the Creative Commons Attribution 4.0 License.
the Creative Commons Attribution 4.0 License.
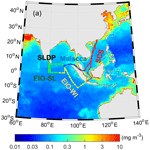
Molecular and spatial distributions of dicarboxylic acids, oxocarboxylic acids, and α-dicarbonyls in marine aerosols from the South China Sea to the eastern Indian Ocean
Jing Yang
Wanyu Zhao
Lianfang Wei
Qiang Zhang
Yue Zhao
Libin Wu
Xiaodong Li
Chandra Mouli Pavuluri
Xiaole Pan
Zifa Wang
Cong-Qiang Liu
Kimitaka Kawamura
Marine aerosol samples collected from the South China Sea (SCS) to the eastern Indian Ocean (EIO) during a cruise from 10 March to 26 April 2015 were studied for diacids and related compounds. In view of air mass backward trajectories, source regions, and geographical features, the cruise area was categorized into the South China Sea (SCS), the eastern Indian Ocean off the coast of western Indonesia (EIO-WI), the EIO off the coast of Sri Lanka (EIO-SL), Malacca, and the Sri Lanka docking point (SLDP). Total concentrations of diacids, oxoacids, and α-dicarbonyls were high at the SLDP, followed by the SCS and Malacca, and they were the low in the EIO-WI. In this study, oxalic acid (C2) was the dominant diacid during the cruise, followed by malonic acid (C3) in the SCS, EIO-WI, EIO-SL, and Malacca, and succinic acid (C4) was relatively more abundant than C3 diacid at the SLDP. Except for SLDP, C3∕C4 mass ratios were always greater than 1, and no significant difference was observed during the cruise. The C2∕C4 and C2∕total diacid ratios also showed similar trends. The average mass ratios of adipic acid (C6) to azelaic acid (C9) were less than unity except for in the EIO-WI; the mass ratios of phthalic acid (Ph) to azelaic acid (C9) were less than 2 except for in the SCS. The concentrations of diacids were higher when the air masses originated from terrestrial regions than when they originated from remote oceanic regions. Based on the molecular distributions of organic acids, the mass ratios, and the linear correlations of selected compounds in each area, we found that the oxidation of biogenic volatile organic compounds (BVOCs) released from the ocean surface and subsequent in situ photochemical oxidation was the main contributor to diacids, oxocarboxylic acids, and α-dicarbonyls from the SCS to the EIO. In addition, the continental outflow, which is enriched in anthropogenic VOCs and their aged products, influenced the organic aerosol loading, particularly over the SCS. Emissions from Sri Lanka terrestrial vegetation as well as fossil fuel combustion and subsequent photochemical oxidation also played a prominent role in controlling the organic aerosol loading and the molecular distribution of diacids and related compounds at the SLDP.
- Article
(6777 KB) -
Supplement
(725 KB) - BibTeX
- EndNote
Land–sea–air interaction is one of the most important issues in Earth system science. Atmospheric aerosols are one of the major components in the Earth's atmosphere and are the key carriers of the global biogeochemical cycle of nutrients (Zhuang et al., 1992; Li et al., 2017; Tan et al., 2011). Atmospheric aerosols containing inorganic and organic materials play a vital role in air quality, atmospheric chemistry, and the health of humans and ecosystems, and they have a critical impact on the global climate system (Ramanathan et al., 2001). Organic aerosols in tropospheric regions account for up to 90 % of the total fine aerosol mass, and most of these aerosols are water soluble (Kanakidou et al., 2005). The abundant diacids, oxoacids, and α-dicarbonyls are reported to be the major fraction of water-soluble organic aerosols (Sorooshian et al., 2010; Ervens et al., 2011; Cong et al., 2015); these water-soluble aerosols enhance the capacity of aerosol particles to act as cloud condensation nuclei and ice nuclei in the atmosphere (Ervens et al., 2011; D. Zhao et al., 2016; Vergara-Temprado et al., 2017; Huang et al., 2018), whereas water-insoluble substances such as lipid-class compounds can reduce their hygroscopic activity (Kawamura et al., 2017).
It has been well established that aerosols in the marine atmosphere are influenced by emissions from the ocean surface, such as plankton activity (Cavalli et al., 2004; O'Dowd and De Leeuw, 2007; Facchini et al., 2008). Marine-emitted aerosols account for more than half of the global natural aerosol burden (1000–3000 Tg yr−1; Iii and Duce, 1988); thus, they significantly influence the Earth's climate system (Haywood, 1999). Previous studies on marine aerosols have mainly focused on the measurement of non-sea-salt and sea-salt sulfates (Charlson et al., 1987, 1992) and mineral dust (Schulz et al., 2012). However, diacids and related compounds in marine aerosols have been paid little attention, despite the fact that they account for more than 10 % of total carbon (TC) in remote oceanic regions (Kawamura and Sakaguchi, 1999; Wang and Kawamura, 2006), which highlights their importance in the marine atmosphere.
Generally, molecular distributions of dicarboxylic acids in atmospheric aerosols are characterized by the dominance of oxalic (C2) acid, followed by malonic (C3) and succinic (C4) acids (Fu et al., 2013a; Kawamura and Bikkina, 2016). They can be directly emitted from anthropogenic emissions, such as fossil fuel combustion (Kawamura and Kaplan, 1987) and biomass burning (Narukawa et al., 1999; Kawamura et al., 2013), and can also be formed by the atmospheric oxidation of various volatile organic compounds (VOCs) emitted from primary sources (Kawamura et al., 1996a). In addition, unsaturated fatty acids emitted from oceanic biota can be further photo-oxidized to form the corresponding diacids in the atmosphere (Kawamura and Sakaguchi, 1999; Rinaldi et al., 2011). Isoprene, an abundant VOC from terrestrial higher plants as well as oceanic biota, is a major precursor of secondary organic aerosols (Lim et al., 2005; Carlton et al., 2009), including low-molecular-weight diacids such as C2–C4 (Nguyen et al., 2010).
Chemical characterization of dicarboxylic acids, oxoacids, and α-dicarbonyls in atmospheric aerosols provides useful knowledge on the relative contribution of possible primary sources, the long-range atmospheric transport, and the photooxidation routes of organic compounds (Kawamura and Bikkina, 2016). Many studies have investigated the impacts of continental air outflows on the concentration, composition, and distribution of organic aerosols in marine regions (Fu et al., 2013a). For example, a long-term observation of aerosols on Chichijima Island in the western North Pacific found a remarkable increase in the concentrations of dicarboxylic acids in winter and spring due to the influence of the East Asian air outflow conveyed by the westerlies (Mochida et al., 2003a). Moreover, organic compounds such as unsaturated fatty acids from marine sources are main contributors to the secondary formation of diacids and related compounds in the ocean atmosphere (Miyazaki et al., 2010; Kawamura and Gagosian, 1987). Bikkina et al. (2014) found that the abundance of C2–C6 and C9 diacids continued to increase with the increase in phytoplankton activity in the North Pacific Ocean. Meanwhile, the oxidation products of isoprene (e.g. pyruvic and glyoxylic acids) observed in the marine boundary layer had a similar concentration trend to that of C2, suggesting that the secondary oxidation of biogenic VOCs from marine sources significantly contributes to the atmospheric loading of diacids and related compounds.
The oceans cover more than 70 % of the Earth's surface, and marine aerosols are important components of the global aerosol system of natural sources. However, current knowledge about the biogeochemical cycles of organic matter in the tropical marine atmosphere is very limited. The South China Sea (SCS) is a large semi-closed marginal basin and one of the largest marginal seas in the world (Liu et al., 2002). The seasonal division of prevailing winds in the SCS is mainly influenced by the northeast monsoon from roughly mid-October to mid-March of the following year and by the southwest monsoon from mid-May to mid-September, while mid-March to mid-May is the spring transition period, during which the wind direction is variable. The climate of the SCS is part of the East Asian monsoon system (Lau et al., 1998). The Indian Ocean is the third largest ocean in the world, with distinct tropical maritime and monsoon climate characteristics. The prevailing wind over the Indian Ocean in summer is the southwest monsoon, while the prevailing wind in winter is the northeast monsoon (Fu et al., 2016; Ramanathan et al., 2005). The Indian Ocean is warmer than the Pacific and Atlantic oceans at the same latitude; thus, it is called the tropical ocean. The tropical eastern Indian Ocean (10∘ S–15∘ N, 65–100∘ E), including the southern Bay of Bengal, the southeastern Arabian Sea, the eastern equatorial Indian Ocean, and parts of the southern Indian Ocean, is one of the key regions affecting climate change, such as drought and flood, in China (Yao et al., 2015).
Although great progress has been made in the field of atmospheric organic aerosols, studies on diacids, oxoacids, and α-dicarbonyls in the marine atmosphere are scarce and have been limited to the Mediterranean, the North Pacific, the South Pacific, the Caribbean, and the Atlantic Ocean (Kawamura and Bikkina, 2016); thus, little is known about marine aerosols over the Indian Ocean. Studies on the molecular composition and distribution of dicarboxylic acids and related compounds can provide useful information for source analysis as well as information regarding the secondary formation and photochemical transformation processes of atmospheric organic aerosols. In this study, total suspended particle (TSP) samples collected on a day- and night-time basis over the SCS and the EIO were studied for diacids, oxoacids, and α-dicarbonyls, which provided an ideal opportunity to investigate the spatial distributions of marine organic aerosols influenced by organics from both marine emissions and long-range continental transport. In addition, carbonaceous components, i.e. organic carbon (OC), elemental carbon (EC), and water-soluble OC (WSOC), in the TSP samples were measured. Based on the molecular and spatial distributions, the mass ratios of organic compounds, and their relations with bulk components as well as backward air mass trajectories, we discuss their sources and possible formation pathways in the study region.
