer (AE33, Magee Scientific Corp.) and a high-resolution time-of-flight aerosol mass spectrometer (HR-ToF-AMS) were deployed at the same site to measure equivalent black carbon (eBC) and non-refractory organics (Org), sulfate (SO4), nitrate (NO3), ammonium (NH4), and chloride (Chl) in PM1. The time resolution of both instruments was 1 min, where the HR-ToF-AMS measured in V mode, and the mass concentration of eBC obtained by AE33 was calculated based on the dual-spot measurement (Rajesh and Ramachandran, 2018; Drinovec et al., 2015). The HR-ToF-AMS data were analyzed using PIKA v 1.24, which showed that NO3 (4.30 µg m−3) and Org (3.80 µg m−3) contributed 68.0 % of the mass concentration of non-refractory submicron aerosols (NR-PM1, 11.92 µg m−3), followed by SO4 (1.91 µg m−3), NH4 (1.69 µg m−3), and Chl (0.22 µg m−3). Organic aerosols (OAs) were analyzed by positive matrix factorization (PMF), and five OA factors were identified including biomass burning and fossil fuel combustion-related OA (FFBBOA); cooking OA (COA); and three secondary OA (SOA) factors, i.e., two oxygenated OA (OOA1 and OOA2) and an aqueous-phase OOA, with mass concentrations of 0.31, 0.87, 0.83, 1.18, and 0.56 µg m−3, respectively. More details on the operations and data analysis of the AE33 and HR-ToF-AMS are given in Xu et al. (2023).
2.2 Data analysis
2.2.1 SPAMS
A total of 2 619 193 particles were sized, and approximately 760 000 particles with both mass spectra and size information were captured by the SPAMS (Table S1 in the Supplement). As shown in Fig. S1 in the Supplement, the counts of total particles correlated reasonably well with non-refractory submicron aerosols (NR-PM1, r=0.76), and those of EC-containing particles correlated well with eBC (r=0.87). The data were then analyzed using a neural network algorithm based on adaptive resonance theory (ART-2a) (Song et al., 1999). The vigilance factor, learning rate, and iterations for ART-2a were set as 0.75, 0.05, and 20, respectively, in this study. According to the mass spectral characteristics, temporal trends, and size distributions (Dall'osto and Harrison, 2006; Phares et al., 2001), more than 99 % of the total particles were grouped into seven major classes including total elemental carbon (Total-EC), total organic carbon (Total-OC), Total-ECOC, total inorganic aerosol (Total-IA), potassium-rich particles from biomass burning (Biomass-K), high-molecular-weight organic matter (HOM), and metals, as well as several subclasses (Table 1). The classification of particles is based on three principles: (1) particles with distinct (n=1, 2, 3…) signals are named EC, (2) particles with distinct OC signals (including 27[C2H3]+, 37[C3H]+, 43[C2H3O]+, 50[C4H2]+, 51[C4H3]+, …) are named OC, and (3) particles are named ECOC when they have comparable EC and OC signals in the positive spectra (Sun et al., 2022a, b). Detailed names of particle types and characteristics are given in Table S2, and the average mass spectra of each type of particle are depicted in Figs. 2 and S2.
2.2.2 Effective density
Given that the DMA and AAC select the mobility diameter (Dm) and aerodynamic diameter (Da) of particles, respectively, two methods are adopted to calculate the ρeff in this study. For the DMA–SPAMS tandem system, the ρeff is determined as the ratio of vacuum aerodynamic diameter (Dva) to Dm as Eq. (1).
where ρ0 is the standard density (1.0 g cm−3).
For the AAC–SPAMS tandem system, the ρeff is calculated as the ratio of particle density (ρp) and particle dynamic shape factor (χγ):
where Dve represents the volume equivalent diameter. A more detailed description of the relationship between Da, Dva, and Dve is given in DeCarlo et al. (2004). Combining the calculated ρeff with the particle mixing states measured by the SPAMS makes it possible to analyze the ρeff of particles with different compositions.
3.1 Mixing state of aerosol particles
Figure 3 depicts the time series of meteorological parameters, pollutant concentrations, and the number fractions of seven types of particles during the entire study. The average (±1σ) mass concentrations of NR-PM1 and eBC for the entire study were 11.92 (±15.77) and 1.34 (±1.41) µg m−3, respectively, and they decreased by 48.7 % and 37.5 % during OWG compared with the nOWG period (Table 2). In addition to emission controls, the favorable meteorological conditions during OWG as indicated by lower RH and T and higher WS also played a role in the low concentrations (Liu et al., 2022).
Figure S3 shows the digital mass spectra of all particles for the whole period. Significant ion peaks of organic and carbon clusters were observed in positive spectra and nitrate (46[NO2]− and 62[NO3]−) and sulfate (97[HSO4]−) in negative spectra. This was consistent with the AMS measurements that nitrate and organics were the major components of NR-PM1, on average accounting for 36.1 % and 31.9 %, respectively, followed by sulfate (16.0 %). The differences in the relative peak areas of aerosol particles between OWG and nOWG periods were also observed (Fig. S3b). The signals of 39[K]+ and organic carbon (27[C2H3]+, 43[C2H3O]+, 50[C4H2]+) and 97[HSO4]− were significantly enhanced during OWG, suggesting the increased importance of organic aerosol and sulfate. In addition, we found that the ratio of peak area of sulfate to nitrate (PAsulfate PAnitrate) during OWG (0.26) was slightly higher than that during nOWG (0.24), suggesting the elevated aging of aerosol particles. In fact, the daily captured particle counts from the SPAMS show that aging particles become more prominent during the OWG period due to the combination of the significant reduction in the number concentration of primary particles (e.g., Total-EC decreased by 61.80 %) and the marked increase in the number concentration of some aging particles, such as ECOC mixed with nitrate and sulfate (ECOC-NS, 17.34 % increase), during the OWG period (Table S3).
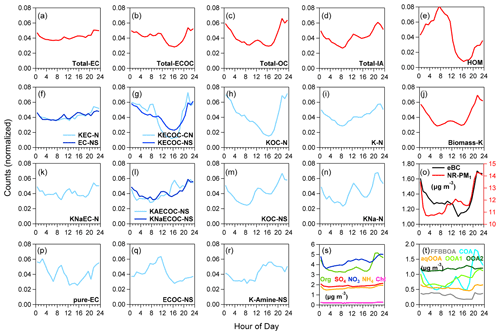
Figure 5Diurnal cycles of (a–n, p–r) normalized counts for each class of particles, (o) mass concentrations of eBC and NR-PM1, and (s) NR-PM1 species, and (t) OA factors.
