the Creative Commons Attribution 4.0 License.
the Creative Commons Attribution 4.0 License.
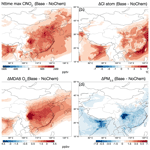
The impact of chlorine chemistry combined with heterogeneous N2O5 reactions on air quality in China
Xiajie Yang
Qiaoqiao Wang
Nan Ma
Weiwei Hu
Zhijiong Huang
Junyu Zheng
Ning Yang
Jiangchuan Tao
Juan Hong
Yafang Cheng
The heterogeneous reaction of N2O5 on Cl-containing aerosols (heterogeneous N2O5 + Cl chemistry) plays a key role in chlorine activation, NOx recycling, and consequently O3 and PM2.5 formation. In this study, we use the GEOS-Chem model with additional anthropogenic and biomass burning chlorine emissions combined with updated parameterizations for the heterogeneous N2O5 + Cl chemistry (i.e., the uptake coefficient of N2O5 () and the ClNO2 yield () to investigate the impacts of chlorine chemistry on air quality in China, the role of the heterogeneous N2O5 + Cl chemistry, and the sensitivity of air pollution formation to chlorine emissions and parameterizations for and . The model simulations are evaluated against multiple observational datasets across China and show significant improvement in reproducing observations of particulate chloride, N2O5, and ClNO2 when including anthropogenic chlorine emissions and updates to the parameterization of the heterogeneous N2O5 + Cl chemistry relative to the default model. The simulations show that total tropospheric chlorine chemistry could increase annual mean maximum daily 8 h average (MDA8) O3 by up to 4.5 ppbv but decrease PM2.5 by up to 7.9 µg m−3 in China, 83 % and 90 % of which could be attributed to the effect of the heterogeneous N2O5 + Cl chemistry. The heterogeneous uptake of N2O5 on chloride-containing aerosol surfaces is an important loss pathway of N2O5 as well as an important source of O3 and hence is particularly useful in elucidating the commonly seen ozone underestimations relative to observations. The importance of chlorine chemistry largely depends on both chlorine emissions and the parameterizations for the heterogeneous N2O5 + Cl chemistry. With the additional chlorine emissions, the simulations show that annual MDA8 O3 in China could be increased by up to 3.5 ppbv. The corresponding effect on PM2.5 concentrations varies largely with regions, with an increase of up to 4.5 µg m−3 in the North China Plain but a decrease of up to 3.7 µg m−3 in the Sichuan Basin. On the other hand, even with the same chlorine emissions, the effects on MDA8 O3 and PM2.5 in China could differ by 48 % and 27 %, respectively, between different parameterizations.
- Article
(13846 KB) - Full-text XML
-
Supplement
(2436 KB) - BibTeX
- EndNote
Chlorine (Cl) plays an important role in atmospheric chemistry in both the stratosphere and the troposphere, primarily via the reactions of the Cl atom with various atmospheric trace gases including dimethyl sulfide, methane, and other volatile organic compounds (VOCs). The chemistry of Cl is quite similar with that of hydroxyl radicals (OH) while Cl atoms react up to 2 orders of magnitude faster with some VOCs than OH (Atkinson et al., 2006). Studies have shown that Cl accounts for around 2.5 %–2.7 % of the global CH4 oxidation in the troposphere, and the contribution varies across regions, reaching up to 10 %–15 % in Cabo Verde and ∼ 20 % in east China (Lawler et al., 2011; Hossaini et al., 2016). The Cl atom, therefore, is regarded as a potentially important tropospheric oxidant.
In general, Cl atoms can be produced from the photo-dissociation and the oxidation of chlorinated organic species (e.g., CH3Cl, CH2Cl2, and CHCl3) and inorganic chlorine species (i.e., HCl and Cl2) (Saiz-Lopez and Von Glasow, 2012; Simpson et al., 2015). Nitryl chloride (ClNO2), formed through the heterogeneous reaction between dinitrogen pentoxide (N2O5) and chloride-containing aerosols (hereinafter referred to as the heterogeneous N2O5 + Cl chemistry), is found to be another important source of tropospheric Cl atoms in polluted regions (Liu et al., 2018; Haskins et al., 2019; Choi et al., 2020; Simpson et al., 2015). The heterogeneous formation of ClNO2 and the subsequent photolysis can be described by Reactions (R1)–(R4) shown below (Finlayson-Pitts et al., 1989; Osthoff et al., 2008). The net Reactions (R1) (N2O5 hydrolysis on non-chloride-containing aerosols) and (R2) (uptake of N2O5 on chloride-containing aerosols) could be expressed as Reaction (R3), in which the ClNO2 yield (i.e., , defined as the molar ratio of produced ClNO2 to total reacted N2O5) represents the fraction of N2O5 reacting via Reaction (R2).
Estimates based on model simulations have suggested that ClNO2 provides a source of Cl atoms totaling 0.66 Tg Cl a−1, with the vast majority (95 %) being in the Northern Hemisphere (Sherwen et al., 2016). The relative contribution of ClNO2 to global tropospheric Cl atoms is 14 % on average and exhibits clear regional variations (Sherwen et al., 2016). For example, the study by Riedel et al. (2012) reported that the relative contribution is approximately 45 % in Los Angeles based on a simple box model combined with local observations.
The heterogeneous formation of ClNO2 also serves as a reservoir for reactive nitrogen at night. The rapid photolysis of ClNO2 at daytime (Reaction R4) not only releases highly reactive Cl atoms but also recycles NO2 back to the atmosphere, which also significantly affects the daytime photochemistry (Riedel et al., 2014). Previous global and hemispheric models found that the heterogeneous N2O5 + Cl chemistry could increase monthly mean values of the maximum daily 8 h average (MDA8) O3 concentrations by 1.0–8.0 ppbv in most Northern Hemisphere regions (Sarwar et al., 2014; Wang et al., 2019). The reaction also impacts secondary aerosol formation, mainly through the recycling of NOx (Staudt et al., 2019; Mitroo et al., 2019). For example, Sarwar et al. (2014) estimated that ClNO2 production decreases nitrate by 3.3 % in winter and 0.3 % in summer averaged over the entire Northern Hemisphere. The influence of the heterogeneous formation of ClNO2 in China is even larger due to the polluted environment, leading to an increase in ozone concentrations by up to 7 ppbv and a decrease in total nitrate by up to 2.35 µg m−3 on a monthly mean basis (Li et al., 2016; Sarwar et al., 2014)
There are two key parameters that determine the uptake efficiency of N2O5 and production of ClNO2, the aerosol uptake coefficient of N2O5 () and the ClNO2 yield (). The most widely used parameterization for and was proposed by Bertram and Thornton (2009) (hereinafter referred to as BT09), which is based on the laboratory studies with considerations of aerosol water content, concentrations of nitrate and chloride, and specific surface area (i.e., the ratio of surface area concentrations to particle volume concentrations). However, recent field and model studies have shown that this parameterization would overestimate both and , especially in regions with high Cl levels (Mcduffie et al., 2018b, a; Xia et al., 2019; Chang et al., 2016; Hong et al., 2020; Yu et al., 2020). The discrepancies could be partly attributed to the complexity of atmospheric aerosols (e.g., mixing state and complex coating materials) in contrast to the simple proxies used in laboratory studies (Yu et al., 2020). Specifically, the suppressive effect of organic coatings is not considered in BT09. Several parameterizations updated from BT09 have been proposed by more recent studies based on field measurements and box model studies (Yu et al., 2020; Mcduffie et al., 2018a, b; Xia et al., 2019). However, some of these previous field-based parameterizations were derived from observations under different ambient conditions which may not be applicable to the highly polluted regions in China. A full evaluation of the representativeness of different parameterizations for the heterogeneous N2O5 + Cl chemistry and the associated impacts on ambient air quality in China is not available yet.
In addition to the parameterization, the influence of the heterogeneous N2O5 + Cl chemistry is also sensitive to chlorine emissions. In early modeling studies, global tropospheric chlorine is mainly from sea salt aerosols (SSA), and most of the chlorine over continental regions in North America and Europe is dominated by the long-range transport of SSA (Wang et al., 2019; Sherwen et al., 2017). The study by Wang et al. (2019) found an addition of anthropogenic chlorine emissions in the model would result in overestimates of HCl observations in the US and suggested insignificant influence of anthropogenic Cl in the US. However, there are also studies pointing out the importance of anthropogenic chlorine emissions in China (Le Breton et al., 2018; Yang et al., 2018; Hong et al., 2020). The study by X. Wang et al. (2020) suggested that anthropogenic chlorine emissions in China are more than 8 times higher than those in the US and could dominate reactive chlorine in China, resulting in an increase in PM2.5 and ozone by up to 3.2 µg m−3 and 1.9 ppbv on an annual mean basis, respectively. The comprehensive effects of anthropogenic chlorine on air quality as well as their sensitivities to different parameterizations for the heterogeneous N2O5 + Cl chemistry, however, has not been investigated in previous studies.
In this work, we use the GEOS-Chem model to investigate the impacts of chlorine chemistry including the heterogeneous N2O5 + Cl chemistry on air quality in China. Multiple observational datasets, including N2O5, ClNO2, O3, PM2.5, and its chemical compositions from different representative sites across China, are used to assess the model performance. With comprehensive chlorine emissions as well as appropriate parameterizations for the heterogeneous N2O5 + Cl chemistry, our objectives are (1) to improve the model's performance regarding the simulation of particulate chloride, ClNO2, N2O5, PM2.5, and O3 concentrations and (2) to extend the investigation on the effects of chlorine chemistry on both PM2.5 and ozone pollution in China as well as their sensitivities to anthropogenic chlorine emissions and the parameterizations for the heterogeneous N2O5 + Cl chemistry.
2.1 GEOS-Chem model
The GEOS-Chem model (version 12.9.3, http://www.geos-chem.org, last access: 14 March 2022, https://doi.org/10.5281/zenodo.3974569) is driven by assimilated meteorological fields (GEOS-FP) from the NASA Global Modeling and Assimilation Office (GMAO) at NASA Goddard Space Flight Center. The simulation in this study was conducted in a nested-grid model with a native horizontal resolution of 0.25∘ × 0.3125∘ (latitude × longitude) and 47 vertical levels over East Asia (15∘ S–55∘ N, 70–140∘ E). The dynamical boundary conditions were from a global simulation with a horizontal resolution of 2∘ × 2.5∘. We initialized the model with a 1 month spinup followed by a 1-year simulation for 2018. The simulation included a detailed representation of coupled NOx–ozone–VOC–aerosol–halogen chemistry (Sherwen et al., 2016; Wang et al., 2019). Previous studies have demonstrated the ability of GEOS-Chem to reasonably reproduce the magnitude and seasonal variation in surface ozone and particulate matter over east Asia and China (Wang et al., 2013; Geng et al., 2015; Li et al., 2019).