2.1 Marine aerosol sampling
Marine aerosol sampling was performed on the R/V “Shiyan 1” during cruise NORC2015-10 in the SCS and the EIO (Fig. 1) from 10 March to 26 April 2015. TSP samples were collected at daytime (n=44) and night-time (n=43) using a high-volume air sampler (Kimoto AS-810A, KIMOTO, Japan) placed on the front upper deck of the vessel's navigation room. The exhaust outlet of the ship was located at the stern, and the aerosol samples were collected underway to avoid potential pollution from the ship's emissions. The three samples collected at the Sri Lanka docking point (SLDP) when the ship was docked in the port were seriously polluted by ship exhaust emission. Therefore, these three samples are discussed separately for comparison with the other samples. The air sampler was operated at a flow rate of 1.0 m3 min−1 with pre-combusted (450 ∘C, 6 h) quartz filters (25 cm × 20 cm, Pallflex® TM, 2500 QAT-UP). We also collected field blanks (n=4) during the cruise. After sampling, the filter samples were wrapped in aluminium foil, packed in zip-lock bags, and stored in the dark at −20 ∘C until the chemical analysis.
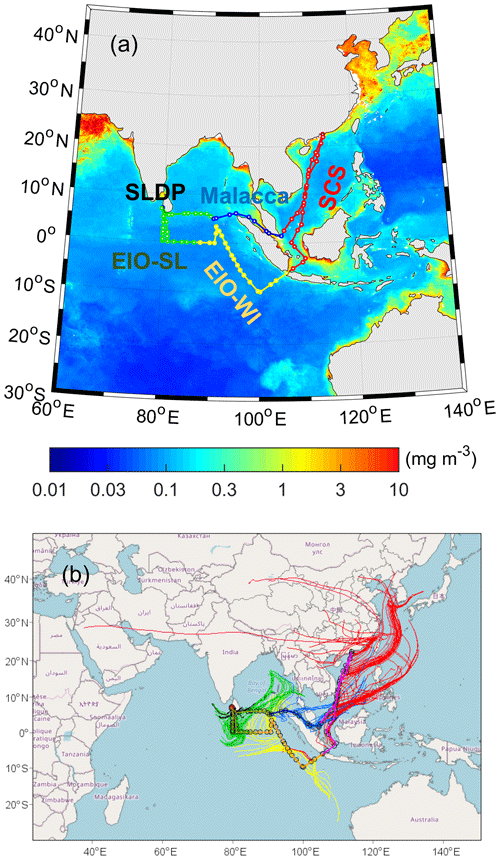
Figure 1(a) Cruise tracks used for aerosol collection in the South China Sea (SCS) and the eastern Indian Ocean (EIO) from 10 March to 26 April 2015. The red line is located in the SCS region, the yellow line is in the eastern Indian Ocean near western Indonesia (EIO-WI), the green line is in the eastern Indian Ocean near Sri Lanka (EIO-SL), the blue line is in the Malacca region, and the red solid points are the collection positions of the three Sri Lanka docking point (SLDP) samples. The base map (© MATLAB) reflects an average composite of Chlorophyll a concentrations from March to April 2015 obtained from the NASA website: https://modis.gsfc.nasa.gov/data/dataprod/chlor a.php (last access: 3 July 2019). (b) Air mass backward trajectories for the sampling days at an arrival height of 100 m; the map was produced using © MeteoInfo software. (Please note that the above figure contains disputed territories.)
The 5 d air mass backward trajectories arriving at 100 m a.s.l. (metres above sea level) each day were computed using the HYSPLIT (https://www.ready.noaa.gov/HYSPLIT.php, last access: 17 March 2019) model (Fig. 1). The air masses that arrived over the South China Sea (SCS) originated from East Asia and the surrounding oceanic regions. The air masses that arrived over the eastern Indian Ocean off the coast of western Indonesia (EIO-WI) originated from the remote EIO, whereas those that arrived over the eastern Indian Ocean off the coast of Sri Lanka (EIO-SL) were mixed from the remote EIO and the Bay of Bengal. The air masses that arrived over Malacca were from the Southeast Asian subcontinent and the surrounding EIO. In addition, the vessel was docked at the Sri Lanka docking point (SLDP) for 3 d, during which the air masses were mainly from the surrounding oceanic regions. Thus, the aerosol samples collected in these different locales during the cruise may have been affected by long-range atmospheric transport; hence, we segregated the TSP samples into five categories: SCS, EIO-WI, EIO-SL, Malacca, and SLDP (Fig. 1).
2.2 Determination of diacids and related compounds
Aerosol samples were determined for water-soluble organic acids using a previously reported method (Kawamura, 1993). Briefly, small pieces (47 mm in diameter) of TSP filter samples were extracted three times using organic-free Milli-Q water (10 mL) under ultrasonication. The extracts were then concentrated to dryness and reacted with 14 % BF3/n-butanol at 100 ∘C for 1 h. The derivatized acids and carbonyls were extracted with n-hexane and injected into a split/splitless gas chromatograph (GC-FID, Agilent 6980) equipped with a HP-5 column for the determination of diacids and related compounds. The field blank filters were also analysed using the same experimental procedure. Recoveries of major organic acids were better than 85 %. The analytical errors in the duplicate analysis were within 10 % for major species. Concentrations of diacids and related compounds were corrected according to the field blanks.
2.3 Measurements of WSOC, OC, and EC
For WSOC measurement, 17.3 or 34.7 cm2 of each filter was extracted with Milli-Q water (20 mL) under ultrasonication for 20 min; the extracts were analysed using a total organic carbon (TOC) analyser (TOC-L, 5000A, Shimadzu, Japan). OC and EC were determined by a carbon analyser (Sunset Laboratory Inc., USA), using a piece (1.5 cm2) of each filter, following the Interagency Monitoring of Protected Visual Environments (IMPROVE) protocol (Pavuluri et al., 2011). The limits of detection (LODs) for both OC and EC were 0.1 µg C cm−2 with a precision of greater than 10 %. The concentrations of all of the carbonaceous components reported here were corrected using the field blanks.
Table 1 shows the concentration levels of OC and EC in TSP samples collected from different marine regions. The concentration of EC (4.24±3.02 µg m−3) in the SLDP aerosols was much higher than the concentrations in other regions (SCS was 0.27±0.15 µg m−3, EIO-WI was 0.05±0.05 µg m−3, EIO-SL was 0.04±0.18 µg m−3, and Malacca was 0.24±0.18 µg m−3), which proved that the SLDP samples were seriously polluted by ship exhaust, but pollution from exhaust in other sea areas should be minor.
Table 1Summary of concentrations (ng m−3) of dicarboxylic acids, oxocarboxylic acids, and α-dicarbonyls as well as concentrations (µg m−3) of EC and OC in TSP samples collected during the NORC2015-10 cruise from the South China Sea (SCS) to the eastern Indian Ocean (EIO).
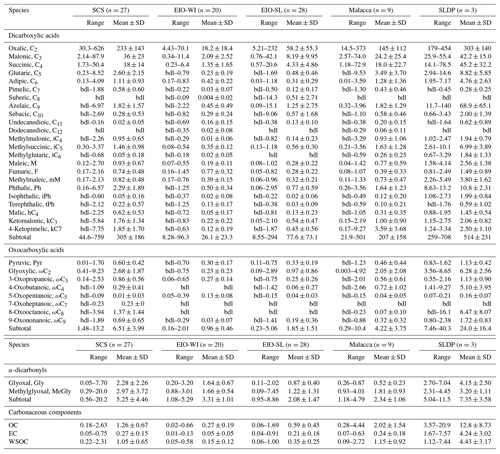
“bdl” represents that a compound was below the limit of detection, which is ca. 0.005 ng m−3 for the target compounds.
3.1 OC, EC, and WSOC concentrations in marine aerosols
The concentrations of OC, EC, and WSOC in each marine region (SCS, EIO-WI, EIO-SL, Malacca, and SLDP) are summarized in Table 1. In the samples collected from the SCS to the EIO from March to April 2015, the mass concentrations of OC and EC varied from 0.02 to 20.9 µg m−3 (mean of 1.29±2.72 µg m−3) and from 0.00 to 7.57 µg m−3 (mean of 0.33±0.90 µg m−3) respectively. Generally, the mass concentrations of OC and EC at the SLDP were the highest, followed by Malacca and SCS, and the lowest values were found in the EIO-WI and EIO-SL. In this study, the mean mass concentrations of OC and EC in the SCS were 1.5 times (OC mean of 0.82 µg m−3) and 1.7 times (EC mean of 0.16 µg m−3) higher than those reported by Fu et al. (2013a) in the SCS in late February 1990. As the sampling season was the same, the above situation indicated that the input of anthropogenic emissions to the SCS carbon aerosols was increasing with the economic development of the areas surrounding the SCS. The mass concentrations of OC and EC in the SCS were lower than those in the western SCS (OC of 2.04 µg m−3 and EC of 3.0 µg m−3) which were strongly affected by biomass burning (Song et al., 2018). In addition, the carbonaceous aerosol levels in Malacca were similar to those in the Bay of Bengal (OC of 1.9 µg m−3 and EC of 0.4 µg m−3; Kumar et al., 2008). The concentrations of carbon components at the SLDP were relatively close to that of Jeju Island (OC of 11.3 µg m−3 and EC of 3.0 µg m−3; Jung and Kawamura, 2011), and were higher than that of Sapporo Island (OC of 6.1 µg m−3 and EC of 1.9 µg m−3; Simoneit et al., 2004); the above-mentioned two sites were greatly affected by terrestrial long-range transmission. Meanwhile, the concentrations of carbon components in the EIO-WI and EIO-SL were lower than those in the northern Atlantic (OC of 0.93 µg m−3 and EC of 0.27 µg m−3; Zhang et al., 2010), the Pacific (OC of 0.82 µg m−3 and EC of 0.22 µg m−3; Zhang et al., 2010), Amsterdam Island (OC of 0.24 µg m−3 and EC not detected; Claeys et al., 2010), the Arctic Ocean (OC of 0.56 µg m−3; Fu et al., 2013b), and so on. These locations were all less affected by terrestrial anthropogenic emissions. The concentration levels of carbonaceous components in the EIO-WI and EIO-SL were lower than those in the Indian Ocean (OC of 0.71 µg m−3 and EC of 0.49 µg m−3; Fu et al., 2011).