The Total-ECOC particles with intense ion peaks of elemental carbon (EC, , n=1, 2, 3…) and organic carbon (OC, 27[C2H3]+, 37[C3H]+, 43[C2H3O]+, 50[C4H2]+, 51[C4H3]+…) represented 47.1 % of the total particles, followed by Total-EC (13.4 %) and Biomass-K (13.0 %). The carbonaceous particles accounting for 71.0 % of the total were divided into 12 subclasses (Table 1). These carbonaceous particles were overall mixed with nitrate (relative peak area ∼0.4) or sulfate (∼0.05–0.2) and showed pronounced 39[K]+, 23[Na]+ or 18[NH4]+ signals in the positive spectra. We observed a clear decrease in Total-EC from 11.5 % to 8.5 % during OWG, while there were increased contributions for Total-ECOC and Biomass-K by 3.3 % and 2.2 %, respectively. These results indicate the changes of mixing state of aerosol particles during OWG. Most importantly, the composition of carbonaceous particles also changed significantly. The largest decreases were observed for EC mixed with nitrate and sulfate (EC-NS) and EC mixed with potassium nitrate (KEC-N) by 5.5 % and 3.4 %, respectively, while the proportion of ECOC-NS increased significantly from 16.8 % to 28.4 %. In addition, the ammonium-containing (18[NH4]+) and trimethylamine-containing (58[C3H8N]+ and 59[C3H9N]+) particles were largely reduced from 8.7 % to 1.1 % during OWG. Such results indicate that emission controls during OWG reduced the mixing of EC with inorganic aerosol species and amines yet increased the mixing of EC with organic aerosol.
The Biomass-K particles were identified with intense 39[K]+ and levoglucosan ion peaks (45[CHO2]−, 59[C2H3O2]−, 71[C3H3O]−, and 73[C3H5O2]−). Pratt et al. (2011) found that levoglucosan can degrade rapidly due to atmospheric oxidation. Therefore, the contribution of 13.0 % in this measurement indicated that the Biomass-K particles may undergo atmospheric oxidation processes to some extent. A class of high-molecular-weight organic matter (HOM) characterized by distinct polycyclic aromatic hydrocarbons (PAHs), e.g., 152[C12H8]+, 165[C13H9]+, 178[C14H10]+, and 189[C15H9]+, was also detected (Zhang et al., 2022c), accounting for 5.5 % of the total particles. The prominent nitrate (46[NO2]− and 62[NO3]−) signals in negative spectra indicated the mix of HOM particles with secondary inorganic aerosol species (Fig. 2e). In addition, the SPAMS also detected some relatively pure inorganic aerosol (IA) particles that did not mix with other components and metal particles (55[Mn]+, 56[Fe]+ and 206,207,208[Pb]+) that were likely from anthropogenic emission and road dust. A large number of rich-Fe particles with peak area ratio 56[Fe]+/54[Fe], accounting for 59.1 % of Metals particles, were mainly observed during periods with snowfall events and were strongly associated with secondary species especially nitrate (Fig. S2). Total-IA and Metals accounted for 7.0 % and 3.5 % of the total particles, respectively, both of which showed decreased contributions during OWG, indicating the suppressed local secondary formation due to reduced precursors and the effect of stopping construction activities.
3.2 Diurnal cycles and sources
Figure 5 depicts the diurnal variations of the normalized counts of different types of particles throughout the campaign. Particles including KECOC-NS and KOC-N showed similar diurnal cycles, with the lowest values occurring at 16:00 (all times are given in local time throughout the paper) and high values at night. The high correlations of these particles with primary OA from fossil fuel combustion and biomass burning (r=0.78 and 0.70, Table S4) highlight their dominant sources of primary emissions. Consistently, the dominant contribution (OWG vs. nOWG: 48.1 % vs. 47.2 %) of KECOC-NS and KOC-N to the carbonaceous particles at low PAsulfate PAnitrate (<0.2), an indicator of particle aging level (Li et al., 2020), supported the properties of fresh emissions as well (Fig. 6). The bivariate polar plots (Fig. S4) indicated that these two types of particles were mainly transported from the southeast during OWG. This result suggests that fresh particles in winter in Beijing could also be from regional transport over a small scale under emission control. Previous studies have demonstrated that particles with significant organic nitrogen fragments (26[CN]− and 42[CNO]−) and 39[K]+ signals may come from wildfires, biomass burning, and coal combustion (Pratt et al., 2011; Zauscher et al., 2013; J. Hu et al., 2021; Bi et al., 2011). Considering the strict emission controls in Beijing during OWG, the higher normalized count of KECOC-CN at night was likely attributed to the regional transport of primarily emitted particles near Beijing (Fig. S4). However, the low peak area ratio of EC to OC (PAEC PAOC) for ECOC-containing particles indicated overall higher aging levels during OWG (Fig. 7e-j) (Pio et al., 2011; Pokhrel et al., 2016). Particularly, the PAEC PAOC of KECOC-NS and KECOC-CN decreased obviously from 1.01 to 0.82 and 0.92 to 0.80, respectively, during OWG.
The pronounced diurnal cycle of HOM indicated the sources of coal combustion and traffic emissions from heavy duty vehicles and diesel trucks and the transport from the southeast (Fig. S4), which reached a maximum weight in total particles at around 8:00 (11.4 % for OWG and 10.2 % for nOWG; Fig. 4). A pronounced diurnal cycle with high values in the morning (∼11:00) was also observed for ECOC-NS, likely indicating the similar sources as HOM, yet the strong 97[HSO4]− signal in mass spectra suggested more aged properties. The moderate correlation (r=0.63) between ECOC-NS and HOM and the bivariate polar plots, especially during nOWG (Fig. S4b), also supported this conclusion. In fact, the contribution of ECOC-NS particles is significant across different PAsulfate PAnitrate values, demonstrating the complexity of its sources (Fig. 6b, d).
In addition, KNa-containing carbonaceous particles are generally considered to be from the incomplete combustion of solid fuels such as coal combustion and traffic emissions (Xie et al., 2020; J. Hu et al., 2021; Li et al., 2018). KNaEC-N and KNaECOC-NS were significantly mixed with nitrate (Fig. S2). The high correlations with FFBBOA and chloride (Table S4) and the bivariate polar plots of the particles emphasized the features of local emissions.
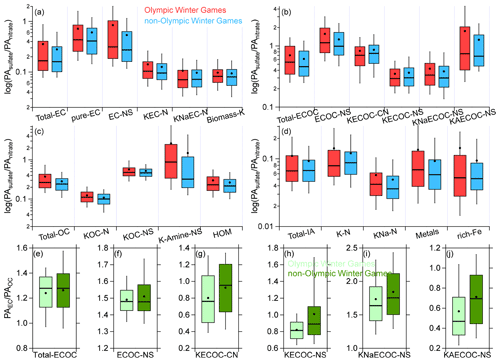
Figure 7Peak area ratios of (a–d) sulfate ( −80 and −97) to nitrate ( −46 and −62) for each type of particles and (e–j) elemental carbon ( , n=1–5) to organic carbon ( 27, 29, 37 and 43) in ECOC-containing particles during OWG and nOWG. Also shown are the median (horizontal lines), mean (circles), 25th and 75th percentiles (lower and upper boxes), and 10th and 90th percentiles (lower and upper whiskers).
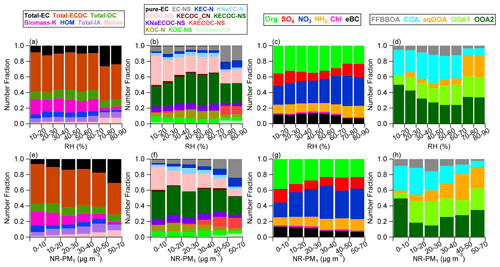
Figure 8Variations of the number fractions and mass fractions of aerosol particles and species as a function of (a–d) relative humidity and (e–h) NR-PM1 mass concentrations.