2.1.1 Chlorine chemistry
The GEOS-Chem model includes a comprehensive chlorine chemistry mechanism coupled with bromine and iodine chemistry. Full details could be found in the study of Wang et al. (2019). Briefly, the model includes 12 gas-phase inorganic chlorine species (Cl, Cl2, Cl2O2, ClNO2, ClNO3, ClO, ClOO, OClO, BrCl, ICl, HOCl, HCl), three gas-phase organic chlorine species (CH3Cl, CH2Cl2, CHCl3), and aerosol Cl− in two size bins (fine mode with radius ≤ 0.5 µm and coarse mode with radius > 0.5 µm). The gas–aerosol equilibrium between HCl and Cl− is calculated with ISORROPIA II (Fountoukis and Nenes, 2007) as part of the H2SO4–HCl–HNO3–NH3–non-volatile cation (NVC) thermodynamic system, where Na+ is used as a proxy for NVCs.
The heterogeneous uptake of N2O5 on aerosol surfaces leading to the production of ClNO2 and HNO3 has also been included in GEOS-Chem with the parameterizations for and proposed by McDuffie et al. (2018b, a) by default (hereinafter referred to as McDuffie parameterization). McDuffie parameterization is the first field-based empirical parameterization derived from the framework proposed in multiple laboratory studies including BT09 (Anttila et al., 2006; Bertram and Thornton, 2009; Riemer et al., 2009) to account for the uptake dependence on aerosol water and nitrate concentrations as well as the resistance from an organic coating. The coefficients for McDuffie parameterization were derived from applying a box model to observations of N2O5, ClNO2, O3, and NOx mixing ratios during the winter in the eastern US. The parameterization for accounts for both the inorganic and organic aerosol components and can be described by Eqs. (1)–(3):
where γcore represents the reactive uptake of the inorganic aerosol core and γcoat represents the retardation of the organic coating; c is the average gas-phase thermal velocity of N2O5 (m s−1), V is the total particle volume concentration (m3 m−3), Sa is the total particle surface area concentration (m2 m−3), KH is the unitless Henry law coefficient for N2O5 with a constant value of 51, [H2O] and are the concentrations of aerosol liquid water content and aerosol nitrate (mol L−1), respectively, and ka is the rate constant ratio representing the competition between aerosol-phase H2O and for the intermediate and is fixed at 0.04 in Eq. (2). R is the ideal gas constant; T is the temperature (K); Haq and Daq are the aqueous Henry law constant and aqueous-phase diffusion coefficient of N2O5, respectively; ε is a linear combination of relative humidity (RH) and O:C ratio (= 0.15 × O:C + 0.0016 × RH); and Rp, Rc, and l are the total particle radius, inorganic core radius, and organic coating thickness, respectively (m).
is calculated following BT09 but is reduced by 75 % based on the observations conducted in the eastern US and offshore in spring 2015 (i.e., the WINTER aircraft campaign) (McDuffie et al., 2018b). It could be described by Eq. (4):
where kc is the rate constant ratio representing the competition between aerosol-phase H2O and Cl− for the intermediate and is fixed at here, and [Cl−] is the concentration of aerosol chloride (mol L−1). For a more detailed description of the McDuffie parameterization, readers are referred to McDuffie et al. (2018b, a). Keep it in mind that the coefficients for the parameterizations in Eqs. (1)–(4) were derived to better reproduce wintertime observations in the eastern US. However, there are large uncertainties in both the values of the coefficients and functional form of the parameterizations, specifically related to their applicability to other regions.
Recently, Yu et al. (2020) proposed new parameterizations of and based on BT09 to account for the dependence on aerosol water, nitrate, and chloride concentrations but with coefficients derived from uptake coefficients directly measured on ambient aerosol in two rural sites in China. The parameterizations of and (hereinafter referred to as the Yu parameterization) are described by Eqs. (5) and (6), respectively.
Here kb is the rate constant ratio representing the competition between aerosol-phase Cl− and for the intermediate and is fixed at 3.4. In contrast to the McDuffie parameterization, ka and kc in the Yu parameterization are fixed at 0.033 and , respectively.
Although both parameterizations are developed based on BT09, there are significant differences of and between the McDuffie and Yu parameterizations. For , the McDuffie parameterization generally follows BT09 for the calculation of the uptake on inorganic aerosols (i.e., γcore) but excludes the dependence on aerosol chloride so as to better reproduce observed wintertime reactive nitrogen in the eastern US. Moreover, the parameterization accounts for the suppressive effects of the organics (i.e., γcoat), which are not directly included in BT09 (Anttila et al., 2006; Riemer et al., 2009; Morgan et al., 2015). In contrast to the McDuffie parameterization, the Yu parameterization excludes the organic suppression but includes the chloride enhancement so as to better reproduce observed in China (Yu et al., 2020). It is worth mentioning that the coefficients applied in the parameterization of also differ between the McDuffie and Yu parameterizations as both are fixed to reproduce the ambient observation representing different pollution conditions. For example, ka is equal to 0.04 in Eq. (2) but 0.033 in Eq. (5). The in the McDuffie parameterization is thus expected to be lower compared with the Yu parameterization due to the resistance from organic coating and the lack of the chloride enhancement. For , both the McDuffie and Yu parameterizations are based on BT09, but with different coefficients (i.e., kc = in Eq. 4 and in Eq. 6). Although kc in Eq. (4) is relatively smaller, the scaling factor of 0.25 applied in Eq. (4) ultimately results in a much smaller in the McDuffie parameterization compared with the Yu parameterization under the same condition. Again, keep it in mind that the McDuffie parameterization is derived from fits to observations over the eastern US (McDuffie et al., 2018a) while the Yu parameterization is fitted to observations at rural locations in China (Yu et al., 2020).
In this study, we updated the parameterizations for and in the heterogeneous N2O5 + Cl chemistry (hereinafter referred to as parameterizations for heterogeneous N2O5 + Cl chemistry) in the GEOS-Chem with the Yu parameterization. Additional simulation cases were also performed to evaluate the representativeness of both the Yu and McDuffie parameterizations regarding the simulation of N2O5, ClNO2, O3, PM2.5, and its chemical compositions in China. Detailed description of the model setup for related cases is provided below in Sect. 2.1.3.
2.1.2 Emissions
The study uses the Hemispheric Transport of Air Pollution (HTAPv2, http://www.htap.org/, last access: 14 March 2022) based on the emission of 2010 as a global anthropogenic inventory. This inventory is overwritten by a regional emission inventory MIX (with a horizontal resolution of 0.25∘ × 0.25∘) over East Asia based on the emission in 2017, which is developed for the Model Inter-Comparison Study for Asia (MICS-Asia) and covers all major anthropogenic sources in 30 Asian countries and regions (Li et al., 2017). In addition, anthropogenic emissions of black carbon (BC) and organic carbon (OC) in Guangdong Province, China (20–26∘ N, 109–117∘ E), are overwritten by a more recent high-resolution inventory (9 km × 9 km) described by Huang et al. (2021). Biomass burning emissions are from the Global Fire Emissions Database (GFED4) (Van et al., 2010) with a 3 h time resolution. The biogenic emissions of VOCs are calculated based on the Model of Emissions of Gases and Aerosols from Nature (MEGAN2.1) (Guenther et al., 2006).
Table 1 lists Cl emissions from all sources in the model. The global tropospheric chlorine by default in the model is mainly from the mobilization of Cl− from SSA distributed over two size bins (fine and coarse modes) (Wang et al., 2019), which is computed online as the integrals of the size-dependent source function depending on wind speeds and sea surface temperatures (Jaeglé et al., 2011). During the simulation year of 2018, SSA contributes 6.5 × 104 Gg Cl−, most of which however is distributed over the ocean due to its relatively short lifetime (∼ 1.5 d) (Choi et al., 2020). The release of atomic Cl from organic chlorine (CH3Cl, CH2Cl2, and CHCl3) via oxidation by OH and Cl is also included in the model by default. These organic chlorine gases are mainly of biogenic marine origin (Simmonds et al., 2006), with a mean tropospheric lifetime longer than 250 d (X. Wang et al., 2020), and are simulated in the model by imposing fixed surface concentrations as described by Schmidt et al. (2016). Total emissions of Cl atoms from CH3Cl, CH2Cl2, and CHCl3 are calculated to be 3.8, 2.4, and 0.70 Gg Cl a−1, respectively.
Table 1Chlorine emissions in China in the model.
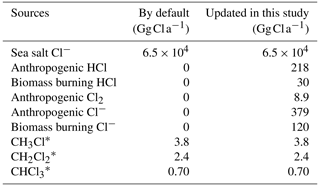
∗ Sources are shown in terms of the chemical release (e.g., +Cl, +OH, +hν).
Considering the importance of anthropogenic chlorine in China, we have further updated chlorine inventories in the model to account for anthropogenic HCl, Cl2 and fine particulate Cl−, and biomass burning HCl and Cl− emissions (also shown in Tables 1 and S1 in the Supplement). For fine particulate Cl− from both anthropogenic and biomass burning, the emissions are estimated based on PM2.5 emissions from MIX and GFED4 inventories combined with the emission ratios of fine particulate Cl− versus PM2.5 for different emission sectors adopted from the study of Fu et al. (2018). Estimated Cl− emissions from anthropogenic and biomass burning are 379 and 120 Gg, respectively, comparable to the results of 486 Gg in total for the year of 2014 by Fu et al. (2018). The anthropogenic emissions of HCl and Cl2 are from ACEIC (Anthropogenic Chlorine Emissions Inventory for China) (Liu et al., 2018) and are estimated to be 218 and 8.9 Gg Cl in China, respectively. For HCl from biomass burning, the emission factors from Lobert et al. (1999) are used for different types of biomass provided in GFED4, and a total emission of 30 Gg Cl is obtained in China in 2018. Total implemented chlorine emission for the simulation year of 2018 is 756 Gg Cl.
Figure 1 shows the distribution of Cl emissions from the sources mentioned above. Anthropogenic and biomass burning emissions of HCl are concentrated in the Northeast Plain, North China Plain, Yangtze River Delta, and Sichuan Basin and are up to 320 in the Sichuan Basin. Emissions of HCl are low in south China, mainly due to the low chlorine content of coal in these regions (Hong et al., 2020). The relative contribution of biomass burning to total HCl emissions in China is 14 % on average but could become dominant in the Northeast Plain due to the discrepancies in the spatial distributions of anthropogenic and biomass burning emissions. The anthropogenic Cl2 emissions have a similar spatial distribution to that of HCl but are 1 order of magnitude lower than HCl emissions. The distribution of non-sea-salt Cl− emissions is also similar to that of HCl and Cl2, except that non-sea-salt Cl− emissions are also high in central China. In contrast, emissions of sea salt Cl− (Fig. S1 in the Supplement) are mainly distributed over the ocean, implying limited influences inland due to rapid deposition during transport. The spatial distributions of different organic chlorine sources are similar, with maximums (∼ 0.5 ) in coastal regions (Fig. S1).