EC is the product of residential coal combustion, fossil fuel combustion, and the incomplete combustion of biomass, whereas OC contains both primary organic carbon and secondary organic aerosol generated by the photochemical reactions of organic gases in the atmosphere. The concentration of EC in SLDP aerosol (4.24±3.02 µg m−3) was much higher than that in other marine areas (SCS was 0.27±0.15 µg m−3, EIO-WI was 0.05±0.05 µg m−3, EIO-SL was 0.21±0.18 µg m−3, and Malacca was 0.24±0.18 µg m−3), indicating that the aerosol samples from the SLDP were seriously polluted by ship exhaust, but the other marine samples were not. OC and EC can roughly determine the source of aerosols. There was a weak correlation between the concentration of OC and EC in aerosols from the SCS to the EIO (R2=0.34, P<0.001), indicating that the origins of organic aerosols were different from those of EC and were affected by multiple sources (Turpin and Huntzicker, 1995). In addition, the OC ∕ EC ratio can be used to indicate the contribution of different sources (Chow et al., 1996). The contribution of different emission sources, the influence of secondary organic aerosol formation, and the removal path of OC and EC can all affect the OC ∕ EC ratio. When the OC ∕ EC ratio is greater than 2, it indicates the presence of secondary organic aerosols. The OC ∕ EC ratio was 5.52±5.33 (range of 1.02–29.7) in the SCS, 11.84±17.44 (range of 1.55–51.0) in the EIO-WI, 0.96±2.33 (range of 0.96–9.28) in the EIO-SL, 8.76±6.84 (range of 2.71–24.4) in Malacca, and 2.98±0.97 (range of 2.14–4.04) at the SLDP. Among these values, the ratio of OC ∕ EC in the SCS was very similar to that measured by Fu et al. (2013a) in TSP samples collected in the SCS (OC ∕ EC: 5.1). The above results indicate that the contribution of secondary organic aerosols was widespread in aerosol samples from the SCS to the EIO, except for some samples in EIO-WI and EIO-SL.
The concentrations of WSOC were highest (range of 1.12–7.44 ng m−3 and mean of 4.43±3.17 ng m−3) at the SLDP followed by Malacca (range of 0.09–2.72 ng m−3 and mean of 1.15±0.92 ng m−3), the SCS (range of 0.22–2.31 ng m−3 and mean of 1.05±0.65 ng m−3), the EIO-SL (range of 0.06–1.00 ng m−3 and mean of 0.35±0.25 ng m−3), and the EIO-WI (range of 0.05–0.58 ng m−3 and mean of 0.15±0.12 ng m−3). The WSOC concentrations from the SCS to EIO are much lower than those in urban aerosols from Tokyo (mean of 13 µg m−3; Sempéré and Kawamura, 1994) and in biomass burning aerosols from Amazonia (18–51 µg m−3; Kundu et al., 2010a). However, WSOC concentrations in the EIO-SL are comparable to those reported in marine aerosols from the central Pacific (mean of 0.30 µg m−3; Hoque and Kawamura, 2016), the western Pacific (mean of 0.33 µg m−3; Sempéré and Kawamura, 2003), and Hawaii (mean of 0.39 µg m−3; Hoffman and Duce, 2013). Kawamura et al. (2010) determined lower WSOC concentrations (range of 0.04–0.30 µg m−3 and a mean of 0.18 µg m−3) in Arctic aerosols, which are comparable to those observed in the EIO-WI.
3.2 Concentrations and molecular distributions of dicarboxylic acids and related compounds
The concentrations of dicarboxylic acids, oxocarboxylic acids, and α-dicarbonyls in each marine region (the SCS, EIO-WI, EIO-SL, Malacca, and the SLDP) are summarized in Table 1. Their chemical structures are shown in Fig. S1 in the Supplement. Most of the organic species were found to be more abundant at the SLDP followed by the SCS, Malacca, the EIO-WI, and the EIO-SL (Table 1). For the aerosol samples from the SCS, the 5 d backward trajectories (Fig. 1b) showed that the air masses originated from East Asia, whereas the air masses that arrived at Malacca were delivered from Southeast Asia. The concentrations of diacids and related compounds were highest in the SCS followed by Malacca, due to increased anthropogenic inputs conveyed by long-range atmospheric transport from East Asia (Y. Zhao et al., 2016) and Southeast Asia respectively. The C2 diacid showed the highest abundance, with the abundance of the other components (see Table 1 for full component abbreviations) showing the following orders for the different locations: over the SCS (Fig. 2a); over the EIO-WI (Fig. 2b); over the EIO-SL; and over Malacca. The dominance of C2 followed by the C3 and C4 diacids is consistent with coastal marine aerosols (Kundu et al., 2010b; Kunwar and Kawamura, 2014) and remote marine aerosols from the western North Pacific (Bikkina et al., 2015), suggesting similar formation processes for dicarboxylic acids in the atmosphere of coastal or remote regions.
Table 1 shows the total concentrations of diacids in marine aerosols from the five regions in this study (the SCS was 0.27±0.15 ng m−3, the EIO-WI was 0.05±0.05 ng m−3, the EIO-SL was 0.04±0.18 ng m−3, Malacca was 0.24±0.18 ng m−3, and the SLDP was 0.27±0.15 ng m−3). Fu et al. (2013a) reported that the mean concentrations of total diacids in marine aerosol samples in the SCS and the Indian Ocean were 489 and 301 ng m−3 respectively. By comparison of this study with aerosols from other marine areas, it was found that the mean concentration of total diacids in the EIO was similar to that in the western Pacific Ocean (60±39 ng m−3; Wang et al., 2006). Furthermore, the concentrations of total diacids from the five sea areas in this study were all lower than that reported for the East China Sea (850 ng m−3; Mochida et al., 2003b) and higher than that reported for the Southern Ocean (4.5±4.0 ng m−3; Wang et al., 2006). In addition, the concentrations of total diacids in aerosol samples in all sea areas in this study were higher than that reported at Alert in the Canadian High Arctic (25 ng m−3; Kawamura et al., 1996a) and lower than that reported in urban aerosol from Xi'an, China, during different dust events (932–2240 ng m−3; Wang et al., 2015) and in aerosol in the megacity of Chennai in India (694.5±176.3 and 640.6±150.6 ng m−3 in early and late winter respectively and in summer 502.9±117.9 ng m−3; Pavuluri et al., 2010). Combined with the geographical location of the SLDP and the fact that the samples were collected in the ship's docking port, it is speculated that the organic aerosol samples from SLDP may have been affected by local coastal and terrestrial biological sources and anthropogenic emissions (especially fossil fuel combustion). The diacid abundance order () observed at SLDP was similar to that reported in continental aerosols (Kawamura and Bikkina, 2016).
3.2.1 Dicarboxylic acids
C2–C4, C6, C9, and phthalic (Ph) acids are important species of dicarboxylic acids; this section briefly summarizes the main sources and/or formation processes of these organic acids. C2 diacid concentrations ranged from 4.43 to 70.1 ng m−3 with a mean of 18.2±18.4 ng m−3 in the EIO-WI, from 5.21 to 232 ng m−3 with a mean of 58.2±55.3 ng m−3 in the EIO-SL, from 14.5 to 373 ng m−3 with a mean of 145±112 ng m−3 in Malacca, from 30.3 to 626 ng m−3 with a mean of 233±143 ng m−3 in the SCS, and from 179 to 454 ng m−3 with a mean of 303±140 ng m−3 at the SLDP. It can clearly be seen that the concentrations of C2 acid in the SCS and Malacca were higher than those in the EIO-SL and EIO-WI (Table 1). This large amount of C2 could have been generated from the following sources: fossil fuel combustion (Kawamura and Kaplan, 1987; Donnelly et al., 2010), biomass combustion (Schauer et al., 2001; Narukawa et al., 1999), cooking emissions (Kawamura and Kaplan, 1987; Rogge et al., 1993), and the photooxidation of VOCs and other precursors (Kawamura and Yasui, 2005; Kundu et al., 2010b). The diurnal variation trend of C2 acid was similar to that of C3 and C4 acids, indicating that these compounds may have had similar photochemical oxidation pathways or emission sources in the atmosphere.
C9 diacid was found to be the most abundant diacid in the range of C7–C11 diacids, which is similar to findings on marine aerosols from the southern and western Pacific Ocean (Wang et al., 2006). Such a high abundance of C9 diacid indicates that marine biogenic emissions and the subsequent photochemical formation of organic aerosols were likely significant over the SCS and EIO, as C9 diacid is a photochemical oxidation product of unsaturated fatty acids emitted from productive marine regions (Bikkina et al., 2014; Hoque et al., 2017). In addition, the photochemical oxidation of C9 diacid leads to the generation of its lower homologues including C4–C6 diacids (Yang et al., 2008; Kawamura et al., 1996a). Thus, the similar abundance of diacids (C2–C6 and C9) in the SCS and at Malacca (Fig. 3) suggests that marine biogenic emission was a major source, although the influence of the continental air masses transported from East and Southeast Asia was also significant. However, the concentrations of diacids over Malacca were relatively low, which indicated that the influence of polluted air masses transported from Southeast Asia was lower than that from air masses from East Asia. In general, the abundance of diacids in the EIO-SL and EIO-WI regions was lower than that in the SCS and Malacca regions. This was likely because the air masses that arrived over the EIO-SL and EIO-WI regions mostly originated from remote oceanic regions; thus, marine biogenic emission should have been the major potential source of organic aerosols and the Asian outflow should not have had a significant influence. Interestingly, the concentrations of C9 diacid (mean of 68.9±65.1 ng m−3) in the SLDP samples were several times higher than that observed in other regions over the SCS and EIO. As noted above, C9 diacid is mostly derived from unsaturated fatty acids of biogenic origin and has been considered as a marker for biogenic organic aerosols (Kawamura and Gagosian, 1987). Therefore, the high concentration of C9 diacid at the SLDP is likely derived from the oxidation of unsaturated fatty acids from mainland biota (Zhao et al., 2018). In fact, terrestrial air masses, which are enriched with higher plant emissions and secondary organic aerosol (SOA) produced upon subsequent oxidation of BVOCs during daytime, are likely transported onshore by land breezes during night-time (Kang et al., 2017). In addition, the photochemical breakdown of C9 diacid should be insignificant during the night-time hours.