The types of EC mixed with potassium nitrate (KEC-N); EC mixed with nitrate and sulfate (EC-NS); ECOC mixed with nitrate, sulfate, and ammonium (KAECOC-NS); potassium-rich organic amine (K-Amine-NS); and potassium nitrate (K-N) particles were closely associated with three SOA factors (Table S4). KEC-N and EC-NS accounted for ∼75.4 % of Total-EC, with relatively small daily variations (Fig. 5f) and greater contribution in the afternoon, which was particularly evident during nOWG (Fig. 4d, h). This result might indicate the background or regional characteristics of these particles, which was consistent with the conclusion of Dall'osto et al. (2016). Interestingly, the contribution of Total-EC to the total particles decreased from 15.4 % to 5.5 % with the increase in PAsulfate PAnitrate from 0 to 1.1 during nOWG (Fig. 6c), whereas the change of Total-EC contribution was relatively flat (from 10.3 % to 7.0 %) during OWG (Fig. 6a). This was mainly attributed to the emission control which led to a decrease in the proportion of EC-containing particles at low aging levels. The minimum daily values of both KAECOC-NS and K-N particles appeared at 08:00 and gradually increased thereafter (Fig. 5i, l). Chen et al. (2020) suggested that the increase in K-N was mainly achieved through the uptake of nitrate from daytime photochemical production. KAECOC-NS particles characterized by high ammonium (18[NH4]+) signal were mainly observed during polluted periods with snowfall. Similarly, K-Amine-NS particles characterized by trimethylamine ion fragments (58[C3H8N]+ and 59[C3H9N]+) (Bhave et al., 2002; Sodeman et al., 2005; Angelino et al., 2001) showed rapid increases in number concentrations with the increase in RH (Zhong et al., 2022). Therefore, K-Amine-NS was most likely from the aging process of primarily emitted particles (e.g., traffic emissions) or mixing with secondary components during snowfall periods (Zhong et al., 2022; Cheng et al., 2018), while it was mainly relevant to regional transport during other periods (Angelino et al., 2001; Chen et al., 2019, 2020). As typical secondary particles, K-Amine-NS (135.9 % increase; Fig. 7) and KAECOC-NS (38.8 %) showed pronounced increases with the increase in PAsulfate PAnitrate during OWG.
Over 70 % of rich-Fe particles were captured during polluted periods with high humidity (∼83 % on average) and occasional snowfall. Aerosol acidification associated with urban pollutants has been well documented to play a substantial role in increasing the solubility of Fe-containing particles (Rubasinghege et al., 2010; Baker and Croot, 2010; Hand et al., 2004; Zhang et al., 2014). Thus, rich-Fe particles were related to the mixing of Fe-containing particles from anthropogenic emissions (e.g., vehicle, coal combustion) with acidic salts under high relative humidity conditions (Zhu et al., 2022).
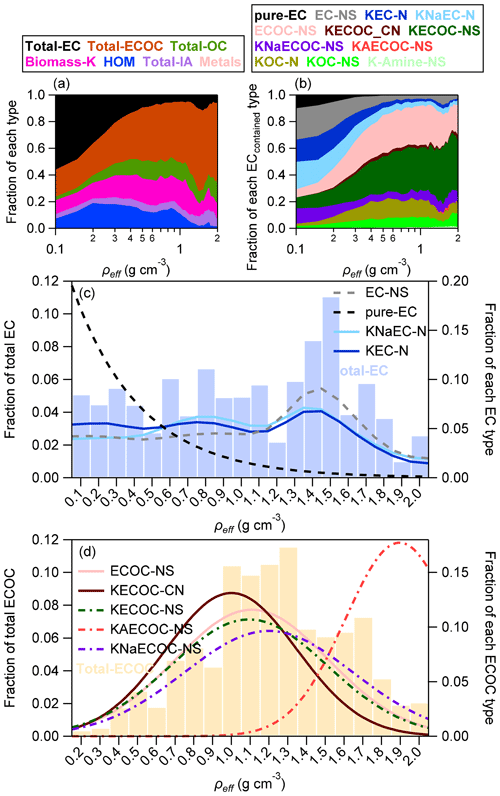
Figure 9Average contributions of (a) major types and (b) carbonaceous particles as a function of ρeff. The distributions of ρeff for (c) Total-EC and (d) Total-ECOC particles. The left y axis applies to the column diagram, and the right y axis applies to the density fitting curves.
The diurnal variation of KNa-N particles showed a clear bimodal character with high values peaking at 12:00 and 21:00 (Fig. 5n), which was similar to that of COA. This result indicates that KNa-N particles are mainly from cooking fume exhaust and photochemical processes (See and Balasubramanian, 2008; Abdullahi et al., 2013; Ito et al., 2016). Similarly, KOC-NS exhibited distinct cooking characteristics and good correlation with COA (r=0.72), suggesting the sources of diverse meat cooking emissions, especially Chinese-style cooking (He et al., 2004; Zhao et al., 2007).
3.3 Effective density of aerosol particles
The average ρeff of aerosol particles for all measured sizes was 1.20 g cm−3, with a higher value during OWG than nOWG (1.26 vs. 1.15 g cm−3; Fig. S5a), and the ρeff varied largely for different types of particles. The highly aged KAECOC-NS particles showed the highest ρeff of 1.62 g cm−3 (Fig. 10j), with an abrupt increase in particle counts during snowfall and high RH periods (>70 %; Fig. 8b), while the average ρeff of pure-EC and HOM associated with fresh primary emissions were 0.36 and 0.87 g cm−3, respectively. The low ρeff of HOM particles associated with traffic and coal combustion and of those containing fragments of PAHs is also attributed to their loose structure. As shown in Fig. S5, the ρeff was characterized by a clear Gaussian distribution, with the peak located at 1.3 g cm−3. The ρeff was proportional to ambient particle size (r2=0.93; Fig. S5b), which was distinct from that of fresh vehicle emissions studied by Su et al. (2021). In addition, it was found by comparing the ρeff of particles in different periods that the majority of particles have higher ρeff during the OWG period, with the most pronounced changes in EC-NS (OWG vs. nOWG: 1.10 vs. 0.99 g cm−3) and ECOC-NS (1.22 vs. 1.15 g cm−3) (Fig. S6). The average ρeff of fresh and fractal-structured pure-EC particles was the same (0.36 g cm−3) in different periods. The ρeff values of the primary emitted KECOC-NS (1.31 vs. 1.30 g cm−3) and KOC-N (1.03 vs. 1.00 g cm−3), which are closely related to the FFBBOA (Table S4) and have similar daily trends, do not change significantly between the two periods either. Irregular primary particles with low densities mix with other compositions into more compact spherical structures after being emitted into the atmosphere (Liu et al., 2019; D. Hu et al., 2021).