2.1.3 Model setup for different simulation cases
In this study, we conducted a series of simulation cases to investigate the effects of chlorine chemistry on air quality in China, the role of N2O5–ClNO2 chemistry, and the associated sensitivities to chlorine emissions as well as the parameterizations for N2O5–ClNO2 chemistry. A detailed model setup for those cases is listed in Table 2. The Base case is the one with all updates in this study, including additional chlorine sources from anthropogenic and biomass burning emissions as well as N2O5 uptake and ClNO2 production represented by the Yu parameterization. The NoEm case is conducted with a similar setup as the Base case but only includes chlorine emissions from SSA and organic chlorine sources so as to evaluate the model improvement originating from the updated chlorine emissions through the comparison with the Base case. The McDuffie case is also performed using the McDuffie instead of Yu parameterization for and while keeping others the same as the Base case so as to evaluate the discrepancies originating from different parameterizations for the heterogeneous N2O5 + Cl chemistry.
In addition, while keeping others the same as the Base case, the NoHet case sets to zero (Eq. 6) and removes the enhancement of N2O5 uptake from aerosol chloride (i.e., [Cl−] = 0 in Eq. 5). The comparison between the Base and NoHet cases could thus evaluate the importance of the heterogeneous N2O5 + Cl chemistry (i.e., the model sensitivities to a smaller gamma N2O5 and zero ClNO2 production). Similarly, combined with three more sensitivity cases (NoChem, NoEmHet, and NoAll; see details in Table 2), the study provides an overall evaluation of the importance of tropospheric chlorine chemistry as well as its sensitivities to chlorine emissions and the parameterizations for the heterogeneous N2O5 + Cl chemistry in the model.
2.2 Observations
Multiple observed datasets were applied in this study to evaluate the performance of GEOS-Chem simulation, including the concentrations of chemical compositions of PM2.5 from three representative sites, located in south (Guangzhou, 23.14∘ N, 113.36∘ E), east (Dongying, 37.82∘ N, 119.05∘ E), and north (Gucheng, 37.36∘ N, 115.96∘ E) China, respectively (Fig. S2). Concentrations of , , , Cl−, and organic matter (OM) in PM2.5 were measured by a high-resolution time-of-flight aerosol mass spectrometer (HR-ToF-AMS; Aerodyne Research Inc., USA; Decarlo et al., 2006) from 2 October to 18 November 2018 (with a time resolution of 1 min) at the Guangzhou site (W. Chen et al., 2021), and from 18 March to 21 April 2018 (with a 1 min time resolution) at the Dongying site. Concentrations of these species were measured by an Aerodyne quadruple aerosol chemical speciation monitor (ACSM; Aerodyne Research Inc., USA; Ng et al., 2011) from 11 November to 18 December 2018, with a time resolution of 2 min at the Gucheng site (Li et al., 2021).
Concentrations of N2O5 and ClNO2 (with a time resolution of 1 min) were also measured at the Guangzhou site by a chemical ionization mass spectrometer (CIMS, THS Instruments Inc., Atlanta; Kercher et al., 2009) from 25 September to 12 November 2018 (Ye et al., 2021). To have a thorough evaluation of the representativeness of different parameterizations for and , observations of ClNO2 and N2O5 at six more sites across China from previous studies (see Table S2 and Fig. S2) are also used in this study. It should be noted that model results sampled at those sites for comparison were simulated in the same months but different years while ignoring the uncertainties associated with the interannual variability.
In addition, we also use observed hourly data of O3 and PM2.5 published by the China National Environmental Monitoring Center (CNEMC, http://www.cnemc.cn/sssj/, last access on 20 June 2021) to evaluate the model's overall performance in China. The network was launched in 2013 as part of the Clean Air Action Plan and included ∼1500 stations located in 370 cities by 2018 (Fig. S2).
3.1 Improved model performance with updated chlorine emissions and parameterizations for the heterogeneous N2O5 + Cl chemistry
Figure 2 shows time series of observed and simulated Cl− concentrations at the Guangzhou, Dongying, and Gucheng sites. The observations show the lowest Cl− concentrations at the Guangzhou site (0.55 ± 0.52 µg m−3), although the site is the closest to the ocean among all three sites, while the highest concentrations (4.7 ± 3.3 µg m−3) are observed at the Gucheng site, away from the sea. Moderate concentrations of Cl− are observed at the Dongying site, around 1.1 ± 0.82 µg m−3. The relatively higher concentrations observed inland again suggest the dominance of non-sea-salt Cl− in China, as mentioned before in the Introduction.
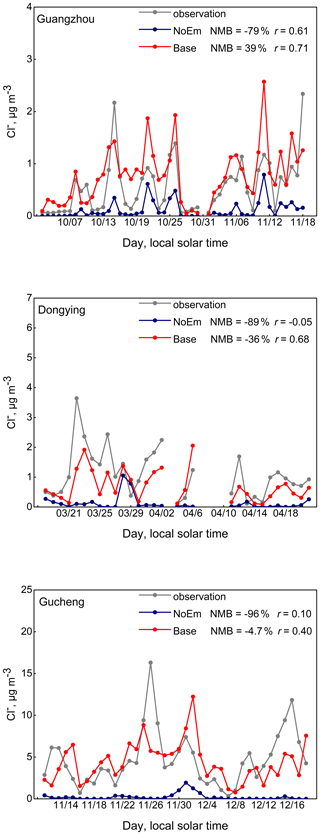
Figure 2Time series of simulated and observed particulate Cl− concentrations at the Guangzhou, Dongying, and Gucheng sites.
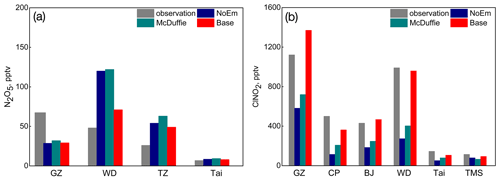
Figure 3Comparison of observed and simulated (a) averaged N2O5 concentrations and (b) mean nighttime maximum mixing ratio of ClNO2 concentrations at different sites. The simulation definitions are provided in Table 2. GZ: Guangzhou; WD: Wangdu; TZ: Taizhou; Tai: Mount Tai; CP: Changping; BJ: Beijing; TMS: Tai Mo Shan.
The comparison between observations and simulated results from the NoEm case shows a serious underestimate of Cl− concentrations, with normalized mean bias (NMB) ranging from −96 % to −79 %, suggesting the missing of significant chlorine sources in addition to sea salt chlorine. In contrast, the Base case with updated chlorine emissions exhibits much higher Cl− concentrations and can successfully reproduce observations, with average concentrations of 0.77 ± 0.54, 0.71 ± 0.52, and 4.5 ± 2.4 µg m−3 (NMB = 39 %, −36 %, and −4.7 %) at the Guangzhou, Dongying, and Gucheng sites, respectively. The increase in Cl− concentrations in the Base case compared with the NoEm case is the most significant at the Gucheng site, by a factor of 24 (from 0.19 to 4.5 µg m−3 on average). The slight underestimates at the Dongying site in the Base case could be to some extent explained by the bias in GFED4, which underestimates emissions from agricultural fires due to their small size and short duration as suggested by the study of Zhang et al. (2020). In spite of that, the model with the Base case reproduces the overall distribution of the observed particulate chloride concentrations in China well. The correlation coefficients (r) between observed and model results at the three sites also increase from −0.05–0.61 in the NoEm case to 0.40–0.71 in the Base case. The significant improvement in the model performance again suggests sources other than SSA play a key role in Cl− concentrations in China.
The comparison between observed and simulated N2O5 (Fig. 3a) shows that NMB for the NoEm case is −58 %, 150 %, 108 %, and 25 % at the Guangzhou, Wangdu, Taizhou, and Mount Tai sites, respectively. In contrast, the corresponding NMB for the Base case is much smaller, −57 %, 48 %, 91 %, and 18 %, respectively. The improvement in the Base case is apparent at most sites, implying that additional chlorine emissions could effectively increase the uptake coefficient of N2O5 in the Yu parameterization. As shown in Fig. S3, although the values of between the Base and NoEm cases are similar over the ocean, the Base case has relatively higher over China compared with the NoEm case (0.016 vs. 0.014 on an annual mean basis). Little improvement is found at the Guangzhou site (−58 % in the NoEm case vs. −57 % in the Base case). Previous studies also found an underestimation of N2O5 in the Pearl River Delta, which could be partly explained by the underestimation of the sources (e.g NO2) and/or the overestimation of the sink of N2O5 there (Dai et al., 2020; Li et al., 2016).
The N2O5 results from the McDuffie case, which uses the McDuffie parameterization (a default setting in GEOS-Chem; see Sects. 2.1.1 and 2.1.3) instead of the Yu parameterization are also shown in Fig. 3a. The NMB for the McDuffie case is −53 %, 154 %, 143 %, and 37 % at the Guangzhou, Wangdu, Taizhou, and Mount Tai sites, respectively. The comparison between the McDuffie and Base cases indicates that the Yu parameterization can reproduce observed N2O5 better in China in general, while the McDuffie parameterization tends to overestimate N2O5 concentrations. The overestimate of N2O5 in the McDuffie parameterization suggests the potential underestimate in the corresponding . As shown Fig. S3, the value of from the McDuffie case is much smaller than that from the Base case (0.0071 vs. 0.016 averaged over China).
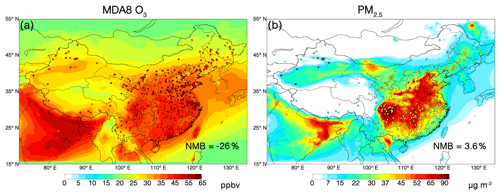
Figure 4Annual mean surface concentrations of (a) MDA8 O3 and (b) PM2.5 over China in 2018. GEOS-Chem model values from the Base case are shown as contours. Observations from the China National Environmental Monitoring Center (CNEMC) are shown as circles.
The underestimate in from the McDuffie case could to a large extent be explained by the suppressive effect of organic coatings (γcoat) as discussed above in Sect. 2.1.1. The magnitude of the organic suppression is highly dependent on many factors (e.g., organic composition, particle phase state) and thus remains poorly quantified (Griffiths et al., 2009; Gross et al., 2009; Thornton et al., 2003). Although many studies have shown that organic aerosol can suppress the N2O5 uptake (Anttila et al., 2006; Riemer et al., 2009), the level of organic suppression may be overpredicted in the currently implemented parameterization attributed to the poorly quantified and/or unknown factors (e.g., Morgan et al., 2015). For example, some studies found that ignoring the difference between water-soluble and water-insoluble organics may lead to an upper limit for the suppressive effect of organic coatings and consequently an underestimate in the solubility and diffusivity of N2O5 in organic matter (Chang et al., 2016; Yu et al., 2020). Although γcoat in the McDuffie parameterization is calculated as a function of organic aerosol O:C ratio and RH (see Eq. 2), which could increase with higher RH and higher O:C ratio, it may still overpredict the suppressive role of organic coatings in China. On the other hand, the study by Yu et al. (2020) found that excluding the organic coating best reproduced uptake coefficients observed in China. In addition, the underestimate in in the McDuffie parameterization in China could also be to some extent explained by the lack of the chloride enhancement (also discussed in Sect. 2.1.1). It is worth noting that the evaluation here is specific to China, and the differences between the Yu and McDuffie parameterizations have not been evaluated elsewhere.