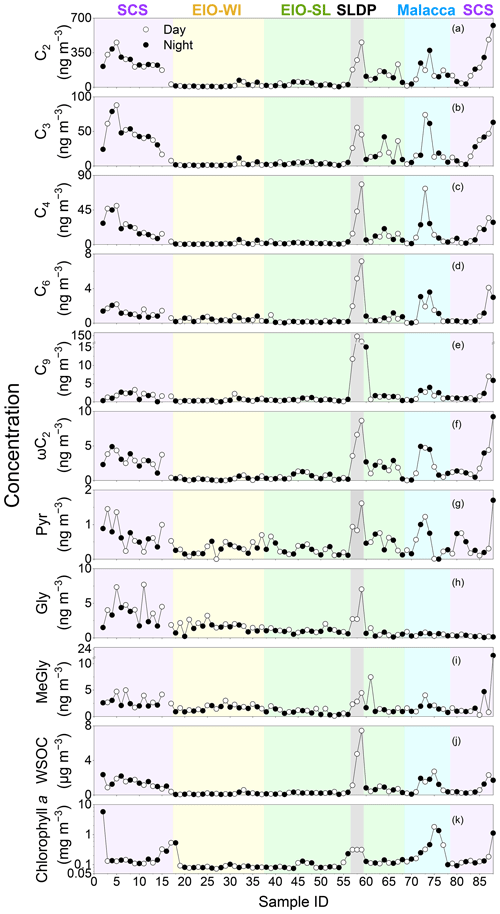
Figure 3Daily variations in the concentrations of selected organic acids and water-soluble organic carbon (WSOC) in TSP aerosol in the South China Sea and the eastern Indian Ocean from 10 March to 26 April 2015; all parameters are in nanograms per cubic metre (ng m−3) except for WSOC, which is in micrograms per cubic metre (µg m−3) and chlorophyll a, which is in milligrams per cubic metre (mg m−3).
Figure 3 shows the temporal variations in selected diacids over the SCS and EIO during the sampling periods. The spatial patterns of C6 and C9 diacids were similar (Fig. 3). C6 diacid could be produced via the oxidation of cyclic olefins emitted from anthropogenic sources (e.g. fossil fuel combustion; Kawamura et al., 1996a), and it could also be derived from photochemical breakdown of biogenic C9 diacid (Kawamura et al., 1996b). Bikkina et al. (2015) concluded that the high mass concentrations of C9 in marine aerosols were consistent with the high biological activity at the ocean surface. This similar temporal variation in C6 and C9 diacids and the results of backward trajectories (Fig. 1b) indicate that the formation of C6 diacid is mostly from the photochemical breakdown of C9 diacid, which was likely derived from marine biogenic emissions. Based on the ultraviolet (UV) irradiation of oleic acid and the ozone system, Matsunaga et al. (1999) found a series of C2–C9 diacids, with high abundance of C9 diacid. Temporal variations in C9, C6, and C2–C4 diacids were also similar (Fig. 3), suggesting that the photochemical breakdown of C9 might be the major formation pathway of short-chain diacids such as C6, C5, and C4 diacids. Further ageing processes eventually led to the formation of C2 diacid in the EIO. Thus, the molecular distributions and temporal variations of diacids inferred that the organic aerosols collected over the EIO were likely primarily derived from marine biogenic emissions. However, the contribution of diacids to WSOC was reduced in the EIO-WI and EIO-SL due to low biological activity in these oceanic regions, as reflected by the low C9 diacid loading.
Phthalic acid (Ph acid) is a tracer for anthropogenic organic aerosols. Concentrations of Ph acid in the EIO-WI and EIO-SL were similar to those in western North Pacific aerosol (more biologically influenced aerosols were 0.6 ng m−3 and less biologically influenced aerosols were 0.5 ng m−3; Bikkina et al., 2015). In addition, concentrations of Ph acid from the SCS to the EIO were much lower than those reported in the urban atmosphere, such as in Chennai (21 ng m−3; Pavuluri et al., 2010) and Chinese cities (90 ng m−3; Ho et al., 2007). Ph acid can be released directly from fossil fuel combustion (Kawamura and Kaplan, 1987). Secondary formation via the atmospheric oxidation of aromatic hydrocarbons such as naphthalene is also important (Fu et al., 2009; Ho et al., 2010). Such low levels of Ph acid suggested that the marine aerosols from the EIO were not as seriously impacted by continental air masses from East and Southeast Asia. It is worth noting that Ph was severalfold more abundant at the SLDP than in other regions (Table 1); therefore, we do not preclude the influence of anthropogenic emissions, particularly fossil fuel combustion, associated with the terrestrial air masses that arrived at the SLDP.
3.2.2 Oxocarboxylic acids
Oxocarboxylic acids (ωC2–ωC9 and pyruvic acid), the intermediate products of the oxidation of monocarboxylic acids, can be further oxidized to form diacids (Warneck, 2003; Carlton et al., 2007). Similar to diacids, oxocarboxylic acids are mainly derived from combustion sources, but they can also be produced by the photooxidation of various organic precursors from anthropogenic and biological sources in the atmosphere (Kawamura and Bikkina, 2016). The concentrations of total oxoacids ranged from 1.48 to 13.2 ng m−3 (mean of 6.51±3.99 ng m−3) in the SCS, from 0.16 to 2.01 ng m−3 (mean of 0.96±0.46 ng m−3) in the EIO-WI, from 0.23 to 5.06 ng m−3 (mean of 1.85±1.51 ng m−3) in the EIO-SL, from 0.29 to 10.4 ng m−3 (mean of 4.22±3.75 ng m−3) in Malacca, and from 7.46 to 40.3 ng m−3 (mean of 24.0±16.4 ng m−3) at the SLDP. Thus, the concentrations of total oxoacids in this study were lower than those from Gosan, Jeju Island, South Korea (mean of 53 ng m−3; Kawamura et al., 2004) and urban sites in China (45 ng m−3; Ho et al., 2007). The concentrations of oxoacids were also lower in the EIO-WI than in the other regions (Table 1). The spatial distributions of ω-oxoacids showed the following order with respect to location: SLDP > SCS > Malacca > EIO-SL > EIO-WI. This is consistent with those of major diacids (C2, C3, and C4) and indicates that these oxoacids are potential precursors of dicarboxylic acids (Sempéré and Kawamura, 1994).
Glyoxylic acid (ωC2) was the most abundant oxoacid followed by pyruvic (Pyr) acid (Table 1). These components are all important intermediates in photooxidation processes and are used in the production of low-carbon-number diacids such as C2, C3, and C4 diacids (Hatakeyama et al., 1987). Several studies have reported that Pyr is produced by the in-cloud oxidation of isoprene in the atmosphere (Carlton et al., 2006, 2009) which can be transported from mainland regions and/or emitted from the ocean surface (Shaw et al., 2010).
3.2.3 α-Dicarbonyls
The concentrations of total α-dicarbonyls (glyoxal and methylglyoxal) varied over a wide range (0.56–20.2 ng m−3) with a relatively high abundance in the SCS (mean of 5.25±4.46 ng m−3) and SLDP (mean of 7.35±3.58 ng m−3). The average concentration of total α-dicarbonyls during the cruise was higher than those in super-micrometre and sub-micrometre aerosols collected over the North Pacific in summer (0.51±0.22 ng m−3 in more biologically influenced aerosols and 0.66±0.20 ng m−3 in less biologically influenced aerosols; Miyazaki et al., 2010). The abundances of α-dicarbonyls were higher in the SCS and Malacca than in the EIO-SL and EIO-WI, which were similar to the spacial patterns of diacids and oxoacids (Fig. 2, Table 1). The concentrations of both glyoxal (Gly) and methylglyoxal (MeGly) were higher in the SCS, and their spatial distributions were consistent with those of diacids. Gly and MeGly can be formed via the atmospheric oxidation of isoprene, which is emitted from terrestrial vegetation and/or marine phytoplankton (Sorooshian et al., 2009). In addition, Gly is also a photochemical oxidation product of aromatic hydrocarbons from the combustion of fossil fuels (Carlton et al., 2007; Volkamer et al., 2007). Both species can exist in the aerosol phase and be further oxidized to form less-volatile organic acids such as Pyr, ωC2, and C2 (Sorooshian et al., 2006). Therefore, the higher abundances of Gly and MeGly over the SCS compared with the other three regions could have been due to enhanced continental outflow as well as the high biological activity at the sea surface. The spatial distributions of total oxoacids and α-dicarbonyls were similar to those of total diacids, indicating that they had similar origins or formation mechanisms (Kunwar et al., 2017). Based on the backward trajectory analysis, we identified four source regions for diacids and related compounds: (a) East Asia, (b) Southeast Asia, (c) the Bay of Bengal, and (d) the eastern Indian Ocean. When air masses originated from East Asia and Southeast Asia and their coastal areas (i.e. SCS and Malacca), the concentrations of these compounds were high; when air masses were mainly derived from the eastern Indian Ocean (i.e. EIO-WI), their concentrations was the lowest; and when the air masses originated from the Bay of Bengal and the eastern Indian Ocean (i.e. EIO-SL), the concentration of these compounds was between the former two.
3.2.4 Fractions of diacids and related compounds in WSOC and OC
Spatial–temporal distributions of WSOC showed much higher values in the SCS and Malacca than in the EIO-WI and EIO-SL regions (Fig. 3j). As discussed above, such a high abundance of WSOC in the SCS and Malacca may have been caused by the enhanced ageing of organic compounds from the surface ocean due to high biological activity and subsequent oxidation that produces more water-soluble organics. In this study, the spatial–temporal trends of diacids and related compounds are generally consistent with those of OC, EC, and WSOC, which are high in the SCS and low in other regions. The fractions of some major diacids, oxoacids, and α-dicarbonyls in WSOC and OC are presented in Table 2. The total diacids account for 12.0±5.75 % (range of 2.00 %–32.4 %) of WSOC over the EIO, whereas the fractions of oxoacids and α-dicarbonyls in WSOC ranged from 0.03 % to 1.22 % (mean of 0.34±0.20 %) and from 0.02 % to 3.12 % (mean of 0.60±0.68 %) respectively. In addition, a strong linear correlation was found between WSOC and C2 diacid (Table 2), which suggests that the WSOC was mainly generated via secondary formation rather than via biomass burning emissions associated with the Asian outflow.