We further analyzed the distribution of Total-EC and Total-ECOC particles as a function of ρeff, which contributed 56.4 % and 59.1 % at low and high ρeff respectively (Fig. 9a). All EC classes except pure-EC showed bimodal distribution characteristics (Fig. 9c), including porous aggregates with low ρeff peaking at 0.8 g cm−3 and dense particles with higher ρeff peaking at 1.4 g cm−3. The results are consistent with the conclusions of previous studies (Rissler et al., 2014; Liu et al., 2019; Ma et al., 2020; Hu et al., 2022). The low ρeff of pure-EC (0.36 g cm−3 on average) suggested the presence of fresh irregularly shaped EC particles from fuel combustion. For instance, the ρeff was comparable to that of diesel exhaust particles (0.25 g cm−3; Qiu et al., 2014), fresh soot particles (0.39 g cm−3; Rissler et al., 2014), and propane flame particles (0.18 g cm−3; Xue et al., 2009). We also observed a considerable fraction of KNaECOC-NS (11.6 %) at low ρeff (<0.2 g cm−3), indicating the major sources of solid fuel combustion. Comparably, ECOC-NS and KECOC-NS dominated the total carbonaceous particles (61.7 %) during periods with high ρeff (>1 g cm−3).
The temporal variations of ρeff are substantial due to the changes in chemical composition and particle mixing state but overall in the range of 0.7−1.7 g cm−3 for 77 % particles. As shown in Fig. 10a–e, the ρeff of most particles was relatively stable throughout the day, with slightly higher values at ∼16:00. Pure-EC was significantly different from the other particles with the ρeff of only 0.27 g cm−3 at 10:00. This was attributed to the large amount of fresh elemental carbon particles during the morning rush hour. HOM, another class of particles related to fossil fuel combustion, showed overall low ρeff (∼0.90 g cm−3) yet with a clear increase during daytime.
The PM dependence of ρeff is shown in Fig. 10. It is clear that the ρeff of almost all types of aerosol particles increased as a function of PM levels. For example, the average ρeff of Total-EC increased from 1.01 g cm−3 during clean periods (NR-PM1 <10 µg m−3) to 1.28 g cm−3 during polluted periods (NR-PM1>50 µg m−3), indicating that the formation process of pollution also led to the changes in both aerosol composition and particle density. Carbonaceous particles contributed up to 79.8 % of the total particles during the polluted period (Fig. 8e), with the most abundant EC-NS and KECOC-NS particles having an average density of 1.18 and 1.70 g cm−3 (NR-PM1>50 µg m−3), respectively. The changes of ρeff as a function of RH were similar considering that severe pollution in winter was generally associated with high RH. We noticed that the average ρeff was minimal during periods with RH = 40 %–50 %, which was 1.09 g cm−3, coincident with the period with NR-PM1 in the range of 20–30 µg m−3. The proportion of primary OA in total OA in this case was considerable (47.7 % and 40.0 %; Fig. 8d, h), indicating that the appearance of low ρeff was mainly caused by fresh particles at moderate RH and PM concentration. The high ρeff (1.36 g cm−3) corresponding to RH above 70 % was mainly associated with the pollution during snowfall and the formation of secondary OA, which accounted for 85.5 % of OA (Fig. 8d). Particularly, the ρeff of K-Amine-NS increased by more than 30 % with the increase in RH, highlighting the formation of N-containing particles through aqueous-phase processing and the change of particle density as well (Zhong et al., 2022).
This study provides a detailed analysis of chemical composition, mixing state, and effective density of ambient aerosol particles during Olympic Winter Games in Beijing using a DMA/AAC+HR–SPAMS tandem system. Nearly 760 000 particles were classified into seven major classes including Total-EC (13.4 %), Total-ECOC (47.1 %), Total-OC (10.5 %), Total-IA (7.0 %), Biomass-K (13.0 %), HOM (5.5 %), and Metals (3.5 %). A total of 71.0 % of particles were found to be carbonaceous particles mixed primarily with sulfate and nitrate. The emission controls during OWG led to decreases in the types of Total-EC particles from 11.5 % to 8.5 % yet increases in aged and regional particles, e.g., ECOC-NS. The average effective density of aerosol particles between 150 and 300 nm was 1.20 g cm−3, with higher values during OWG (1.26 g cm−3 vs. 1.15 g cm−3 for nOWG). The Total-EC particles dominated the total particles at low effective densities (56.4 %), and the effective densities of EC class particles except pure-EC showed a bimodal distribution peaking at 0.8 and 1.4 g cm−3, respectively. Comparatively, the fresh pure-EC showed much lower density, with an average effective density of 0.36 g cm−3. The effective density varies largely depending on the particle types, secondary formation, and the changes in RH. Overall, high effective densities usually occur during the periods with high PM (NR-PM1>50 µg m−3) and RH (>70 %), highlighting the impact of aging processes on the effective density of aerosol particles. It is worth mentioning that due to the different sensitivities of the ionizing laser of SPAMS for the detection of different chemical compositions, its capability of quantitative analysis needs to be further evaluated in future studies.
The data in this study are available from the authors upon request (sunyele@mail.iap.ac.cn).
The supplement related to this article is available online at: https://doi.org/10.5194/acp-23-13597-2023-supplement.
YS and AD designed the research. AD, HL, WX, YZ, JS, YL, and WZ conducted the measurements and experiments. AD, WX, HL, YZ, and WZ analyzed the data. LL, XD, XP, and ZW reviewed and commented on the paper. AD, YS, and JS wrote the paper.
The contact author has declared that none of the authors has any competing interests.
Publisher’s note: Copernicus Publications remains neutral with regard to jurisdictional claims in published maps and institutional affiliations.
This research has been supported by the National Natural Science Foundation of China (grant no. 92044301).
This paper was edited by Jianping Huang and reviewed by two anonymous referees.
Abdullahi, K. L., Delgado-Saborit, J. M., and Harrison, R. M.: Emissions and indoor concentrations of particulate matter and its specific chemical components from cooking: A review, Atmos. Environ., 71, 260–294, https://doi.org/10.1016/j.atmosenv.2013.01.061, 2013.
Anderson, T. L., Charlson, R. J., Schwartz, S. E., Knutti, R., Boucher, O., Rodhe, H., and Heintzenberg, J.: Climate forcing by aerosols: a hazy picture, Science, 300, 1103–1104, https://doi.org/10.1126/science.1084777, 2003.
Angelino, S., Suess, D. T., and Prather, K. A.: Formation of Aerosol Particles from Reactions of Secondary and Tertiary Alkylamines: Characterization by Aerosol Time-of-Flight Mass Spectrometry, Environ. Sci. Technol., 35, 3130–3138, https://doi.org/10.1021/es0015444 2001.
Baker, A. R. and Croot, P. L.: Atmospheric and marine controls on aerosol iron solubility in seawater, Mar. Chem., 120, 4–13, https://doi.org/10.1016/j.marchem.2008.09.003, 2010.
Bhave, P. V., Allen, J. O., Morrical, B. D., Fergenson, D. P., Cass, G. R., and Prather, K. A.: A Field-Based Approach for Determining ATOFMS Instrument Sensitivities to Ammonium and Nitrate, Environ. Sci. Technol., 36, 4868–4879, https://doi.org/10.1021/es015823i 2002.