For the comparison of ClNO2 (Fig. 3b), we use the mean nighttime (excluding the data at a local time of 10:00–16:00) maximum mixing ratio, as suggested by Wang et al. (2019). Observed ClNO2 is high in Guangzhou (1121 pptv) and Wangdu (∼ 990 pptv), followed by Changping (∼ 500 pptv) and Beijing (∼ 430 pptv). The lowest concentrations are obtained at Mount Tai and Tai Mo Shan (∼ 150 and 120 pptv, respectively) due to relatively clean conditions at high altitude. The comparison between observed and simulated ClNO2 at different sites also suggests a better model performance for the Base case, with NMB in the range of −28 %–22 %, compared with the NMB of −77 % to −31 % and −59 % to −36 % for the NoEm and McDuffie cases, respectively. The difference in ClNO2 concentrations is mainly associated with distinct values among different cases. As shown in Fig. S4, the value of is significantly higher in the Base case (0.36 averaged over China) than in the NoEm (0.14) and McDuffie (0.11) cases. The large difference between the NoEm and Base cases again emphasizes the important role of non-sea-salt chlorine in the formation of ClNO2. The overall underestimates in the McDuffie parameterization on the other hand may suggest that the scaling factor of 0.25 applied to in Eq. (4) is too much for the atmospheric condition in China. More field measurements and model evaluations are required to come up with a more precise parameterization better representing in China.
Overall, with updated chlorine emissions and Yu parameterization for and , the Base case agrees better with both the magnitude and the spatial variation in observed N2O5 and ClNO2 in China. The differences in and could also affect the ratios of ClNO2 to HNO3. As shown in Fig. S5, the value of is the highest from the Base case (9.8 % averaged in China and up to 47 % in the Sichuan Basin on an annual mean basis), followed by the McDuffie (4.7 % averaged in China and up to 18 % in the Sichuan Basin) and NoEm (3.1 % averaged in China and up to 12 % in coastal regions) cases.
To further elucidate how the model behaves in reproducing the spatial distribution of ozone and PM2.5 through the incorporation of the additional chlorine emissions and Yu parameterization for the heterogeneous N2O5 + Cl chemistry, simulated MDA8 O3 and PM2.5 from different cases were compared with observations across China. Figure 4 shows simulated annual mean MDA8 O3 and PM2.5 in 2018 in China from the Base case compared with the observations from CNEMC (China National Environmental Monitoring Center, introduced in Sect. 2.2). The observed annual mean MDA8 O3 and PM2.5 are 49 ppbv and 39 µg m−3, respectively, in 2018 in China. Model results from the Base case could generally reproduce observed spatial and seasonal variations in annual mean MDA8 O3 and PM2.5 concentrations, with NMB of −26 % and 3.6 % and r of 0.83 and 0.81, respectively (Fig. S6).
Table 3Normalized mean bias (NMB) and correlation coefficients (r) between observed and simulated MDA8 O3 and PM2.5 concentrations during 2018 in China.
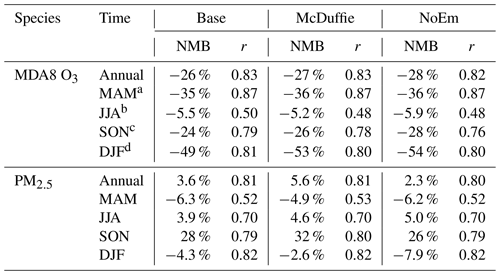
a March, April, and May (spring). b June, July, and August (summer). c September, October, and November (autumn). d December, January, and February (winter).
Table 3 also summarized the model performance on both annual and seasonal scales regarding the simulation of O3 and PM2.5 from different cases. For the comparison with observed MDA8 O3, although different simulation cases show a similar range of r, the Base case tends to have a slightly smaller bias in general, with NMB of −26 % on an annual average (−49 % to −5.5 % on seasonal mean) vs. −28 % (−54 % to −5.9 %) in the NoEm case and −27 % (−53 % to −5.2 %) in the McDuffie case. For the comparison with observed PM2.5, the NMB bias from the Base case is 3.6 % on an annual average (−6.3 %–28 % seasonal mean). Compared with the NoEm case, there is some improvement in summer (5.0 % vs. 3.9 %) and winter (−7.9 % vs. −4.3 %) but slightly larger bias in autumn (26 % vs. 28 %). The McDuffie case on the other hand produces slightly higher PM2.5 concentrations, with NMB of 5.6 %. Regarding the chemical compositions of PM2.5 (Table S3), although the model performance varies with sites and species, the Base case demonstrates better agreement with observations compared with the NoEm and McDuffie cases in general.
On the whole, the model performance is better with the additional anthropogenic and biomass burning chlorine emissions combined with the Yu parameterization for the heterogeneous N2O5 + Cl chemistry. Therefore, the following investigation of the impacts of chlorine chemistry on air quality in China as well as their sensitivities to chlorine emissions and parameterizations for the heterogeneous N2O5 + Cl chemistry is mainly based on the Base case.
3.2 Impacts of tropospheric chlorine chemistry on air quality and the role of the heterogeneous N2O5 + Cl chemistry
To comprehensively quantify the importance of chlorine chemistry, we conducted a sensitivity case in which all related tropospheric chlorine chemistry was turned off (NoChem, also listed in Table 2). The differences between the Base and NoChem cases (Figs. 5 and S7) could thus represent the impact of the chlorine chemistry. The comparison shows that chlorine chemistry could increase annual mean nighttime max ClNO2 surface concentrations by 243 pptv averaged in China (up to 1548 pptv in the Sichuan Basin). The increase in annual mean Cl atoms is 1.7 × 103 averaged in China (up to 7 × 103 in coastal regions). The increased Cl atoms could react with VOCs (especially alkanes), producing more peroxy radicals, including organic peroxy radicals (RO2) and hydroperoxyl radicals (HO2). As shown in Fig. S7 (a), the chlorine chemistry could increase annual mean HO2 concentrations by 1.6 × 106 averaged in China (up to 8.6 × 106 in the coastal regions). In the presence of NO, the peroxy radicals recycle OH while they oxidize NO to NO2. The subsequent photolysis of NO2 could further lead to more O3 production and consequently also more OH (Osthoff et al., 2008; Riedel et al., 2014; Simpson et al., 2015). On the other hand, the recycling of NOx back into the atmosphere associated with the photolysis of ClNO2 could also lead to more O3 production. The results here show a significant increase in surface annual mean OH (Fig. S7b) and MDA8 O3 (Fig. 5c) by 3.8 × 104 and 1.1 ppbv, respectively, averaged in China (up to 1.2 × 105 and 4.5 ppbv, respectively in the Sichuan Basin). In contrast, annual mean PM2.5 surface concentrations are decreased by 0.91 µg m−3 averaged in China (up to 7.9 µg m−3 in the Sichuan Basin), mainly due to the decrease in and (up to 6.4 and 1.9 µg m−3, respectively), although concentrations are increased slightly by up to 1.2 µg m−3 in the Sichuan Basin (Fig. S7).
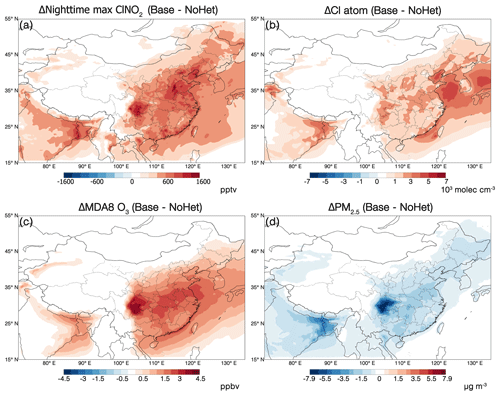
Figure 6Effects of the heterogeneous N2O5 + Cl chemistry on annual mean surface concentrations of (a) nighttime max ClNO2, (b) Cl atom, (c) MDA8 O3, and (d) PM2.5 in China, estimated as the differences between the Base and NoHet cases.
Both global and regional studies suggested that the heterogeneous N2O5 + Cl chemistry can enhance O3 production through the production of Cl atoms and the recycling of NOx (Li et al., 2016; Sarwar et al., 2014; Wang et al., 2019). Therefore, we further investigate the role that the heterogeneous N2O5 + Cl chemistry plays in tropospheric chlorine chemistry through the comparison between the Base and NoHet (Figs. 6 and S8) cases. Keep it in mind that the comparison mainly assesses the impact of ClNO2 production, namely the uptake of N2O5 on chloride aerosol, not the general role of N2O5 heterogeneous chemistry. The comparison illustrates that the heterogeneous N2O5 + Cl chemistry could result in a significant production of ClNO2, reaching 600–1400 pptv for annual mean nighttime max surface concentrations in the North China Plain and up to 1546 pptv in the Sichuan Basin. The change in the surface concentrations of Cl atoms (an annual mean increase of 1–4 × 103 in central and eastern China) is mainly due to the photolysis of ClNO2 and accounts for 74 % of total change in annual mean Cl atoms due to all tropospheric chlorine chemistry in China, which is consistent with the results from the previous study by Liu et al. (2017).
In addition to the production of Cl atoms, the ClNO2 formation also affects the partitioning of NOy from HNO3 into more reactive forms (e.g., NOx and ClNO2) through the recycling of NOx and is therefore of great importance in atmospheric chemistry (Bertram et al., 2013; Li et al., 2016; S. Wang et al., 2020). To analyze the impact of the heterogeneous N2O5 + Cl chemistry on NOy partitioning, Fig. S9 shows the change in the ratios of NOx to NOy and to NOy as the difference between the Base and NoHet cases. Since ClNO2 could be treated as a reservoir for reactive nitrogen at night, we include ClNO2 as part of NOx in the calculation (NOx = NO + NO2 + ClNO2 and NOy + NO + NO2 + ClNO2 + HNO3 + 2 × N2O5 + NO3 + HONO + HNO4 + + various organic nitrates). The results show that due to the ClNO2 production, the ratios of NOx to NOy increase by 1.8 % averaged in China and up to 5.4 % in the Sichuan Basin, Northeast Plain, and North China Plain on an annual mean basis. Meanwhile, the ratios of to NOy decrease by 1.1 % averaged in China and up to 5.1 % in the Sichuan Basin on an annual mean basis.