3.3 Relative abundances of diacids and related compounds
The concentrations of diacids and related compounds and their relative abundances in the atmosphere are controlled by the emission of their precursors and subsequent oxidation processes. Pie diagrams of the percentage contributions of individual straight-chain diacids to total aliphatic diacids in the different regions from the SCS to EIO (SCS, EIO-WI, EIO-SL, Malacca, and SLDP) are depicted in Fig. 4. The percentage contributions of low-molecular-weight diacids, C2–C4, to total mass concentrations, Σ(C2–C12), varied from 96.4 % to 98.9 % (mean of 97.6±1.25 %) in the SCS, from 85.2 % to 96.0 % (mean of 90.6±5.38 %) in the EIO-WI, from 90.6 % to 99.4 % (mean of 95.0±4.44 %) in the EIO-SL, from 94.2 % to 98.0 % (mean of 96.1±1.88 %) in Malacca, and from 72.0 % to 95.0 % (mean of 83.5±11.5 %) at the SLDP. Interestingly, the relative abundances of C2 to the total mass concentrations of C2–C12 diacids, ΣC2–C12, were similar in four regions (Fig. 4). A significant difference was found in the relative abundance of C2 diacid between SLDP and the other four regions with the lowest value found at the SLDP, whereas C9 diacid showed the opposite behaviour.
Figure 5 presents the relative abundances of the sums of short-chain diacids (C2–C4), long-chain diacids (C5–C12), unsaturated diacids (M, F, mM, Ph, iPh, and tPh), diacids containing an additional functional group (hC4, kC3, and kC7), oxoacids (ωC2–ωC9 and Pyr), and α-dicarbonyls (MeGly and Gly) in the measured total diacids and related compounds in different regions from the SCS to EIO. The relative abundances of short-chain diacids were much higher, accounting for about 90 % of the total in the SCS and about 60 % in the EIO-WI. Long-chain diacids were found to be second highest at the SLDP and Malacca with respect to abundance, whereas α-dicarbonyls were second highest in the EIO-WI and EIO-SL; the highest abundances of long-chain diacids and α-dicarbonyls were observed at the SLDP and in the EIO-SL respectively (Fig. 5). Furthermore, the abundance of long-chain diacids was relatively high in the EIO-WI and EIO-SL but was the lowest in the SCS. The relative abundances of unsaturated diacids were the highest in the EIO-WI followed by SLDP, whereas oxoacids were highest in SLDP, followed by the EIO-WI, and showed the lowest values in the SCS and Malacca (Fig. 5). The high abundances of short-chain diacids indicate that the aerosols from the SCS to EIO were significantly aged during long-range transport, while the high abundances of long-chain diacids and α-dicarbonyls suggest that the contributions from biogenic emissions were much higher over the EIO and at SLDP. The high atmospheric levels of unsaturated fatty acids and oxoacids at the SLDP infer that the contributions from anthropogenic sources are also significant at this location.
3.4 Implications for the origins and formation pathways of diacids and related compounds
Different emission sources and the extent of ageing lead to large differences in the concentrations of diacids and related compounds; hence, the mass concentration ratios of selected species can serve as effective markers for the identification of their origins and formation/transformation processes. Temporal variations in the mass ratios of several organic acids as well as the relative abundance of C2 to total aliphatic diacids are depicted in Fig. 6. The spatial distributions of C3∕C4, C2∕C4, and ) ratios are similar to each other. It has been reported that C3 diacid can be produced from C4 diacid by oxidative reaction (Kawamura and Ikushima, 1993). There were robust relationships among C2, C3, and C4 diacids in SCS, EIO-WI, and EIO-SL regions and between C3 and C4 diacids in Malacca (Table S1 in the Supplement), suggesting that they were likely derived from similar sources. The C3∕C4 ratio can be used as an effective marker to assess the extent of organic aerosol ageing (Kawamura and Ikushima, 1993). The C3∕C4 ratios were reported to be low (0.25–0.44) in vehicular emissions, because the C3 diacid is too thermally unstable to be easily degraded during combustion (Kawamura and Kaplan, 1987). In contrast, the C3∕C4 ratios were reported to be high (range of 1.0–11 and a mean of 3.9) in marine aerosols collected from the North Pacific (including the tropics), which were considered to be photochemically aged during atmospheric transport (Kawamura and Sakaguchi, 1999). The C3∕C4 ratios in this study ranged from 0.3 to 4.6 with a mean value of 2.0. They are higher than those in urban aerosols from Chinese megacities (range of 0.6–1.1 and mean of 0.74; Ho et al., 2007), Tokyo (range of 0.56–2.9 and mean of 1.6; Kawamura and Ikushima, 1993), and New Delhi, India (range of 0.40–1.1 and mean of 0.66 at daytime and 0.58 at night-time; Miyazaki et al., 2009). It is worth noting that both malonic and succinic acids show a net loss from coastal areas to the open oceans, but the net loss is less for C3 diacid – which is potentially owing to photochemical processing (Fu et al., 2013a). Therefore, these higher C3∕C4 ratios suggest that organic aerosols from the SCS to EIO are subjected to significant, intensive photochemical ageing during atmospheric long-range transport via the outflows from East and Southeast Asia to remote oceanic regions (Fig. 1). Furthermore, the C3∕C4 ratios increased with the decrease of latitude over the SCS, suggesting that the photochemical transformation of C4 diacid to C3 diacid was enhanced at lower latitudes due to the increase in temperature and solar radiation. However, such a trend did not appear during the return voyage, and the C3∕C4 ratios were much lower than those reported over the North Pacific. In addition, the temporal variations in their concentrations were similar throughout the campaign. These results indicate that their in situ formation from the BVOCs (e.g. isoprene) emitted from marine biota might also be significant.
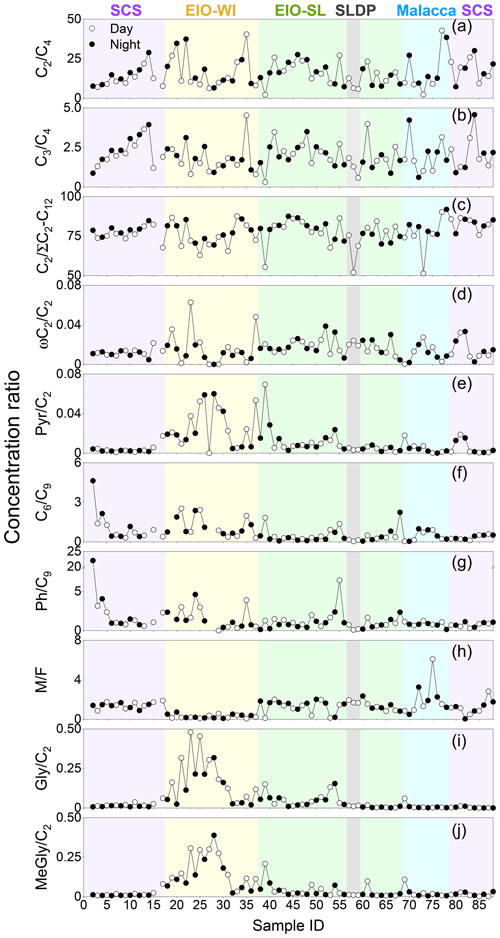
Figure 6Daily variations in the concentration ratios of (a) C2∕C4, (b) C3∕C4, (c) C2∕ΣC2–C12, (d) C2∕ωC2, (e) C6∕C9, (f) C6∕C9, (g) Ph∕C9, (h) M∕F, (i) C2∕Gly, and (j) C2∕MeGly in TSP samples collected during the NORC2015-10 cruise.
Table 3Diagnostic mass ratios of selected diacids and related compounds in TSP samples collected during the NORC2015-10 cruise.
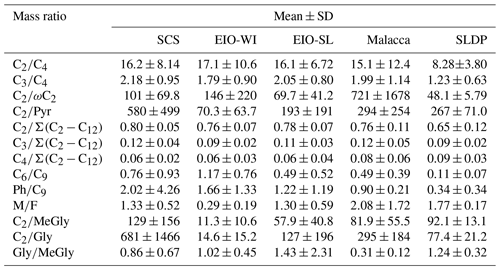
The C2∕C4 mass ratios can also be used to assess the extent of organic aerosols' ageing (Sorooshian et al., 2007). In the SCS, the C2/C4 ratios (7.6–28.9) showed an increasing trend with the decreasing latitude. The C2∕C4 ratios in the EIO were higher than those reported in Chinese cities (mean of 7.1; Ho et al., 2007), Sapporo (mean of 3.1; Aggarwal and Kawamura, 2008), and South Korea (mean of 8.6; Kundu et al., 2010b). These results indicate that C2 could be largely generated by the photochemical degradation of C4 diacid. Moreover, a significant correlation was found between the relative abundance of C2 (C2 %) and the C2∕C4 mass ratio (Fig. S2c), suggesting the potential formation of C2 diacid from the photochemical breakdown of C4 diacid. A concurrent trend (Fig. 6c) was also obtained for the increased contribution of C2 to total aliphatic diacids, , suggesting more photochemical ageing of organic aerosols at low latitudes with stronger solar radiation.
The ratio has also been considered as an indicator of the degree of organic aerosols' ageing during long-range atmospheric transport (Wang et al., 2006). In general, the more the aerosol particles are aged, the higher the ratios (Kawamura and Sakaguchi, 1999). The was higher (0.76±0.06) in the SCS than in the EIO-SL (0.74±0.08) and Malacca (0.71±0.11), followed by the EIO-WI (0.65±0.11) and SLDP (0.60±0.11). The values found in this study were also lower than the value reported in wintertime (0.8±0.04) and higher than that reported in summertime (0.5±0.01) in Himalayan aerosols (Hegde and Kawamura, 2012). These results indicate that the organic aerosols in the SCS were likely more aged and/or influenced by anthropogenic emissions from East Asia, whereas the ageing of organic aerosols was possibly less intensive and influenced by only marine biogenic emissions in the EIO-WI and Malacca. At the SLDP, the low ratio was likely driven by the local coastal biota and the terrestrial biogenic and anthropogenic emissions as well as the less ageing, particularly at night-time.