Bi, X., Zhang, G., Li, L., Wang, X., Li, M., Sheng, G., Fu, J., and Zhou, Z.: Mixing state of biomass burning particles by single particle aerosol mass spectrometer in the urban area of PRD, China, Atmos. Environ., 45, 3447–3453, https://doi.org/10.1016/j.atmosenv.2011.03.034, 2011.
Buseck, P. R. and Posfai, M.: Airborne minerals and related aerosol particles: effects on climate and the environment, P. Natl. Acad. Sci. USA, 96, 3372–3379, https://doi.org/10.1073/pnas.96.7.3372, 1999.
Cheng, C., Huang, Z., Chan, C. K., Chu, Y., Li, M., Zhang, T., Ou, Y., Chen, D., Cheng, P., Li, L., Gao, W., Huang, Z., Huang, B., Fu, Z., and Zhou, Z.: Characteristics and mixing state of amine-containing particles at a rural site in the Pearl River Delta, China, Atmos. Chem. Phys., 18, 9147–9159, https://doi.org/10.5194/acp-18-9147-2018, 2018. .
Chen, Y., Wenger, J. C., Yang, F., Cao, J., Huang, R., Shi, G., Zhang, S., Tian, M., and Wang, H.: Source characterization of urban particles from meat smoking activities in Chongqing, China using single particle aerosol mass spectrometry, Environ. Pollut., 228, 92–101, https://doi.org/10.1016/j.envpol.2017.05.022, 2017.
Chen, Y., Tian, M., Huang, R.-J., Shi, G., Wang, H., Peng, C., Cao, J., Wang, Q., Zhang, S., Guo, D., Zhang, L., and Yang, F.: Characterization of urban amine-containing particles in southwestern China: seasonal variation, source, and processing, Atmos. Chem. Phys., 19, 3245–3255, https://doi.org/10.5194/acp-19-3245-2019, 2019.
Chen, Y., Cai, J., Wang, Z., Peng, C., Yao, X., Tian, M., Han, Y., Shi, G., Shi, Z., Liu, Y., Yang, X., Zheng, M., Zhu, T., He, K., Zhang, Q., and Yang, F.: Simultaneous measurements of urban and rural particles in Beijing – Part 1: Chemical composition and mixing state, Atmos. Chem. Phys., 20, 9231–9247, https://doi.org/10.5194/acp-20-9231-2020, 2020.
Cheng, J., Su, J., Cui, T., Li, X., Dong, X., Sun, F., Yang, Y., Tong, D., Zheng, Y., Li, Y., Li, J., Zhang, Q., and He, K.: Dominant role of emission reduction in PM2.5 air quality improvement in Beijing during 2013–2017: a model-based decomposition analysis, Atmos. Chem. Phys., 19, 6125–6146, https://doi.org/10.5194/acp-19-6125-2019, 2019.
Dall'Osto, M. and Harrison, R.: Chemical characterisation of single airborne particles in Athens (Greece) by ATOFMS, Atmos. Environ., 40, 7614–7631, https://doi.org/10.1016/j.atmosenv.2006.06.053, 2006.
Dall'Osto, M., Beddows, D. C. S., McGillicuddy, E. J., Esser-Gietl, J. K., Harrison, R. M., and Wenger, J. C.: On the simultaneous deployment of two single-particle mass spectrometers at an urban background and a roadside site during SAPUSS, Atmos. Chem. Phys., 16, 9693–9710, https://doi.org/10.5194/acp-16-9693-2016, 2016.
DeCarlo, P. F., Slowik, J. G., Worsnop, D. R., Davidovits, P., and Jimenez, J. L.: Particle Morphology and Density Characterization by Combined Mobility and Aerodynamic Diameter Measurements. Part 1: Theory, Aerosol Sci. Tech., 38, 1185–1205, https://doi.org/10.1080/027868290903907, 2004.
Drinovec, L., Močnik, G., Zotter, P., Prévôt, A. S. H., Ruckstuhl, C., Coz, E., Rupakheti, M., Sciare, J., Müller, T., Wiedensohler, A., and Hansen, A. D. A.: The ”dual-spot” Aethalometer: an improved measurement of aerosol black carbon with real-time loading compensation, Atmos. Meas. Tech., 8, 1965–1979, https://doi.org/10.5194/amt-8-1965-2015, 2015.
Feng, Z., Liu, Y., Zheng, F., Yan, C., Fu, P., Zhang, Y., Lian, C., Wang, W., Cai, J., Du, W., Chu, B., Wang, Y., Kangasluoma, J., Bianchi, F., Petäjä, T., and Kulmala, M.: Highly oxidized organic aerosols in Beijing: Possible contribution of aqueous-phase chemistry, Atmos. Environ., 273, 118971, https://doi.org/10.1016/j.atmosenv.2022.118971, 2022.
Gao, M., Liu, Z., Wang, Y., Lu, X., Ji, D., Wang, L., Li, M., Wang, Z., Zhang, Q., and Carmichael, G. R.: Distinguishing the roles of meteorology, emission control measures, regional transport, and co-benefits of reduced aerosol feedbacks in “APEC Blue”, Atmos. Environ., 167, 476–486, https://doi.org/10.1016/j.atmosenv.2017.08.054, 2017.
Giorio, C., Tapparo, A., Dall'Osto, M., Beddows, D. C., Esser-Gietl, J. K., Healy, R. M., and Harrison, R. M.: Local and regional components of aerosol in a heavily trafficked street canyon in central London derived from PMF and cluster analysis of single-particle ATOFMS spectra, Environ. Sci. Technol., 49, 3330-3340, https://doi.org/10.1021/es506249z, 2015.
Guo, S., Hu, M., Zamora, M. L., Peng, J., Shang, D., Zheng, J., Du, Z., Wu, Z., Shao, M., Zeng, L., Molina, M. J., and Zhang, R.: Elucidating severe urban haze formation in China, P. Natl. Acad. Sci. USA, 111, 17373–17378, https://doi.org/10.1073/pnas.1419604111, 2014.
Han, T., Xu, W., Chen, C., Liu, X., Wang, Q., Li, J., Zhao, X., Du, W., Wang, Z., and Sun, Y.: Chemical apportionment of aerosol optical properties during the Asia Economic Cooperation summit in Beijing, China, J. Geophys. Res.-Atmos., 120, 12281–12295, https://doi.org/10.1002/2015jd023918, 2015.
Hand, J. L., Mahowald, N. M., Chen, Y., Siefert, R. L., Luo, C., Subramaniam, A., and Fung, I.: Estimates of atmospheric-processed soluble iron from observations and a global mineral aerosol model: Biogeochemical implications, J. Geophys. Res., 109, D17205, https://doi.org/10.1029/2004jd004574, 2004.
He, L., Hu, M., Huang, X., Yu, B., Zhang, Y., and Liu, D.: Measurement of emissions of fine particulate organic matter from Chinese cooking, Atmos. Environ., 38, 6557–6564, https://doi.org/10.1016/j.atmosenv.2004.08.034, 2004.