Consequently, the annual mean MDA8 O3 surface concentrations are increased by 1.5–3 ppbv in central and eastern China and up to 3.8 ppbv in the Sichuan Basin, accounting for 83 % of total change in annual mean MDA8 O3 due to all tropospheric chlorine chemistry in China. It is interesting to note that while MDA8 O3 surface concentrations show maxima in summer and minima in winter in general, the influence of the heterogeneous N2O5 + Cl chemistry on O3 concentrations exhibits a different seasonality. The increase in seasonal mean MDA8 O3 concentrations is the largest in winter (by up to 6.5 ppbv in the Sichuan Basin) but is less than 1.5 ppbv in summer. This is because of more accumulation of N2O5 and ClNO2 in dark conditions in long winter nights (Sarwar et al., 2014).
There is also an obvious decrease in the annual mean surface concentrations of PM2.5 attributed to the heterogeneous N2O5 + Cl chemistry, ranging from 1.5 to 4.5 µg m−3 in central and eastern China (accounting for 90 % of total change in annual mean PM2.5 due to all tropospheric chlorine chemistry in China). The decrease is more significant in autumn and winter in China, with a range of 3.5–5.5 µg m−3 in central and eastern China and up to 11 µg m−3 in the Sichuan Basin. In contrast, the decrease in PM2.5 is less than 2 µg m−3 in summer in China. The change in PM2.5 is mainly due to the decrease in (up to 6.2 µg m−3 in the Sichuan Basin on an annual average). In addition, is also decreased by up to 1.8 µg m−3 in the Sichuan Basin annually on average, following the pattern of . This is because NH3 is in excess in most regions in China (Xu et al., 2019), and the formation of ClNO2 via Reaction (R2) could hinder the formation of HNO3 and shift the partitioning between NH3 and towards NH3. Unlike the change in and , the heterogeneous N2O5 + Cl chemistry increases surface concentration slightly, which could be explained by the enhancements of atmospheric oxidation associated with the increase in Cl atoms, OH, and O3, facilitating the formation of secondary aerosols (Sarwar et al., 2014).
On the other hand, the effect of tropospheric chlorine chemistry without the heterogeneous N2O5 + Cl chemistry is much smaller (Fig. S10, the comparison between the NoHet and NoChem cases), leading to an increase of up to 0.7 ppbv in inland China and a decrease of 0.3–0.5 ppbv in coastal regions for annual mean MDA8 O3 concentrations. The increase is probably associated with Cl atoms from photolysis of gas-phase chlorine, especially non-sea-salt Cl2 in inland China, while the decrease at coastal regions is mainly due to catalytic production of bromine and iodine radicals originating from sea salt aerosols. The comparison demonstrates the dominance of the heterogeneous N2O5 + Cl chemistry in total tropospheric chlorine chemistry in China.
3.3 The effect of heterogeneous N2O5 + Cl chemistry in response to chlorine emissions
Since both and in the Yu parameterization are highly dependent on [Cl−], the effect of the heterogeneous N2O5 + Cl chemistry on air quality is thus sensitive to chlorine emissions. Figure 7 shows the effects of the additional chlorine emissions from anthropogenic and biomass burning sources on annual mean surface concentrations of different species (Cl−, Cl atoms, OH, MDA8 O3, PM2.5, and ) in China, calculated as the differences between the Base and the NoEm cases. With the implementation of the additional chlorine emissions, the particulate Cl− concentration increased significantly in inland China, with the largest increase in the Sichuan Basin (4.5 µg m−3) and little change in west China. The increase is in the range of 1.5–3.5 µg m−3 in the North China Plain and <0.5 µg m−3 in south China. The spatial distribution of ΔCl atoms is also consistent with that of the additional chlorine emissions and ΔCl−, showing the largest increment in the Sichuan Basin (about 4.5–5 × 103 ). There is also a moderate increase in Cl atoms in the Northeast Plain and North China Plain, with a range of 1.5–4 × 103 . Only a minor increase in Cl atoms is found in south China (< 1 × 103 ).
As discussed earlier in Sect. 3.2, increased Cl atoms could lead to more HO2 and OH via VOC oxidation. Combined with increased NOx associated with the release of NO2 upon the photolysis of ClNO2, further increases in both O3 and OH could also be expected. The increase in OH is around 2–9 × 104 in central and eastern China on an annual mean basis. The increase in MDA8 O3 surface concentrations ranges from 0.5 to 3 ppbv in central and eastern China and reaches up to 3.5 ppbv in the Sichuan Basin on an annual average. The impacts of chlorine sources on O3 formation also vary with seasons. Although O3 pollution is generally severe in summer, the change in MDA8 O3 due to the additional chlorine sources is relatively minor, with maxima of 0.7 ppbv in the Sichuan Basin and <0.5 ppbv in most other regions averaged in summer. In contrast, the increase is most obvious in winter, with a maximum of 5.2 ppbv in the Sichuan Basin seasonally on average.
The effects of the additional chlorine emissions on surface PM2.5 concentrations are complicated. The North China Plain shows the largest increase (3–4.5 µg m−3 annually on average), mainly due to the increase in Cl−, which could also promote the heterogeneous N2O5 + Cl chemistry and lead to more production (C. Chen et al., 2021). In contrast, the Sichuan Basin exhibits both an increase (by up to 4.2 µg m−3) and a decrease (by up to 3.7 µg m−3). The decrease in PM2.5 in the Sichuan Basin is mainly due to the large decrease in there. In the Sichuan Basin, nitrate formation is dominated by the heterogeneous hydrolysis of N2O5 (Tian et al., 2019), while the additional Cl− could hinder the path of N2O5 hydrolysis due to the competition with the path of ClNO2 formation. Consequently, the additional chlorine emissions result in a decrease in of up to 5.6 µg m−3 in the Sichuan Basin annually on average.
In addition, concentrations could also be affected through the Reaction (R5):
In the Northeast Plain and North China Plain where anthropogenic and biomass burning emissions of HCl are high, the annual mean surface concentrations are increased by 0.5–1.5 µg m−3 (Fig. S11a). concentrations are also affected by the gas–particle partitioning equilibrium and decrease as the pH value gets higher (or increase with H+ concentrations). Therefore, the competition between the heterogeneous N2O5 + Cl chemistry and N2O5 hydrolysis could also affect the formation of . In other words, increased Cl− concentrations could result in less H+ and thus less . Consequently, there is also some decrease in concentrations in the Sichuan Basin associated with the large decrease in concentrations. In contrast, little change is found for surface concentrations, less than 0.5 µg m−3 in most regions of China (Fig. S11b).
It is worth mentioning that the effects of the additional chlorine emissions work mainly through the heterogeneous N2O5 + Cl chemistry. Without this heterogeneous chemistry, the increase in chlorine emissions shows only a minor change in Cl atoms (<103 in China, estimated as the difference between the NoHet and NoEmHet cases in Fig. S12). The impact of chlorine emissions on O3 concentrations also weakens when the heterogeneous N2O5 + Cl chemistry is turned off, with an increase of 0.5–1 ppbv in MDA8 O3 annually on average (vs. 0.5–3 ppbv mentioned above).
On the other hand, the impacts of heterogeneous N2O5 + Cl chemistry on air quality in inland China would be seriously underestimated if the additional anthropogenic and biomass burning chlorine sources are ignored. If only sea salt chlorine emission is included in the simulation, the increase in ClNO2 surface concentrations resulting from heterogeneous N2O5 + Cl chemistry only occurs in coastal regions due to the heterogeneous uptake of N2O5 on sea salt chloride aerosols (by up to 260 pptv annually on average, indicated by the difference between the NoEm and NoEmHet cases, Fig. S13). Consequently, the increase in Cl atoms and MDA8 O3 surface concentrations is found mainly in coastal regions. For instance, annual mean MDA8 O3 concentrations are increased by up to 2 ppbv in coastal regions, but by less than 0.5 ppbv in inland China. In other words, the dominance of the heterogeneous N2O5 + Cl chemistry in the impact of chlorine chemistry on air quality in China is to a large extent driven by the additional chlorine emissions.
3.4 The effect of heterogeneous N2O5 + Cl chemistry in response to parameterizations for and
It should be noted that the impact of the heterogeneous N2O5 + Cl chemistry on air quality not only depends on the amount of chlorine emissions but is also sensitive to the parameterizations for and . As discussed earlier (Fig. 3a), there exists a large difference in simulated N2O5 between the Base and NoEm cases at the Wangdu site, implying the sensitivity of to chlorine emissions in the Yu parameterization and thus the importance of non-sea-salt chlorine emissions in China. This is consistent with the dependence on chloride in the Yu parameterization, which is included to better reproduce observations in China (Yu et al., 2020). The comparison between the Base and NoHet cases ( = 0.016 and 0.014, respectively) also suggests that the heterogeneous uptake of N2O5 on chloride-containing aerosol surfaces in the Yu parameterization is an important loss pathway of N2O5 and should not be ignored.
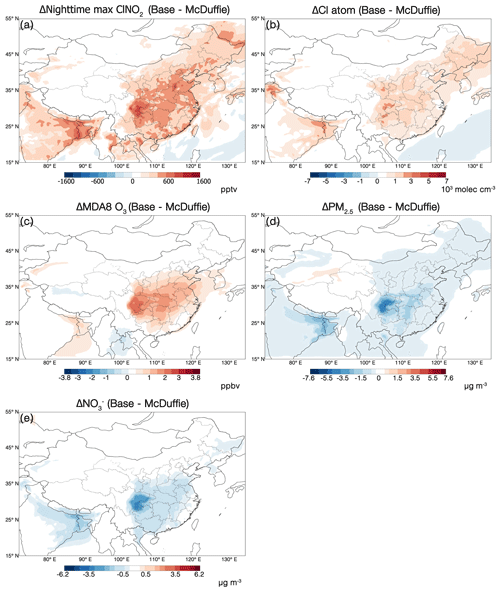
Figure 8Effects of different parameterizations on annual mean surface concentrations of (a) nighttime max ClNO2, (b) Cl atoms, (c) MDA8 O3, (d) PM2.5, and (e) in China, estimated as the differences between the Base and McDuffie cases.
Unlike the Yu parameterization, N2O5 concentrations have little dependence on chlorine emissions in the McDuffie parameterization (Fig. 3a). This insensitivity to chlorine emissions could be expected from Eq. (2), where the dependence on aerosol chloride is not included so as to better reproduce wintertime reactive nitrogen observations in the eastern US. The little dependence of on concentrations of Cl− together with the lower value of make the results from the McDuffie case less sensitive to chlorine emissions, producing fewer ClNO2 and Cl atoms compared with the Base case (with the Yu parameterization), although with the same emission. Consequently, the McDuffie case produces less O3, with annual mean surface concentrations of MDA8 O3 lower by 0.47 ppbv averaged in China (by up to 2 ppbv in the Sichuan Basin) but results in more PM2.5 (0.63 µg m−3 averaged in China and up to 4.7 µg m−3 in the Sichuan Basin on an annual mean basis mainly due to changes in ) (Fig. 8). In other words, compared to the Base case with the Yu parameterization, the impacts of chlorine emissions on annual MDA8 O3 and PM2.5 in the McDuffie case have been decreased by 48 % and 27 %, respectively, averaged in China. Therefore, even with the same amounts of chlorine emissions, the impacts of the heterogeneous N2O5 + Cl chemistry on air quality vary significantly with different parameterizations.