Maleic acid (M, cis form) is transformed to fumaric acid (F, trans form) with enhanced photochemical activity; hence, the M∕F mass ratio has been considered as a proxy to assess the degree of aerosol ageing (Kawamura and Sakaguchi, 1999). In general, low M∕F values reflect that secondary oxidation is an important source. M∕F ratios were found to be lower in the EIO-WI (0.07–0.73) than in other regions (0.9–2.3; Table 3). The mean M∕F value was 1.33 in samples collected in the SCS, 0.29 in the EIO-WI, 1.30 in the EIO-SL, 2.08 in Malacca, and 1.77 at the SLDP. They were all lower than those in Xi'an aerosols (summer value of 2.22 and winter value of 2.38), and they were about the same or less than those reported at the Korean Gosan site (spring value of 1.38, summer value of 0.76, autumn value of 1.62, and winter value of 2.21), which were considered to be aged. This indicates that the ageing of organic aerosols was more intensive in the EIO-WI, whereas in Malacca and SLDP, the organic aerosols were less aged and/or influenced by local emissions – thus, the cis–trans transformation was weak.
According to the description of the origins of C6, Ph, and C9 in the above sections, the C6∕C9 and Ph∕C9 ratios could provide insight into the relative strength of biogenic and anthropogenic sources in the given area. C6∕C9 ratios showed lower values in the EIO (except sample no. 2; Fig. 6f), which can be attributed to the large influence from marine air masses containing fatty acids like oleic acid, a precursor of C9 diacid (Kawamura and Gagosian, 1987). Temporal variations in the Ph∕C9 ratios from the SCS to EIO are shown in Fig. 6g, with a mean of 2.02 in the SCS, 1.56 in the EIO-WI, 1.22 in the EIO-SL, and 0.90 in Malacca. These values were lower than that (5.71) reported for wintertime Gosan aerosols from South Korea (Kundu et al., 2010a) but higher than the values reported for summertime (1.7) and wintertime (1.78) aerosols in Xi'an, China (Wang et al., 2012) and aerosols in the western Pacific (1.41; Sempéré and Kawamura, 2003). These comparisons suggest significant secondary formation of organic aerosols from anthropogenic precursors (e.g. naphthalene) transported from East and Southeast Asia (Fig. 1). However, C6 and C9 diacids showed a positive correlation (R=0.51, p<0.0001) among all samples, indicating that C6 diacid is likely also significantly derived from the photooxidation of BVOCs such as cyclic olefins. The higher Ph∕C9 ratios found over the SCS compared with other regions (Fig. 6g) again suggest that the contribution from fossil fuel combustion is also significant, which is associated with the continental outflow mainly over the SCS and slightly over the EIO.
The origins of MeGly, Gly, Pyr, and isoprene have been discussed in the above sections. The mass ratios such as Pyr∕C2, ωC2∕C2, Gly∕C2, and MeGly∕C2 can be used to explore the C2 formation pathway and/or its origin. Here, all the ratios were found to peak in the EIO-WI and/or EIO-SL regions (Fig. 6), where the clean oceanic air masses arrived at the sampling points (Fig. 1), whereas in the SCS, Malacca, and SLDP, these ratios were insignificant except for ωC2∕C2 (Fig. 6). These spatial distributions of the Pyr∕C2, ωC2∕C2, Gly∕C2, and MeGly∕C2 ratios indicate that the loading of organic aerosols in the EIO-WI and EIO-SL regions was likely influenced by marine biogenic emissions and their subsequent oxidation processes. In addition, there were good correlations among Pyr, ωC2, and MeGly in the SCS and Malacca, whereas the correlations were poor in the samples from EIO-WI and EIO-SL (except for Pyr and MeGly in EIO-WI and ωC2 and Pyr in EIO-SL). The results showed that organic aerosols produced by BVOCs (e.g. isoprene) emitted from the ocean surface were more aged in the EIO-WI and EIO-SL than in the SCS and at Malacca, where they were affected by anthropogenic emissions.
Finally, to better understand the enhanced emission of unsaturated fatty acids and BVOCs from the biologically active ocean surface from the SCS to EIO, we present the satellite image of chlorophyll-a loading in the EIO in Fig. 1a. The concentrations of chlorophyll a in the SCS and Malacca were higher than in the other regions studied. Interestingly, high concentrations of diacids and related compounds (Fig. 3) were consistent with high concentrations of chlorophyll a at the marine surface. This consistency indicates that the high abundance of diacids in the SCS and Malacca attributes to the oceanic emissions of BVOCs from areas of high biological activity followed by photooxidation, although the influence of anthropogenic emissions associated with the continental outflow cannot be excluded.
The dicarboxylic acids, oxocarboxylic acids, and α-dicarbonyls in marine aerosols collected from the SCS to EIO from 10 March to 26 April 2015 provided new insight into their origins and formation processes in tropical regions. The spatial distribution of diacids showed high concentrations in nearshore regions (the SCS, Malacca, and the SLDP) and relatively low concentrations in remote regions (the EIO-WI and EIO-SL). Their molecular distributions were characterized by the dominance of C2 diacid. Anthropogenic pollutants under Asian outflow conditions strongly influenced the concentrations of dicarboxylic acids in the SCS and Malacca, whereas oceanic biogenic emissions and subsequent oxidation influenced the distributions the above-mentioned species in the EIO-WI and EIO-SL. A close relationship between the temporal variability of C9, C6, and the total concentration of short-chain diacids (C2–C4) implied that the in situ secondary formation of long-chain diacids (including C3 and C4) and related compounds was significant from the SCS to EIO rather than the photochemical breakdown of higher diacids to their lower homologues (despite significant ageing of the air masses transported from the terrestrial region), due to the enhanced emission of VOCs from marine biota in the region. This finding was also supported by good linear relationships among C4, hC4, and C3 diacids. The positive correlations obtained between C2 % and the C2∕C4 ratios and the negative correlations obtained between C2 % and C3 % as well as C4 % indicated that the formation of C2 from C4 diacid via C3 is significant. Furthermore, the enhanced emissions of biogenic unsaturated fatty acids and VOCs from the ocean surface and subsequent photochemical oxidation controlled the high and low levels of diacids and related compounds measured in the SCS and Malacca and in the EIO-WI and EIO-SL respectively. These results combined with 5 d backward air mass trajectories and the satellite image of chlorophyll a suggest that the emission of BVOCs from the biologically active ocean surface and their subsequent oxidation reactions were more significant over the SCS and Malacca than that over the EIO-WI and EIO-SL regions. Finally, SLDP samples were affected by all of the sources mentioned above. In addition, terrestrial biogenic and anthropogenic emissions might have played a significant role in the loading of diacids and related compounds at the SLDP. Further work on the chemical characterization of the marine organic aerosols at a molecular level using high-solution mass spectrometry (MS), such as Orbitrap and Fourier-transform ion cyclotron resonance MS (FT-ICR-MS), are needed to obtain detailed information on sulfur-containing organics from both biogenic and anthropogenic sources.
The dataset used in this paper is presented in the tables and figures and is available upon request from the corresponding author (fupingqing@tju.edu.cn).
The supplement related to this article is available online at: https://doi.org/10.5194/acp-20-6841-2020-supplement.
PF designed the research. PF and WH supported the research. Samples were collected by LW. Laboratory analyses were performed by JY and WZ. The paper was written by JY, PF, and CMP with consultation from all co-authors.
The authors declare that they have no conflict of interest.
We sincerely thank the captain and crews of the R/V “Shiyan 1” that were involved with the NORC2015-10 open research cruise, which was supported by the NSFC Ship-time Sharing Project.
This research has been supported by the National Natural Science Foundation of China (grant nos. 41977183, 41625014, and 41805118).
This paper was edited by Aijun Ding and reviewed by two anonymous referees.
Aggarwal, S. G. and Kawamura, K.: Molecular distributions and stable carbon isotopic compositions of dicarboxylic acids and related compounds in aerosols from Sapporo, Japan: Implications for photochemical aging during long-range atmospheric transport, J. Geophys. Res.-Atmos., 113, D14301, https://doi.org/10.1029/2007JD009365, 2008.
Bikkina, S., Kawamura, K., Miyazaki, Y., and Fu, P.: High abundances of oxalic, azelaic, and glyoxylic acids and methylglyoxal in the open ocean with high biological activity: Implication for secondary OA formation from isoprene, Geophys. Res. Lett., 41, 3649–3657, https://doi.org/10.1002/2014gl059913, 2014.
Bikkina, S., Kawamura, K., and Miyazaki, Y.: Latitudinal distributions of atmospheric dicarboxylic acids, oxocarboxylic acids, and α-dicarbonyls over the western North Pacific: Sources and formation pathways, J. Geophys. Res.-Atmos., 120, 5010–5035, https://doi.org/10.1002/2014jd022235, 2015.
Carlton, A. G., Turpin, B. J., Lim, H. J., Altieri, K. E., Seitzinger, S., and Lim, H. J.: Link between isoprene and secondary organic aerosol (SOA): Pyruvic acid oxidation yields low volatility organic acids in clouds, Geophys. Res. Lett., 33, 272-288, 2006.
Carlton, A. M., J. Turpin, B., Altieri, K., Seitzinger, S., Reff, A., Lim, H.-J., and Ervens, B.: Atmospheric oxalic acid and SOA production from glyoxal: Results of aqueous photooxidation experiments, Atmos. Environ., 41, 7588–7602, https://doi.org/10.1016/j.atmosenv.2007.05.035, 2007.
Carlton, A. G., Wiedinmyer, C., and Kroll, J. H.: A review of Secondary Organic Aerosol (SOA) formation from isoprene, Atmos. Chem. Phys., 9, 4987–5005, https://doi.org/10.5194/acp-9-4987-2009, 2009.
Cavalli, F., Facchini, M. C., Decesari, S., Mircea, M., and 'Acqua, A. D.: Advances in characterization of size-resolved organic matter in marine aerosol over the North Atlantic, J. Geophys. Res.-Atmos., 109, 89–92, 2004.