Hu, D., Alfarra, M. R., Szpek, K., Langridge, J. M., Cotterell, M. I., Belcher, C., Rule, I., Liu, Z., Yu, C., Shao, Y., Voliotis, A., Du, M., Smith, B., Smallwood, G., Lobo, P., Liu, D., Haywood, J. M., Coe, H., and Allan, J. D.: Physical and chemical properties of black carbon and organic matter from different combustion and photochemical sources using aerodynamic aerosol classification, Atmos. Chem. Phys., 21, 16161–16182, https://doi.org/10.5194/acp-21-16161-2021, 2021.
Hu, J., Xie, C., Xu, L., Qi, X., Zhu, S., Zhu, H., Dong, J., Cheng, P., and Zhou, Z.: Direct Analysis of Soil Composition for Source Apportionment by Laser Ablation Single-Particle Aerosol Mass Spectrometry, Environ. Sci. Technol., 55, 9721–9729, https://doi.org/10.1021/acs.est.0c07983, 2021.
Hu, K., Liu, D. T., Tian, P., Wu, Y. Z., Li, S. Y., Zhao, D. L., Li, R. J., Sheng, J. J., Huang, M. Y., Ding, D. P., Liu, Q., Jiang, X. T., Li, Q., and Tao, J.: Identifying the Fraction of Core–Shell Black Carbon Particles in a Complex Mixture to Constrain the Absorption Enhancement by Coatings, Environ. Sci. Tech. Lett., 9, 272–279, https://doi.org/10.1021/acs.estlett.2c00060, 2022.
Huang, R., Zhang, Y., Bozzetti, C., Ho, K., Cao, J., Han, Y., Daellenbach, K. R., Slowik, J. G., Platt, S. M., Canonaco, F., Zotter, P., Wolf, R., Pieber, S. M., Bruns, E. A., Crippa, M., Ciarelli, G., Piazzalunga, A., Schwikowski, M., Abbaszade, G., Schnelle-Kreis, J., Zimmermann, R., An, Z., Szidat, S., Baltensperger, U., El Haddad, I., and Prevot, A. S.: High secondary aerosol contribution to particulate pollution during haze events in China, Nature, 514, 218–222, https://doi.org/10.1038/nature13774, 2014.
Ito, K., Johnson, S., Kheirbek, I., Clougherty, J., Pezeshki, G., Ross, Z., Eisl, H., and Matte, T. D.: Intraurban Variation of Fine Particle Elemental Concentrations in New York City, Environ. Sci. Technol., 50, 7517–7526, https://doi.org/10.1021/acs.est.6b00599, 2016.
Lei, L., Zhou, W., Chen, C., He, Y., Li, Z., Sun, J., Tang, X., Fu, P., Wang, Z., and Sun, Y.: Long-term characterization of aerosol chemistry in cold season from 2013 to 2020 in Beijing, China, Environ. Pollut., 268, 115952, https://doi.org/10.1016/j.envpol.2020.115952, 2021a.
Lei, L., Sun, Y., Ouyang, B., Qiu, Y., Xie, C., Tang, G., Zhou, W., He, Y., Wang, Q., Cheng, X., Fu, P., and Wang, Z.: Vertical Distributions of Primary and Secondary Aerosols in Urban Boundary Layer: Insights into Sources, Chemistry, and Interaction with Meteorology, Environ. Sci. Technol., 55, 4542–4552, https://doi.org/10.1021/acs.est.1c00479, 2021b.
Li, K., Ye, X., Pang, H., Lu, X., Chen, H., Wang, X., Yang, X., Chen, J., and Chen, Y.: Temporal variations in the hygroscopicity and mixing state of black carbon aerosols in a polluted megacity area, Atmos. Chem. Phys., 18, 15201–15218, https://doi.org/10.5194/acp-18-15201-2018, 2018.
Li, L., Li, M., Huang, Z., Gao, W., Nian, H., Fu, Z., Gao, J., Chai, F., and Zhou, Z.: Ambient particle characterization by single particle aerosol mass spectrometry in an urban area of Beijing, Atmos. Environ., 94, 323–331, https://doi.org/10.1016/j.atmosenv.2014.03.048, 2014.
Li, S., Zhang, F., Jin, X., Sun, Y., Wu, H., Xie, C., Chen, L., Liu, J., Wu, T., Jiang, S., Cribb, M., and Li, Z.: Characterizing the ratio of nitrate to sulfate in ambient fine particles of urban Beijing during 2018–2019, Atmos. Environ., 237, 117662, https://doi.org/10.1016/j.atmosenv.2020.117662, 2020.
Liu, H., Pan, X., Wu, Y., Ji, D., Tian, Y., Chen, X., and Wang, Z.: Size-resolved mixing state and optical properties of black carbon at an urban site in Beijing, Sci. Total Environ., 749, 141523, https://doi.org/10.1016/j.scitotenv.2020.141523, 2020.
Liu, H., Pan, X., Wu, Y., Wang, D., Tian, Y., Liu, X., Lei, L., Sun, Y., Fu, P., and Wang, Z.: Effective densities of soot particles and their relationships with the mixing state at an urban site in the Beijing megacity in the winter of 2018, Atmos. Chem. Phys., 19, 14791–14804, https://doi.org/10.5194/acp-19-14791-2019, 2019.
Liu, Y., Xu, X., Yang, X., He, J., Ji, D., and Wang, Y.: Significant Reduction in Fine Particulate Matter in Beijing during 2022 Beijing Winter Olympics, Environ. Sci. Tech. Lett., 9, 822–828, https://doi.org/10.1021/acs.estlett.2c00532, 2022.
Ma, Y., Huang, C., Jabbour, H., Zheng, Z., Wang, Y., Jiang, Y., Zhu, W., Ge, X., Collier, S., and Zheng, J.: Mixing state and light absorption enhancement of black carbon aerosols in summertime Nanjing, China, Atmos. Environ., 222, 117141, https://doi.org/10.1016/j.atmosenv.2019.117141, 2020.
Okuda, T., Matsuura, S., Yamaguchi, D., Umemura, T., Hanada, E., Orihara, H., Tanaka, S., He, K., Ma, Y., Cheng, Y., and Liang, L.: The impact of the pollution control measures for the 2008 Beijing Olympic Games on the chemical composition of aerosols, Atmos. Environ., 45, 2789–2794, https://doi.org/10.1016/j.atmosenv.2011.01.053, 2011.
Peng, L., Li, L., Zhang, G., Du, X., Wang, X., Peng, P., Sheng, G., and Bi, X.: Technical note: Measurement of chemically resolved volume equivalent diameter and effective density of particles by AAC-SPAMS, Atmos. Chem. Phys., 21, 5605–5613, https://doi.org/10.5194/acp-21-5605-2021, 2021.
Phares, D. J., Rhoads, K. P., Wexler, A. S., Kane, D. B., and Johnston, M. V.: Application of the ART-2a algorithm to laser ablation aerosol mass spectrometry of particle standards, Anal. Chem., 73, 2338–2344, https://doi.org/10.1021/ac0015063, 2001.