Considering the importance of chlorine chemistry in modulating the O3 and PM2.5 as well as the previously ignored chlorine emission from anthropogenic and biomass burning, we updated the GOES-Chem model in this study with comprehensive chlorine emissions and a new parameterization based on the study of Yu et al. (2020) for the heterogeneous N2O5 + Cl chemistry, followed by the extensive evaluation of model performance. Through the utilization of a large number of observational datasets, we found a substantial improvement has been achieved by the additional chlorine emissions, with NMB decreasing from −96 % to −79 % to −36 % to 39 % for Cl− simulation. The comparison with observed N2O5 and ClNO2 also indicates better model performance with the Yu parameterization while and are underestimated in the McDuffie parameterization (a default setting in GEOS-Chem), resulting in larger model bias. The simulation of O3 and PM2.5 also agrees better with observations in general in the Base case (with the additional chlorine emissions and the Yu parameterization) than the others.
Total tropospheric chlorine chemistry could increase Cl atoms by up to 7 × 103 and leads to an increase of up to 4.5 ppbv in MDA8 O3 but a decrease of up to 7.9 µg m−3 in PM2.5 concentrations on an annual mean basis in China. The decrease in PM2.5 is mainly associated with the decrease in and , by up to 6.4 and 1.9 µg m−3, respectively. The results also indicate that the heterogeneous N2O5 + Cl chemistry dominates the impact of chlorine chemistry, accounting for 83 % and 90 % of total change in O3 and PM2.5 concentrations. In other words, the chlorine chemistry without the heterogeneous N2O5 + Cl chemistry has a minor effect on annual mean MDA8 O3 (less than 0.7 ppbv) and PM2.5 (less than 1.5 µg m−3) concentrations in China. This mechanism is particularly useful in elucidating the commonly seen O3 underestimations relative to observations (e.g., Ma et al. 2019).
The effect of the heterogeneous N2O5 + Cl chemistry is sensitive to chlorine emissions. With the additional anthropogenic and biomass burning sources, simulated PM2.5 concentrations are increased by up to 4.5 µg m−3 in the North China Plain but decreased by up to 3.7 µg m−3 in the Sichuan Basin on an annual basis. The latter is mainly driven by the decrease in due to the competition between the formation of ClNO2 and HNO3 upon the uptake of N2O5 on aerosol surfaces. The additional emissions also increase Cl atoms and OH in China associated with the photolysis of ClNO2, consequently leading to an increase in annual mean MDA8 O3 concentrations by up to 3.5 ppbv. In contrast, the significance of the heterogeneous N2O5 + Cl chemistry, especially over inland China, would be severely underestimated if only sea salt chlorine is considered, with only a slight increase in MDA8 O3 (<0.5 ppbv) and a minor decrease in PM2.5 (< 1.5 µg m−3) in inland China.
Moreover, we found the importance of chlorine chemistry not only depends on the amount of emissions, but is also sensitive to the parameterizations for the heterogeneous N2O5 + Cl chemistry. Although they have the same emission, the effects on MDA8 O3 and PM2.5 in China from the McDuffie case are lower compared to the results with the Yu parameterization: differing by 48 % and 27 % in the annual average, respectively.
The data used in this study are available from Qiaoqiao Wang (qwang@jnu.edu.cn) upon request. The revised codes for different simulations could be downloaded via https://doi.org/10.5281/zenodo.5957287 (Yang et al., 2021).
The supplement related to this article is available online at: https://doi.org/10.5194/acp-22-3743-2022-supplement.
QW designed the study. XY performed the analysis. NM, WH, and BY provided the observation data. ZH and JZ provided the emission inventory. NM, WH, YG, BY, NY, JT, JH, YC, and HS discussed the results. XY and QW wrote the paper with input from all the other co-authors.
The contact author has declared that neither they nor their co-authors have any competing interests.
Publisher's note: Copernicus Publications remains neutral with regard to jurisdictional claims in published maps and institutional affiliations.
This research has been supported by the National Key Research and Development Program of China (grant no. 2018YFC0213901), the National Natural Science Foundation of China (grant nos. 41907182, 41877303, 91644218, 41877302, and 41875156), the Fundamental Research Funds for the Central Universities (grant no. 21621105), the Guangdong Innovative and Entrepreneurial Research Team Program (grant no. 2016ZT06N263), the Special Fund Project for Science and Technology Innovation Strategy of Guangdong Province (grant no. 2019B121205004), and the Guangdong Natural Science Funds for Distinguished Young Scholar (grant no. 2018B030306037).
This paper was edited by Steven Brown and reviewed by Erin E. McDuffie and one anonymous referee.
Anttila, T., Kiendler-Scharr, A., Tillmann, R., and Mentel, T. F.: On the Reactive Uptake of Gaseous Compounds by Organic-Coated Aqueous Aerosols: Theoretical Analysis and Application to the Heterogeneous Hydrolysis of N2O5, J. Phys. Chem. A, 110, 10435–10443, https://doi.org/10.1021/jp062403c, 2006..
Atkinson, R., Baulch, D. L., Cox, R. A., Crowley, J. N., Hampson, R. F., Hynes, R. G., Jenkin, M. E., Rossi, M. J., Troe, J., and IUPAC Subcommittee: Evaluated kinetic and photochemical data for atmospheric chemistry: Volume II – gas phase reactions of organic species, Atmos. Chem. Phys., 6, 3625–4055, https://doi.org/10.5194/acp-6-3625-2006, 2006.
Bertram, T. H. and Thornton, J. A.: Toward a general parameterization of N2O5 reactivity on aqueous particles: the competing effects of particle liquid water, nitrate and chloride, Atmos. Chem. Phys., 9, 8351–8363, https://doi.org/10.5194/acp-9-8351-2009, 2009.
Bertram, T. H., Perring, A. E., Wooldridge, P. J., Dibb, J., Avery, M. A., and Cohen, R. C.: On the export of reactive nitrogen from Asia: NOx partitioning and effects on ozone, Atmos. Chem. Phys., 13, 4617–4630, https://doi.org/10.5194/acp-13-4617-2013, 2013.
Chang, W. L., Brown, S. S., Stutz, J., Middlebrook, A. M., Bahreini, R., Wagner, N. L., Dubé, W. P., Pollack, I. B., Ryerson, T. B., and Riemer, N.: Evaluating N2O5 heterogeneous hydrolysis parameterizations for CalNex 2010, J. Geophys. Res.-Atmos., 121, 5051–5070, https://doi.org/10.1002/2015JD024737, 2016.
Chen, C., Zhang, H., Yan, W., Wu, N., Zhang, Q., and He, K.: Aerosol water content enhancement leads to changes in the major formation mechanisms of nitrate and secondary organic aerosols in winter over the North China Plain, Environ. Pollut., 287, 117625, https://doi.org/10.1016/j.envpol.2021.117625, 2021.
Chen, W., Ye, Y., Hu, W., Zhou, H., Pan, T., Wang, Y., Song, W., Song, Q., Ye, C., Wang, C., Wang, B., Huang, S., Yuan, B., Zhu, M., Lian, X., Zhang, G., Bi, X., Jiang, F., Liu, J., Canonaco, F., Prevot, A. S. H., Shao, M., and Wang, X.: Real-Time Characterization of Aerosol Compositions, Sources, and Aging Processes in Guangzhou During PRIDE-GBA 2018 Campaign, J. Geophys. Res.-Atmos., 126, e2021JD035114, https://doi.org/10.1029/2021JD035114, 2021.
Choi, M. S., Qiu, X., Zhang, J., Wang, S., Li, X., Sun, Y., Chen, J., and Ying, Q.: Study of Secondary Organic Aerosol Formation from Chlorine Radical-Initiated Oxidation of Volatile Organic Compounds in a Polluted Atmosphere Using a 3D Chemical Transport Model, Environ. Sci. Technol., 54, 13409–13418, https://doi.org/10.1021/acs.est.0c02958, 2020.
Dai, J., Liu, Y., Wang, P., Fu, X., Xia, M., and Wang, T.: The impact of sea-salt chloride on ozone through heterogeneous reaction with N2O5 in a coastal region of south China, Atmos. Environ., 236, 117604, https://doi.org/10.1016/j.atmosenv.2020.117604, 2020.
DeCarlo, P. F., Kimmel, J. R., Trimborn, A., Northway, M. J., Jayne, J. T., Aiken, A. C., Gonin, M., Fuhrer, K., Horvath, T., Docherty, K. S., Worsnop, D. R., and Jimenez, J. L.: Field-Deployable, High-Resolution, Time-of-Flight Aerosol Mass Spectrometer, Anal. Chem., 78, 8281–8289, https://doi.org/10.1021/ac061249n, 2006.
Finlayson-Pitts, B. J., Ezell, M. J., and Pitts, J. N. J.: Formation of chemically active chlorine compounds by reactions of atmospheric NaCl particles with gaseous N2O5 and ClONO2, Cheminform, 20, 241–244, 1989.
Fountoukis, C. and Nenes, A.: ISORROPIA II: a computationally efficient thermodynamic equilibrium model for K+–Ca2+–Mg2+––Na+–––Cl−–H2O aerosols, Atmos. Chem. Phys., 7, 4639–4659, https://doi.org/10.5194/acp-7-4639-2007, 2007.
Fu, X., Wang, T., Wang, S., Zhang, L., Cai, S., Xing, J., and Hao, J.: Anthropogenic Emissions of Hydrogen Chloride and Fine Particulate Chloride in China, Environ. Sci. Technol., 52, 1644–1654, https://doi.org/10.1021/acs.est.7b05030, 2018.
Geng, G., Zhang, Q., Martin, R. V., Van Donkelaar, A., Huo, H., Che, H., Lin, J., and He, K.: Estimating long-term PM2.5 concentrations in China using satellite-based aerosol optical depth and a chemical transport model, Remote Sens. Environ., 166, 262–270, 2015.
Griffiths, P. T., Badger, C. L., Cox, R. A., Folkers, M., Henk, H. H., and Mentel, T. F.: Reactive Uptake of N2O5 by Aerosols Containing Dicarboxylic Acids. Effect of Particle Phase, Composition, and Nitrate Content, J. Phys. Chem. A, 113, 5082–5090, https://doi.org/10.1021/jp8096814, 2009.