Charlson, R. J., Lovelock, J. E., Andreae, M. O., and Warren, S. G.: Oceanic phytoplankton, atmospheric sulphur, cloud albedo and climate, Nature, 326, 655–661, 1987.
Charlson, R. J., Schwartz, S. E., Hales, J. M., Cess, R. D., Coakley, J. A., Hansen, J. E., and Hofmann, D. J.: Climate Forcing by Anthropogenic Aerosols, Science, 255, 423–430, 1992.
Chow, J. C., Watson, J. G., Lu, Z., Lowenthal, D. H., Frazier, C. A., Solomon, P. A., Thuillier, R. H., and Magliano, K.: Descriptive analysis of PM2.5 and PM10 at regionally representative locations during SJVAQS/AUSPEX, Atmos. Environ., 30, 2079–2112, 1996.
Claeys, M., Wang, W., Vermeylen, R., Kourtchev, I., Chi, X., Farhat, Y., Surratt, J. D., Gómez-González, Y., Sciare, J., and Maenhaut, W.: Chemical characterisation of marine aerosol at Amsterdam Island during the austral summer of 2006–2007, J. Aerosol Sci., 41, 13–22, 2010.
Cong, Z. Y., Kawamura, K., Kang, S. C., and Fu, P. Q.: Penetration of biomass-burning emissions from South Asia through the Himalayas: new insights from atmospheric organic acids, Sci. Rep., 5, 9580, https://doi.org/10.1038/srep09580, 2015.
Donnelly, T. H., Shergold, J. H., and Southgate, P. N.: Anomalous geochemical signals from phosphatic Middle Cambrian rocks in the southern Georgina Basin, Australia, Sedimentology, 35, 549–570, 2010.
Ervens, B., Turpin, B. J., and Weber, R. J.: Secondary organic aerosol formation in cloud droplets and aqueous particles (aqSOA): a review of laboratory, field and model studies, Atmos. Chem. Phys., 11, 11069–11102, https://doi.org/10.5194/acp-11-11069-2011, 2011.
Facchini, M. C., Rinaldi, M., Decesari, S., Carbone, C., Finessi, E., Mircea, M., Fuzzi, S., Ceburnis, D., Flanagan, R., and Nilsson, E. D.: Primary submicron marine aerosol dominated by insoluble organic colloids and aggregates, Geophys. Res. Lett., 35, L17814, https://doi.org/10.1029/2008gl034210, 2008.
Fu, P. Q., Kawamura, K., and Barrie, L. A.: Photochemical and other sources of organic compounds in the Canadian high arctic aerosol pollution during winter–spring, Environ. Sci. Technol., 43, 286–292, 2009.
Fu, P. Q., Kawamura, K., and Miura, K.: Molecular characterization of marine organic aerosols collected during a round-the-world cruise, J. Geophys. Res.-Atmos., 116, D13302, https://doi.org/10.1029/2011JD015604, 2011.
Fu, P. Q., Kawamura, K., Usukura, K., and Miura, K.: Dicarboxylic acids, ketocarboxylic acids and glyoxal in the marine aerosols collected during a round-the-world cruise, Mar. Chem., 148, 22–32, https://doi.org/10.1016/j.marchem.2012.11.002, 2013a.
Fu, P. Q., Kawamura, K., Chen, J., Charrière, B., and Sempéré, R.: Organic molecular composition of marine aerosols over the Arctic Ocean in summer: contributions of primary emission and secondary aerosol formation, Biogeosciences, 10, 653–667, https://doi.org/10.5194/bg-10-653-2013, 2013b.
Fu, P. Q., Aggarwal, S. G., Chen, J., Li, J., Sun, Y. L., Wang, Z. F., Chen, H. S., Liao, H., Ding, A. J., Umarji, G. S., Patil, R. S., Chen, Q., and Kawamura, K.: Molecular Markers of Secondary Organic Aerosol in Mumbai, India, Environ. Sci. Technol., 50, 4659–4667, https://doi.org/10.1021/acs.est.6b00372, 2016.
Hatakeyama, S., Ohno, M., Weng, J., Takagi, H., and Akimoto, H.: Mechanism for the formation of gaseous and particulate products from ozone-cycloalkene reactions in air, Environ. Sci. Technol., 21, 52–57, 1987.
Haywood, M. J.: Tropospheric Aerosol Climate Forcing in Clear-Sky Satellite Observations over the Oceans, Science, 283, 1299–1303, 1999.
Hegde, P. and Kawamura, K.: Seasonal variations of water-soluble organic carbon, dicarboxylic acids, ketocarboxylic acids, and α-dicarbonyls in Central Himalayan aerosols, Atmos. Chem. Phys., 12, 6645–6665, https://doi.org/10.5194/acp-12-6645-2012, 2012.
Ho, K., Cao, J., Lee, S., Kawamura, K., Zhang, R., Chow, J. C., and Watson, J. G.: Dicarboxylic acids, ketocarboxylic acids, and dicarbonyls in the urban atmosphere of China, J. Geophys. Res.-Atmos., 112, D22S27, https://doi.org/10.1029/2006JD008011, 2007.
Ho, K., Lee, S., Ho, S. S. H., Kawamura, K., Tachibana, E., Cheng, Y., and Zhu, T.: Dicarboxylic acids, ketocarboxylic acids, α-dicarbonyls, fatty acids, and benzoic acid in urban aerosols collected during the 2006 Campaign of Air Quality Research in Beijing (CAREBeijing-2006), J. Geophys. Res.-Atmos., 115, D19312, https://doi.org/10.1029/2009JD013304, 2010.
Hoffman, E. J. and Duce, R. A.: Organic carbon in marine atmospheric particulate matter: Concentration and particle size distribution, Geophys. Res. Lett., 4, 449–452, 2013.
Hoque, M. M. M. and Kawamura, K.: Longitudinal distributions of dicarboxylic acids, ω-oxoacids, pyruvic acid, α-dicarbonyls, and fatty acids in the marine aerosols from the central Pacific including equatorial upwelling, Global Biogeochem. Cy., 30, 534–548, https://doi.org/10.1002/2015gb005346, 2016.
Hoque, M. M. M., Kawamura, K., and Uematsu, M.: Spatio-temporal distributions of dicarboxylic acids, ω-oxocarboxylic acids, pyruvic acid, α dicarbonyls and fatty acids in the marine aerosols from the North and South Pacific, Atmos. Res., 185, 158–168, https://doi.org/10.1016/j.atmosres.2016.10.022, 2017.
Huang, W. T. K., Ickes, L., Tegen, I., Rinaldi, M., Ceburnis, D., and Lohmann, U.: Global relevance of marine organic aerosol as ice nucleating particles, Atmos. Chem. Phys., 18, 11423–11445, https://doi.org/10.5194/acp-18-11423-2018, 2018.
Iii, D. J. E. and Duce, R. A.: On the global flux of atmospheric sea salt, J. Geophys. Res.-Oceans, 93, 14079–14088, 1988.
Jung, J. and Kawamura, K.: Springtime carbon emission episodes at the Gosan background site revealed by total carbon, stable carbon isotopic composition, and thermal characteristics of carbonaceous particles, Atmos. Chem. Phys., 11, 10911–10928, https://doi.org/10.5194/acp-11-10911-2011, 2011.
Kanakidou, M., Seinfeld, J. H., Pandis, S. N., Barnes, I., Dentener, F. J., Facchini, M. C., Van Dingenen, R., Ervens, B., Nenes, A., Nielsen, C. J., Swietlicki, E., Putaud, J. P., Balkanski, Y., Fuzzi, S., Horth, J., Moortgat, G. K., Winterhalter, R., Myhre, C. E. L., Tsigaridis, K., Vignati, E., Stephanou, E. G., and Wilson, J.: Organic aerosol and global climate modelling: a review, Atmos. Chem. Phys., 5, 1053–1123, https://doi.org/10.5194/acp-5-1053-2005, 2005.
Kang, M. J., Yang, F., Ren, H., Zhao, W. Y., Zhao, Y., Li, L. J., Yan, Y., Zhang, Y. J., Lai, S. C., Zhang, Y. Y., Yang, Y., Wang, Z. F., Sun, Y. L., and Fu, P. Q.: Influence of continental organic aerosols to the marine atmosphere over the East China Sea: Insights from lipids, PAHs and phthalates, Sci. Total Environ., 607, 339–350, https://doi.org/10.1016/j.scitotenv.2017.06.214, 2017.
Kawamura, K.: Identification of C2–C10 ω-oxocarboxylic acids, pyruvic acid, and C2–C3 α-dicarbonyls in wet precipitation and aerosol samples by capillary GC and GC/MS, Anal. Chem., 65, 3505–3511, 1993.
Kawamura, K. and Bikkina, S.: A review of dicarboxylic acids and related compounds in atmospheric aerosols: Molecular distributions, sources and transformation, Atmos. Res., 170, 140–160, https://doi.org/10.1016/j.atmosres.2015.11.018, 2016.
Kawamura, K. and Gagosian, R. B.: Implications of w-oxocarboxylic acids in the remote marine atmosphere for photo-oxidation of unsaturated fatty acids, Nature, 325, 330–332, 1987.
Kawamura, K. and Ikushima, K.: Seasonal changes in the distribution of dicarboxylic acids in the urban atmosphere, Environ. Sci. Technol., 27, 2227–2235, 1993.
Kawamura, K. and Kaplan, I. R.: Motor exhaust as a primary source for dicarboxylic acids in Los Angeles ambient air, Environ. Sci. Technol., 21, 105–110, 1987.
Kawamura, K. and Sakaguchi, F.: Molecular distribution of water soluble dicarboxylic acids in marine aerosols over the Pacific Ocean including tropics, J. Geophys. Res.-Atmos., 104, 3501–3509, 1999.
Kawamura, K. and Yasui, O.: Diurnal changes in the distribution of dicarboxylic acids, ketocarboxylic acids and dicarbonyls in the urban Tokyo atmosphere, Atmos. Environ., 39, 1945–1960, 2005.
Kawamura, K., Kasukabe, H., and Barrie, L. A.: Source and reaction pathways of dicarboxylic acids, ketoacids and dicarbonyls in arctic aerosols: One year of observations, Atmos. Environ., 30, 1709–1722, 1996a.