Pio, C., Cerqueira, M., Harrison, R. M., Nunes, T., Mirante, F., Alves, C., Oliveira, C., Sanchez de la Campa, A., Artíñano, B., and Matos, M.: OC/EC ratio observations in Europe: Re-thinking the approach for apportionment between primary and secondary organic carbon, Atmos. Environ., 45, 6121–6132, https://doi.org/10.1016/j.atmosenv.2011.08.045, 2011.
Pitz, M., Cyrys, J., and Karg, E.: Wiedensohler, A., Wichmann, H. E., and Heinrich, J.: Variability of apparent particle density of an urban aerosol, Environ. Sci. Technol., 37, 4336–4342, https://doi.org/10.1021/es034322p 2003.
Pokhrel, R. P., Wagner, N. L., Langridge, J. M., Lack, D. A., Jayarathne, T., Stone, E. A., Stockwell, C. E., Yokelson, R. J., and Murphy, S. M.: Parameterization of single-scattering albedo (SSA) and absorption Ångström exponent (AAE) with EC OC for aerosol emissions from biomass burning, Atmos. Chem. Phys., 16, 9549–9561, https://doi.org/10.5194/acp-16-9549-2016, 2016.
Prather, K. A.: Our current understanding of the impact of aerosols on climate change, ChemSusChem, 2, 377–379, https://doi.org/10.1002/cssc.200900037, 2009.
Pratt, K. A., Murphy, S. M., Subramanian, R., DeMott, P. J., Kok, G. L., Campos, T., Rogers, D. C., Prenni, A. J., Heymsfield, A. J., Seinfeld, J. H., and Prather, K. A.: Flight-based chemical characterization of biomass burning aerosols within two prescribed burn smoke plumes, Atmos. Chem. Phys., 11, 12549–12565, https://doi.org/10.5194/acp-11-12549-2011, 2011.
Qiu, C., Khalizov, A. F., Hogan, B., Petersen, E. L., and Zhang, R.: High sensitivity of diesel soot morphological and optical properties to combustion temperature in a shock tube, Environ. Sci. Technol., 48, 6444–6452, https://doi.org/10.1021/es405589d, 2014.
Rajesh, T. A. and Ramachandran, S.: Black carbon aerosol mass concentration, absorption and single scattering albedo from single and dual spot aethalometers: Radiative implications, J. Aerosol Sci., 119, 77–90, https://doi.org/10.1016/j.jaerosci.2018.02.001, 2018.
Rajesh, T. A. and Ramachandran, S.: Assessment of the coronavirus disease 2019 (COVID-19) pandemic imposed lockdown and unlock effects on black carbon aerosol, its source apportionment, and aerosol radiative forcing over an urban city in India, Atmos. Res., 267, 105924, https://doi.org/10.1016/j.atmosres.2021.105924, 2022.
Ramanathan, V., Crutzen, P. J., Kiehl, J. T., and Rosenfeld, D.: Aerosols, climate, and the hydrological cycle, Science, 294, 2119–2124, https://doi.org/10.1126/science.1064034, 2001.
Ren, H., Kang, M., Ren, L., Zhao, Y., Pan, X., Yue, S., Li, L., Zhao, W., Wei, L., Xie, Q., Li, J., Wang, Z., Sun, Y., Kawamura, K., and Fu, P.: The organic molecular composition, diurnal variation, and stable carbon isotope ratios of PM(2.5) in Beijing during the 2014 APEC summit, Environ. Pollut., 243, 919–928, https://doi.org/10.1016/j.envpol.2018.08.094, 2018.
Rissler, J., Nordin, E. Z., Eriksson, A. C., Nilsson, P. T., Frosch, M., Sporre, M. K., Wierzbicka, A., Svenningsson, B., Londahl, J., Messing, M. E., Sjogren, S., Hemmingsen, J. G., Loft, S., Pagels, J. H., and Swietlicki, E.: Effective density and mixing state of aerosol particles in a near-traffic urban environment, Environ. Sci. Technol., 48, 6300–6308, https://doi.org/10.1021/es5000353, 2014.
Rubasinghege, G., Lentz, R. W., Scherer, M. M., and Grassian, V. H.: Simulated atmospheric processing of iron oxyhydroxide minerals at low pH: roles of particle size and acid anion in iron dissolution, P. Natl. Acad. Sci. USA, 107, 6628–6633, https://doi.org/10.1073/pnas.0910809107, 2010.
See, S. W. and Balasubramanian, R.: Chemical characteristics of fine particles emitted from different gas cooking methods, Atmos. Environ., 42, 8852–8862, https://doi.org/10.1016/j.atmosenv.2008.09.011, 2008.
Sodeman, D. A., Toner, S. M., and Prather, K. A.: Determination of Single Particle Mass Spectral Signatures from Light-Duty Vehicle Emissions, Environ. Sci. Technol., 39, 4569–4580, https://doi.org/10.1021/es0489947 2005.
Song, X. H., Hopke, P. K., Fergenson, D. P., and Prather, K. A.: Classification of single particles analyzed by ATOFMS using an artificial neural network, ART-2A, Anal. Chem., 71, 860–865, https://doi.org/10.1021/ac9809682, 1999.
Spencer, M. T., Shields, L. G., and Prather, K. A.: Simultaneous Measurement of the Effective Density and Chemical Composition of Ambient Aerosol Particles, Environ. Sci. Technol., 41, 1303–1309, https://doi.org/10.1021/es061425+ 2007.
Su, B., Zhang, G., Zhuo, Z., Xie, Q., Du, X., Fu, Y., Wu, S., Huang, F., Bi, X., Li, X., Li, L., and Zhou, Z.: Different characteristics of individual particles from light-duty diesel vehicle at the launching and idling state by AAC-SPAMS, J. Hazard. Mater., 418, 126304, https://doi.org/10.1016/j.jhazmat.2021.126304, 2021.
Sun, J., Li, Y., Xu, W., Zhou, W., Du, A., Li, L., Du, X., Huang, F., Li, Z., Zhang, Z., Wang, Z., and Sun, Y.: Single-particle volatility and implications for brown carbon absorption in Beijing, China, Sci. Total Environ., 854, 158874, https://doi.org/10.1016/j.scitotenv.2022.158874, 2022a.
Sun, J., Sun, Y., Xie, C., Xu, W., Chen, C., Wang, Z., Li, L., Du, X., Huang, F., Li, Y., Li, Z., Pan, X., Ma, N., Xu, W., Fu, P., and Wang, Z.: The chemical composition and mixing state of BC-containing particles and the implications on light absorption enhancement, Atmos. Chem. Phys., 22, 7619–7630, https://doi.org/10.5194/acp-22-7619-2022, 2022b.
Sun, Y., Du, W., Fu, P., Wang, Q., Li, J., Ge, X., Zhang, Q., Zhu, C., Ren, L., Xu, W., Zhao, J., Han, T., Worsnop, D. R., and Wang, Z.: Primary and secondary aerosols in Beijing in winter: sources, variations and processes, Atmos. Chem. Phys., 16, 8309–8329, https://doi.org/10.5194/acp-16-8309-2016, 2016.