Gross, S., Iannone, R., Xiao, S., and Bertram, A. K.: Reactive uptake studies of NO3 and N2O5 on alkenoic acid, alkanoate, and polyalcohol substrates to probe nighttime aerosol chemistry, Phys. Chem. Chem. Phys., 11, 7792–7803, https://doi.org/10.1039/B904741G, 2009.
Guenther, A., Karl, T., Harley, P., Wiedinmyer, C., Palmer, P. I., and Geron, C.: Estimates of global terrestrial isoprene emissions using MEGAN (Model of Emissions of Gases and Aerosols from Nature), Atmos. Chem. Phys., 6, 3181–3210, https://doi.org/10.5194/acp-6-3181-2006, 2006.
Haskins, J. D., Lee, B. H., Lopez-Hilifiker, F. D., Peng, Q., Jaeglé, L., Reeves, J. M., Schroder, J. C., Campuzano-Jost, P., Fibiger, D., McDuffie, E. E., Jiménez, J. L., Brown, S. S., and Thornton, J. A.: Observational Constraints on the Formation of Cl2 From the Reactive Uptake of ClNO2 on Aerosols in the Polluted Marine Boundary Layer, J. Geophys. Res.-Atmos., 124, 8851–8869, https://doi.org/10.1029/2019JD030627, 2019.
Hong, Y., Liu, Y., Chen, X., Fan, Q., Chen, C., Chen, X., and Wang, M.: The role of anthropogenic chlorine emission in surface ozone formation during different seasons over eastern China, Sci. Total Environ., 723, 137697, https://doi.org/10.1016/j.scitotenv.2020.137697, 2020.
Hossaini, R., Chipperfield, M. P., Saiz-Lopez, A., Fernandez, R., Monks, S., Feng, W., Brauer, P., and von Glasow, R.: A global model of tropospheric chlorine chemistry: Organic versus inorganic sources and impact on methane oxidation, J. Geophys. Res.-Atmos., 121, 14,271-214,297, https://doi.org/10.1002/2016JD025756, 2016.
Huang, Z., Zhong, Z., Sha, Q., Xu, Y., Zhang, Z., Wu, L., Wang, Y., Zhang, L., Cui, X., Tang, M., Shi, B., Zheng, C., Li, Z., Hu, M., Bi, L., Zheng, J., and Yan, M.: An updated model-ready emission inventory for Guangdong Province by incorporating big data and mapping onto multiple chemical mechanisms, Sci. Total Environ., 769, 144535, https://doi.org/10.1016/j.scitotenv.2020.144535, 2021.
Jaeglé, L., Quinn, P. K., Bates, T. S., Alexander, B., and Lin, J.-T.: Global distribution of sea salt aerosols: new constraints from in situ and remote sensing observations, Atmos. Chem. Phys., 11, 3137–3157, https://doi.org/10.5194/acp-11-3137-2011, 2011.
Kercher, J. P., Riedel, T. P., and Thornton, J. A.: Chlorine activation by N2O5: simultaneous, in situ detection of ClNO2 and N2O5 by chemical ionization mass spectrometry, Atmos. Meas. Tech., 2, 193–204, https://doi.org/10.5194/amt-2-193-2009, 2009.
Lawler, M. J., Sander, R., Carpenter, L. J., Lee, J. D., von Glasow, R., Sommariva, R., and Saltzman, E. S.: HOCl and Cl2 observations in marine air, Atmos. Chem. Phys., 11, 7617–7628, https://doi.org/10.5194/acp-11-7617-2011, 2011.
Le Breton, M., Hallquist, Å. M., Pathak, R. K., Simpson, D., Wang, Y., Johansson, J., Zheng, J., Yang, Y., Shang, D., Wang, H., Liu, Q., Chan, C., Wang, T., Bannan, T. J., Priestley, M., Percival, C. J., Shallcross, D. E., Lu, K., Guo, S., Hu, M., and Hallquist, M.: Chlorine oxidation of VOCs at a semi-rural site in Beijing: significant chlorine liberation from ClNO2 and subsequent gas- and particle-phase Cl–VOC production, Atmos. Chem. Phys., 18, 13013–13030, https://doi.org/10.5194/acp-18-13013-2018, 2018.
Li, G., Su, H., Ma, N., Tao, J., Kuang, Y., Wang, Q., Hong, J., Zhang, Y., Kuhn, U., Zhang, S., Pan, X., Lu, N., Tang, M., Zheng, G., Wang, Z., Gao, Y., Cheng, P., Xu, W., Zhou, G., Zhao, C., Yuan, B., Shao, M., Ding, A., Zhang, Q., Fu, P., Sun, Y., Pöschl, U., and Cheng, Y.: Multiphase chemistry experiment in Fogs and Aerosols in the North China Plain (McFAN): integrated analysis and intensive winter campaign 2018, Faraday Discuss., 226, 207–222, https://doi.org/10.1039/D0FD00099J, 2021.
Li, K., Jacob, D. J., Liao, H., Shen, L., Zhang, Q., and Bates, K. H.: Anthropogenic drivers of 2013–2017 trends in summer surface ozone in China, P. Natl. Acad. Sci. USA, 116, 422–427, https://doi.org/10.1073/pnas.1812168116, 2019.
Li, M., Zhang, Q., Kurokawa, J.-I., Woo, J.-H., He, K., Lu, Z., Ohara, T., Song, Y., Streets, D. G., Carmichael, G. R., Cheng, Y., Hong, C., Huo, H., Jiang, X., Kang, S., Liu, F., Su, H., and Zheng, B.: MIX: a mosaic Asian anthropogenic emission inventory under the international collaboration framework of the MICS-Asia and HTAP, Atmos. Chem. Phys., 17, 935–963, https://doi.org/10.5194/acp-17-935-2017, 2017.
Li, Q., Zhang, L., Wang, T., Tham, Y. J., Ahmadov, R., Xue, L., Zhang, Q., and Zheng, J.: Impacts of heterogeneous uptake of dinitrogen pentoxide and chlorine activation on ozone and reactive nitrogen partitioning: improvement and application of the WRF-Chem model in southern China, Atmos. Chem. Phys., 16, 14875–14890, https://doi.org/10.5194/acp-16-14875-2016, 2016.
Liu, X., Qu, H., Huey, L. G., Wang, Y., Sjostedt, S., Zeng, L., Lu, K., Wu, Y., Hu, M., Shao, M., Zhu, T., and Zhang, Y.: High Levels of Daytime Molecular Chlorine and Nitryl Chloride at a Rural Site on the North China Plain, Environ. Sci. Technol., 51, 9588–9595, https://doi.org/10.1021/acs.est.7b03039, 2017.
Liu, Y., Fan, Q., Chen, X., Zhao, J., Ling, Z., Hong, Y., Li, W., Chen, X., Wang, M., and Wei, X.: Modeling the impact of chlorine emissions from coal combustion and prescribed waste incineration on tropospheric ozone formation in China, Atmos. Chem. Phys., 18, 2709–2724, https://doi.org/10.5194/acp-18-2709-2018, 2018.
Lobert, J. M., Keene, W. C., Logan, J. A., and Yevich, R.: Global chlorine emissions from biomass burning: Reactive Chlorine Emissions Inventory, J. Geophys. Res.-Atmos., 104, 8373–8389, https://doi.org/10.1029/1998JD100077, 1999.
Ma, M., Gao, Y., Wang, Y., Zhang, S., Leung, L. R., Liu, C., Wang, S., Zhao, B., Chang, X., Su, H., Zhang, T., Sheng, L., Yao, X., and Gao, H.: Substantial ozone enhancement over the North China Plain from increased biogenic emissions due to heat waves and land cover in summer 2017, Atmos. Chem. Phys., 19, 12195–12207, https://doi.org/10.5194/acp-19-12195-2019, 2019.
McDuffie, E. E., Fibiger, D. L., Dubé, W. P., Lopez Hilfiker, F., Lee, B. H., Jaeglé, L., Guo, H., Weber, R. J., Reeves, J. M., Weinheimer, A. J., Schroder, J. C., Campuzano-Jost, P., Jimenez, J. L., Dibb, J. E., Veres, P., Ebben, C., Sparks, T. L., Wooldridge, P. J., Cohen, R. C., Campos, T., Hall, S. R., Ullmann, K., Roberts, J. M., Thornton, J. A., and Brown, S. S.: ClNO2 Yields From Aircraft Measurements During the 2015 WINTER Campaign and Critical Evaluation of the Current Parameterization, J. Geophys. Res.-Atmos., 123, 12,994-913,015, https://doi.org/10.1029/2018JD029358, 2018a.
McDuffie, E. E., Fibiger, D. L., Dubé, W. P., Lopez-Hilfiker, F., Lee, B. H., Thornton, J. A., Shah, V., Jaeglé, L., Guo, H., Weber, R. J., Michael Reeves, J., Weinheimer, A. J., Schroder, J. C., Campuzano-Jost, P., Jimenez, J. L., Dibb, J. E., Veres, P., Ebben, C., Sparks, T. L., Wooldridge, P. J., Cohen, R. C., Hornbrook, R. S., Apel, E. C., Campos, T., Hall, S. R., Ullmann, K., and Brown, S. S.: Heterogeneous N2O5 Uptake During Winter: Aircraft Measurements During the 2015 WINTER Campaign and Critical Evaluation of Current Parameterizations, J. Geophys. Res.-Atmos., 123, 4345–4372, https://doi.org/10.1002/2018JD028336, 2018b.
Mitroo, D., Gill, T. E., Haas, S., Pratt, K. A., and Gaston, C. J.: ClNO2 Production from N2O5 Uptake on Saline Playa Dusts: New Insights into Potential Inland Sources of ClNO2, Environ. Sci. Technol., 53, 7442–7452, 2019.
Morgan, W. T., Ouyang, B., Allan, J. D., Aruffo, E., Di Carlo, P., Kennedy, O. J., Lowe, D., Flynn, M. J., Rosenberg, P. D., Williams, P. I., Jones, R., McFiggans, G. B., and Coe, H.: Influence of aerosol chemical composition on N2O5 uptake: airborne regional measurements in northwestern Europe, Atmos. Chem. Phys., 15, 973–990, https://doi.org/10.5194/acp-15-973-2015, 2015.
Ng, N. L., Herndon, S. C., Trimborn, A., Canagaratna, M. R., Croteau, P. L., Onasch, T. B., Sueper, D., Worsnop, D. R., Zhang, Q., Sun, Y. L., and Jayne, J. T.: An Aerosol Chemical Speciation Monitor (ACSM) for Routine Monitoring of the Composition and Mass Concentrations of Ambient Aerosol, Aerosol Sci. Technol., 45, 780–794, https://doi.org/10.1080/02786826.2011.560211, 2011.