Sun, Y., Lei, L., Zhou, W., Chen, C., He, Y., Sun, J., Li, Z., Xu, W., Wang, Q., Ji, D., Fu, P., Wang, Z., and Worsnop, D. R.: A chemical cocktail during the COVID-19 outbreak in Beijing, China: Insights from six-year aerosol particle composition measurements during the Chinese New Year holiday, Sci. Total Environ., 742, 140739, https://doi.org/10.1016/j.scitotenv.2020.140739, 2020.
The People's Government of Beijing Municipality: Notice of the People's Government of Beijing Municipality on Temporary Traffic Management Measures during the Beijing 2022 Winter Olympic Games and Winter Paralympic Games, http://www.beijing.gov.cn/zhengce/zfwj/zfwj2016/szfwj/202201/t20220114_2590998.html (last access: 17 October 2023), 2022.
Wang, S., Zhao, M., Xing, J., Wu, Y., Zhou, Y., Lei, Y., He, K., Fu, L., and Hao, J.: Quantifying the Air Pollutants Emission Reduction during the 2008 Olympic Games in Beijing, Environ. Sci. Technol., 44, 2490–2496, https://doi.org/10.1021/es9028167, 2010.
Xie, C., He, Y., Lei, L., Zhou, W., Liu, J., Wang, Q., Xu, W., Qiu, Y., Zhao, J., Sun, J., Li, L., Li, M., Zhou, Z., Fu, P., Wang, Z., and Sun, Y.: Contrasting mixing state of black carbon-containing particles in summer and winter in Beijing, Environ. Pollut., 263, 114455, https://doi.org/10.1016/j.envpol.2020.114455, 2020.
Xu, W., Li, Z., Lambe, A. T., Li, J., Liu, T., Du, A., Zhang, Z., Zhou, W., and Sun, Y.: Secondary organic aerosol formation and aging from ambient air in an oxidation flow reactor during wintertime in Beijing, China, Environ. Res., 209, 112751, https://doi.org/10.1016/j.envres.2022.112751, 2022.
Xue, H., Khalizov, A. F., Wang, L., Zheng, J., and Zhang, R.: Effects of Coating of Dicarboxylic Acids on the Mass-Mobility Relationship of Soot Particles, Environ. Sci. Technol., 43, 2787–2792, https://doi.org/10.1021/es803287v 2009.
Yu, C., Liu, D., Hu, K., Tian, P., Wu, Y., Zhao, D., Wu, H., Hu, D., Guo, W., Li, Q., Huang, M., Ding, D., and Allan, J. D.: Aerodynamic size-resolved composition and cloud condensation nuclei properties of aerosols in a Beijing suburban region, Atmos. Chem. Phys., 22, 4375–4391, https://doi.org/10.5194/acp-22-4375-2022, 2022.
Zauscher, M. D., Wang, Y., Moore, M. J., Gaston, C. J., and Prather, K. A.: Air quality impact and physicochemical aging of biomass burning aerosols during the 2007 San Diego wildfires, Environ. Sci. Technol., 47, 7633–7643, https://doi.org/10.1021/es4004137, 2013.
Zhai, J., Lu, X., Li, L., Zhang, Q., Zhang, C., Chen, H., Yang, X., and Chen, J.: Size-resolved chemical composition, effective density, and optical properties of biomass burning particles, Atmos. Chem. Phys., 17, 7481–7493, https://doi.org/10.5194/acp-17-7481-2017, 2017.
Zhang, G., Bi, X., Lou, S., Li, L., Wang, H., Wang, X., Zhou, Z., Sheng, G., Fu, J., and Chen, C.: Source and mixing state of iron-containing particles in Shanghai by individual particle analysis, Chemosphere, 95, 9–16, https://doi.org/10.1016/j.chemosphere.2013.04.046, 2014.
Zhang, J., Huang, X., Wang, Y., Luo, B., Zhang, J., Song, H., Zhang, W., Liu, P., Schäfer, K., Wang, S., Luo, J., and Wu, P.: Characterization, mixing state, and evolution of single particles in a megacity of Sichuan Basin, southwest China, Atmos. Res., 209, 179–187, https://doi.org/10.1016/j.atmosres.2018.03.014, 2018.
Zhang, J., Li, H., Chen, L., Huang, X., Zhang, W., and Zhao, R.: Particle composition, sources and evolution during the COVID-19 lockdown period in Chengdu, southwest China: Insights from single particle aerosol mass spectrometer data, Atmos. Environ., 268, 118844, https://doi.org/10.1016/j.atmosenv.2021.118844, 2022a.
Zhang, J., Liu, Q., Chen, L., Li, H., Zhao, R., Huang, X., Zhang, W., and Wang, Y.: Interannual evolution of elemental carbon-containing particles in winter in the atmosphere of Chengdu, China, Sci. Total Environ., 804, 150133, https://doi.org/10.1016/j.scitotenv.2021.150133, 2022b.
Zhang, Y., Pei, C., Zhang, J., Cheng, C., Lian, X., Chen, M., Huang, B., Fu, Z., Zhou, Z., and Li, M.: Detection of polycyclic aromatic hydrocarbons using a high performance-single particle aerosol mass spectrometer, J. Environ. Sci., 124, 806–822, https://doi.org/10.1016/j.jes.2022.02.003, 2022c.
Zhao, W., Hopke, P. K., Gelfand, E. W., and Rabinovitch, N.: Use of an expanded receptor model for personal exposure analysis in schoolchildren with asthma, Atmos. Environ., 41, 4084–4096, https://doi.org/10.1016/j.atmosenv.2007.01.037, 2007.
Zhong, Q., Cheng, C., Li, M., Yang, S., Wang, Z., Yun, L., Liu, S., Mao, L., Fu, Z., and Zhou, Z.: Insights into the different mixing states and formation processes of amine-containing single particles in Guangzhou, China, Sci. Total. Environ., 846, 157440, https://doi.org/10.1016/j.scitotenv.2022.157440, 2022.
Zhou, R., Yan, C., Yang, Q., Niu, H., Liu, J., Xue, F., Chen, B., Zhou, T., Chen, H., Liu, J., and Jin, Y.: Characteristics of wintertime carbonaceous aerosols in two typical cities in Beijing-Tianjin-Hebei region, China: Insights from multiyear measurements, Environ. Res., 216, 114469, https://doi.org/10.1016/j.envres.2022.114469, 2023.
Zhou, Y., Wu, Y., Yang, L., Fu, L., He, K., Wang, S., Hao, J., Chen, J., and Li, C.: The impact of transportation control measures on emission reductions during the 2008 Olympic Games in Beijing, China, Atmos. Environ., 44, 285–293, https://doi.org/10.1016/j.atmosenv.2009.10.040, 2010.
Zhu, Y., Li, W., Wang, Y., Zhang, J., Liu, L., Xu, L., Xu, J., Shi, J., Shao, L., Fu, P., Zhang, D., and Shi, Z.: Sources and processes of iron aerosols in a megacity in Eastern China, Atmos. Chem. Phys., 22, 2191–2202, https://doi.org/10.5194/acp-22-2191-2022, 2022.