Osthoff, H. D., Roberts, J. M., Ravishankara, A. R., Williams, E. J., Lerner, B. M., Sommariva, R., Bates, T. S., Coffman, D., Quinn, P. K., Dibb, J. E., Stark, H., Burkholder, J. B., Talukdar, R. K., Meagher, J., Fehsenfeld, F. C., and Brown, S. S.: High levels of nitryl chloride in the polluted subtropical marine boundary layer, Nat. Geosci., 1, 324–328, https://doi.org/10.1038/ngeo177, 2008.
Riedel, T. P., Bertram, T. H., Crisp, T. A., Williams, E. J., Lerner, B. M., Vlasenko, A., Li, S. M., Gilman, J., De Gouw, J., and Bon, D. M.: Nitryl Chloride and Molecular Chlorine in the Coastal Marine Boundary Layer, Environ. Sci. Technol., 46, 10463–10470, 2012.
Riedel, T. P., Wolfe, G. M., Danas, K. T., Gilman, J. B., Kuster, W. C., Bon, D. M., Vlasenko, A., Li, S. M., Williams, E. J., Lerner, B. M., Veres, P. R., Roberts, J. M., Holloway, J. S., Lefer, B., Brown, S. S., and Thornton, J. A.: An MCM modeling study of nitryl chloride (ClNO2) impacts on oxidation, ozone production and nitrogen oxide partitioning in polluted continental outflow, Atmos. Chem. Phys., 14, 3789–3800, https://doi.org/10.5194/acp-14-3789-2014, 2014.
Riemer, N., Vogel, H., Vogel, B., Anttila, T., Kiendler-Scharr, A., and Mentel, T. F.: Relative importance of organic coatings for the heterogeneous hydrolysis of N2O5 during summer in Europe, J. Geophys. Res.-Atmos., 114, D17307, https://doi.org/10.1029/2008JD011369, 2009.
Saiz-Lopez, A. and von Glasow, R.: Reactive halogen chemistry in the troposphere, Chem. Soc. Rev., 41, 6448–6472, https://doi.org/10.1039/C2CS35208G, 2012.
Sarwar, G., Simon, H., Xing, J., and Mathur, R.: Importance of tropospheric ClNO2 chemistry across the Northern Hemisphere, Geophys. Res. Lett., 41, 4050–4058, https://doi.org/10.1002/2014GL059962, 2014.
Schmidt, J. A., Jacob, D. J., Horowitz, H. M., Hu, L., Sherwen, T., Evans, M. J., Liang, Q., Suleiman, R. M., Oram, D. E., Le Breton, M., Percival, C. J., Wang, S., Dix, B., and Volkamer, R.: Modeling the observed tropospheric BrO background: Importance of multiphase chemistry and implications for ozone, OH, and mercury, J. Geophys. Res.-Atmos., 121, 11,819-811,835, https://doi.org/10.1002/2015JD024229, 2016.
Sherwen, T., Schmidt, J. A., Evans, M. J., Carpenter, L. J., Großmann, K., Eastham, S. D., Jacob, D. J., Dix, B., Koenig, T. K., Sinreich, R., Ortega, I., Volkamer, R., Saiz-Lopez, A., Prados-Roman, C., Mahajan, A. S., and Ordóñez, C.: Global impacts of tropospheric halogens (Cl, Br, I) on oxidants and composition in GEOS-Chem, Atmos. Chem. Phys., 16, 12239–12271, https://doi.org/10.5194/acp-16-12239-2016, 2016.
Sherwen, T., Evans, M. J., Sommariva, R., Hollis, L. D. J., Ball, S. M., Monks, P. S., Reed, C., Carpenter, L. J., Lee, J. D., Forster, G., Bandy, B., Reeves, C. E., and Bloss, W. J.: Effects of halogens on European air-quality, Faraday Discuss., 200, 75–100, https://doi.org/10.1039/C7FD00026J, 2017.
Simmonds, P. G., Manning, A. J., Cunnold, D. M., McCulloch, A., O'Doherty, S., Derwent, R. G., Krummel, P. B., Fraser, P. J., Dunse, B., Porter, L. W., Wang, R. H. J., Greally, B. R., Miller, B. R., Salameh, P., Weiss, R. F., and Prinn, R. G.: Global trends, seasonal cycles, and European emissions of dichloromethane, trichloroethene, and tetrachloroethene from the AGAGE observations at Mace Head, Ireland, and Cape Grim, Tasmania, J. Geophys. Res.-Atmos., 111, D18304, https://doi.org/10.1029/2006JD007082, 2006.
Simpson, W. R., Brown, S. S., Saiz-Lopez, A., Thornton, J. A., and von Glasow, R.: Tropospheric Halogen Chemistry: Sources, Cycling, and Impacts, Chem. Rev., 115, 4035–4062, https://doi.org/10.1021/cr5006638, 2015.
Staudt, S., Gord, J. R., Karimova, N. V., McDuffie, E. E., Brown, S. S., Gerber, R. B., Nathanson, G. M., and Bertram, T. H.: Sulfate and Carboxylate Suppress the Formation of ClNO2 at Atmospheric Interfaces, ACS Earth and Space Chemistry, 3, 1987–1997, https://doi.org/10.1021/acsearthspacechem.9b00177, 2019.
Thornton, J. A., Braban, C. F., and Abbatt, J. P. D.: N2O5 hydrolysis on sub-micron organic aerosols: the effect of relative humidity, particle phase, and particle size, Phys. Chem. Chem. Phys., 5, 4593–4603, https://doi.org/10.1039/B307498F, 2003.
Tian, M., Liu, Y., Yang, F., Zhang, L., Peng, C., Chen, Y., Shi, G., Wang, H., Luo, B., Jiang, C., Li, B., Takeda, N., and Koizumi, K.: Increasing importance of nitrate formation for heavy aerosol pollution in two megacities in Sichuan Basin, southwest China, Environ. Pollut., 250, 898–905, https://doi.org/10.1016/j.envpol.2019.04.098, 2019.
van der Werf, G. R., Randerson, J. T., Giglio, L., Collatz, G. J., Mu, M., Kasibhatla, P. S., Morton, D. C., DeFries, R. S., Jin, Y., and van Leeuwen, T. T.: Global fire emissions and the contribution of deforestation, savanna, forest, agricultural, and peat fires (1997–2009), Atmos. Chem. Phys., 10, 11707–11735, https://doi.org/10.5194/acp-10-11707-2010, 2010.
Wang, S., Su, H., Chen, C., Tao, W., Streets, D. G., Lu, Z., Zheng, B., Carmichael, G. R., Lelieveld, J., Pöschl, U., and Cheng, Y.: Natural gas shortages during the “coal-to-gas” transition in China have caused a large redistribution of air pollution in winter 2017, P. Natl. Acad. Sci. USA, 117, 31018–31025, https://doi.org/10.1073/pnas.2007513117, 2020.
Wang, X., Jacob, D. J., Eastham, S. D., Sulprizio, M. P., Zhu, L., Chen, Q., Alexander, B., Sherwen, T., Evans, M. J., Lee, B. H., Haskins, J. D., Lopez-Hilfiker, F. D., Thornton, J. A., Huey, G. L., and Liao, H.: The role of chlorine in global tropospheric chemistry, Atmos. Chem. Phys., 19, 3981–4003, https://doi.org/10.5194/acp-19-3981-2019, 2019.
Wang, X., Jacob, D. J., Fu, X., Wang, T., and Liao, H.: Effects of Anthropogenic Chlorine on PM2.5 and Ozone Air Quality in China, Environ. Sci. Technol., 54, 9908–9916, 2020.
Wang, Y., Shen, L., Wu, S., Mickley, L., He, J., and Hao, J.: Sensitivity of surface ozone over China to 2000–2050 global changes of climate and emissions, Atmos. Environ., 75, 374–382, 2013.
Xia, M., Wang, W., Wang, Z., Gao, J., Li, H., Liang, Y., Yu, C., Zhang, Y., Wang, P., Zhang, Y., Bi, F., Cheng, X., and Wang, T.: Heterogeneous Uptake of N2O5 in Sand Dust and Urban Aerosols Observed during the Dry Season in Beijing, Atmosphere, 10, 204, https://doi.org/10.3390/atmos10040204, 2019.
Xu, Z., Liu, M., Zhang, M., Song, Y., Wang, S., Zhang, L., Xu, T., Wang, T., Yan, C., Zhou, T., Sun, Y., Pan, Y., Hu, M., Zheng, M., and Zhu, T.: High efficiency of livestock ammonia emission controls in alleviating particulate nitrate during a severe winter haze episode in northern China, Atmos. Chem. Phys., 19, 5605–5613, https://doi.org/10.5194/acp-19-5605-2019, 2019.
Yang, X., Wang, T., Xia, M., Gao, X., Li, Q., Zhang, N., Gao, Y., Lee, S., Wang, X., Xue, L., Yang, L., and Wang, W.: Abundance and origin of fine particulate chloride in continental China, Sci. Total Environ., 624, 1041–1051, https://doi.org/10.1016/j.scitotenv.2017.12.205, 2018.
Yang, X., Wang, Q., Ma, N., Hu, W., Gao, Y., Huang, Z., Zheng, J., Yuan, B., Yang, N., Tao, J., Hong, J., Cheng, Y., and Su, H.: The impact of chlorine chemistry combined with heterogeneous N2O5 reactions on air quality in China, Zenodo [data set], https://doi.org/10.5281/zenodo.5957287, 2021.
Ye, C., Yuan, B., Lin, Y., Wang, Z., Hu, W., Li, T., Chen, W., Wu, C., Wang, C., Huang, S., Qi, J., Wang, B., Wang, C., Song, W., Wang, X., Zheng, E., Krechmer, J. E., Ye, P., Zhang, Z., Wang, X., Worsnop, D. R., and Shao, M.: Chemical characterization of oxygenated organic compounds in the gas phase and particle phase using iodide CIMS with FIGAERO in urban air, Atmos. Chem. Phys., 21, 8455–8478, https://doi.org/10.5194/acp-21-8455-2021, 2021.
Yu, C., Wang, Z., Xia, M., Fu, X., Wang, W., Tham, Y. J., Chen, T., Zheng, P., Li, H., Shan, Y., Wang, X., Xue, L., Zhou, Y., Yue, D., Ou, Y., Gao, J., Lu, K., Brown, S. S., Zhang, Y., and Wang, T.: Heterogeneous N2O5 reactions on atmospheric aerosols at four Chinese sites: improving model representation of uptake parameters, Atmos. Chem. Phys., 20, 4367–4378, https://doi.org/10.5194/acp-20-4367-2020, 2020.
Zhang, T., de Jong, M. C., Wooster, M. J., Xu, W., and Wang, L.: Trends in eastern China agricultural fire emissions derived from a combination of geostationary (Himawari) and polar (VIIRS) orbiter fire radiative power products, Atmos. Chem. Phys., 20, 10687–10705, https://doi.org/10.5194/acp-20-10687-2020, 2020.