Kawamura, K., Seméré R., Imai, Y., Fujii, Y., and Hayashi, M.: Water soluble dicarboxylic acids and related compounds in Antarctic aerosols, J. Geophys. Res.-Atmos., 101, 18721–18728, 1996b.
Kawamura, K., Kobayashi, M., Tsubonuma, N., Mochida, M., Watanabe, T., and Lee, M.: Organic and inorganic compositions of marine aerosols from East Asia: Seasonal variations of water-soluble dicarboxylic acids, major ions, total carbon and nitrogen, and stable C and N isotopic composition, in: The Geochemical Society Special Publications, Geochemical Investigations in Earth and Space Science: A Tribute to Isaac R. Kaplan, Elsevier, 243–265, 2004.
Kawamura, K., Kasukabe, H., and Barrie, L. A.: Secondary formation of water-soluble organic acids and α-dicarbonyls and their contributions to total carbon and water-soluble organic carbon: Photochemical aging of organic aerosols in the Arctic spring, J. Geophys. Res.-Atmos., 115, D21306, https://doi.org/10.1029/2010JD014299, 2010.
Kawamura, K., Tachibana, E., Okuzawa, K., Aggarwal, S. G., Kanaya, Y., and Wang, Z. F.: High abundances of water-soluble dicarboxylic acids, ketocarboxylic acids and a-dicarbonyls in the mountaintop aerosols over the North China Plain during wheat burning season, Atmos. Chem. Phys., 13, 8285–8302, https://doi.org/10.5194/acp-13-8285-2013, 2013.
Kawamura, K., Hoque, M. M. M., Bates, T. S., and Quinn, P. K.: Molecular distributions and isotopic compositions of organic aerosols over the western North Atlantic: Dicarboxylic acids, related compounds, sugars, and secondary organic aerosol tracers, Org. Geochem., 113, 229–238, https://doi.org/10.1016/j.orggeochem.2017.08.007, 2017.
Kumar, A., Sudheer, A., and Sarin, M.: Chemical characteristics of aerosols in MABL of Bay of Bengal and Arabian Sea during spring inter-monsoon: a comparative study, J. Earst Syst. Sci., 117, 325–332, 2008.
Kundu, S., Kawamura, K., Andreae, T. W., Hoffer, A., and Andreae, M. O.: Molecular distributions of dicarboxylic acids, ketocarboxylic acids and α-dicarbonyls in biomass burning aerosols: implications for photochemical production and degradation in smoke layers, Atmos. Chem. Phys., 10, 2209–2225, https://doi.org/10.5194/acp-10-2209-2010, 2010a.
Kundu, S., Kawamura, K., and Lee, M.: Seasonal variations of diacids, ketoacids, and α-dicarbonyls in aerosols at Gosan, Jeju Island, South Korea: Implications for sources, formation, and degradation during long-range transport, J. Geophys. Res., 115, D19307, https://doi.org/10.1029/2010jd013973, 2010b.
Kunwar, B. and Kawamura, K.: Seasonal distributions and sources of low molecular weight dicarboxylic acids, ω-oxocarboxylic acids, pyruvic acid, α-dicarbonyls and fatty acids in ambient aerosols from subtropical Okinawa in the western Pacific Rim, Environ. Chem., 11, 673–689, 2014.
Kunwar, B., Torii, K., and Kawamura, K.: Springtime influences of Asian outflow and photochemistry on the distributions of diacids, oxoacids and α-dicarbonyls in the aerosols from the western North Pacific Rim, Tellus B, 69, 1369341, https://doi.org/10.1080/16000889.2017.1369341, 2017.
Lau, K.-M., Wu, H.-T., and Yang, S.: Hydrologic processes associated with the first transition of the Asian summer monsoon: A pilot satellite study, B. Am. Meteorol. Soc., 79, 1871–1882, 1998.
Li, W. J., Xu, L., Liu, X. H., Zhang, J. C., Lin, Y. T., Yao, X. H., Gao, H. W., Zhang, D. Z., Chen, J. M., Wang, W. X., Harrison, R. M., Zhang, X. Y., Shao, L. Y., Fu, P. Q., Nenes, A., and Shi, Z. B.: Air pollution-aerosol interactions produce more bioavailable iron for ocean ecosystems, Sci. Adv., 3, e1601749, https://doi.org/10.1126/sciadv.1601749, 2017.
Lim, H.-J., Carlton, A. G., and Turpin, B. J.: Isoprene forms secondary organic aerosol through cloud processing: Model simulations, Environ. Sci. Technol., 39, 4441–4446, 2005.
Liu, K. K., Chao, S. Y., Shaw, P. T., Gong, G. C., Chen, C. C., and Tang, T. Y.: Monsoon-forced chlorophyll distribution and primary production in the South China Sea: observations and a numerical study, Deep-Sea Res. Pt. I, 49, 1387–1412, 2002.
Matsunaga, S., Kawamura, K., Nakatsuka, T., and Ohkouchi, N.: Preliminary study on laboratory photochemical formation of low molecular weight dicarboxylic acids from unsaturated fatty acid (oleic acid), Org. Geochem., 14, 19–25, 1999.
Miyazaki, Y., Aggarwal, S. G., Singh, K., Gupta, P. K., and Kawamura, K.: Dicarboxylic acids and water-soluble organic carbon in aerosols in New Delhi, India, in winter: Characteristics and formation processes, J. Geophys. Res.-Atmos., 114, D19206, https://doi.org/10.1029/2009JD011790, 2009.
Miyazaki, Y., Kawamura, K., and Sawano, M.: Size distributions and chemical characterization of water-soluble organic aerosols over the western North Pacific in summer, J. Geophys. Res.-Atmos., 115, D23210, https://doi.org/10.1029/2010JD014439, 2010.
Mochida, M., Kawabata, A., Kawamura, K., Hatsushika, H., and Yamazaki, K.: Seasonal variation and origins of dicarboxylic acids in the marine atmosphere over the western North Pacific, J. Geophys. Res.-Atmos., 108, 4193, https://doi.org/10.1029/2002JD002355, 2003a.
Mochida, M., Kawamura, K., Umemoto, N., Kobayashi, M., Matsunaga, S., Lim, H. J., Turpin, B. J., Bates, T. S., and Simoneit, B. R.: Spatial distributions of oxygenated organic compounds (dicarboxylic acids, fatty acids, and levoglucosan) in marine aerosols over the western Pacific and off the coast of East Asia: Continental outflow of organic aerosols during the ACE-Asia campaign, J. Geophys. Res.-Atmos., 108, 8638, https://doi.org/10.1029/2002JD003249, 2003b.
Narukawa, M., Kawamura, K., Takeuchi, N., and Nakajima, T.: Distribution of dicarboxylic acids and carbon isotopic compositions in aerosols from 1997 Indonesian forest fires, Geophys. Res. Lett., 26, 3101–3104, 1999.
Nguyen, T. B., Bateman, A. P., Bones, D. L., Nizkorodov, S. A., Laskin, J., and Laskin, A.: High-resolution mass spectrometry analysis of secondary organic aerosol generated by ozonolysis of isoprene, Atmos. Environ., 44, 1032–1042, 2010.
O'Dowd, C. D. and De Leeuw, G.: Marine aerosol production: a review of the current knowledge, Philos. T. Roy. Soc. A, 365, 1753–1774, 2007.
Pavuluri, C. M., Kawamura, K., Aggarwal, S. G., and Swaminathan, T.: Characteristics, seasonality and sources of carbonaceous and ionic components in the tropical aerosols from Indian region, Atmos. Chem. Phys., 11, 8215–8230, https://doi.org/10.5194/acp-11-8215-2011, 2011.
Pavuluri, C. M., Kawamura, K., and Swaminathan, T.: Water-soluble organic carbon, dicarboxylic acids, ketoacids, and α-dicarbonyls in the tropical Indian aerosols, J. Geophys. Res.-Atmos., 115, D11302, https://doi.org/10.1029/2009JD012661, 2010.
Ramanathan, V., Crutzen, P. J., Kiehl, J. T., and Rosenfeld, D.: Atmosphere – Aerosols, climate, and the hydrological cycle, Science, 294, 2119–2124, 2001.
Ramanathan, V., Chung, C., Kim, D., Bettge, T., Buja, L., Kiehl, J. T., Washington, W. M., Fu, Q., Sikka, D. R., and Wild, M.: Atmospheric brown clouds: Impacts on South Asia climate and hydrological cycle, P. Natl. Acas. Sci. USA, 102, 5326–5333, 2005.
Rinaldi, M., Decesari, S., Carbone, C., Finessi, E., Fuzzi, S., Ceburnis, D., O'Dowd, C. D., Sciare, J., Burrows, J. P., and Vrekoussis, M.: Evidence of a natural marine source of oxalic acid and a possible link to glyoxal, J. Geophys. Res.-Atmos., 116, D16204, https://doi.org/10.1029/2011JD015659, 2011.
Rogge, W. F., Mazurek, M. A., Hildemann, L. M., Cass, G. R., and Simoneit,B. R.: Quantification of urban organic aerosols at a molecular level: identification, abundance and seasonal variation, Atmos. Environ. A, 27, 1309–1330, 1993.
Schauer, J. J., Kleeman, M. J., Cass, G. R., and Simoneit, B. R.: Measurement of emissions from air pollution sources. 3. C1–C29 organic compounds from fireplace combustion of wood, Environ. Sci. Technol., 35, 1716–1728, 2001.
Schulz, M., Prospero, J. M., Baker, A. R., Dentener, F., Ickes, L., Liss, P. S., Mahowald, N. M., Nickovic, S., García-Pando, C. P., and Rodríguez, S.: Atmospheric Transportand Deposition of Mineral Dustto the Ocean: Implications for Research Needs, Environ. Sci. Technol., 46, 10390–10404, 2012.
Sempéré, R. and Kawamura, K.: Comparative distributions of dicarboxylic acids and related polar compounds in snow, rain and aerosols from urban atmosphere, Atmos. Environ., 28, 449–459, 1994.
Sempéré, R. and Kawamura, K.: Trans-hemispheric contribution of C2–C10 α, ω-dicarboxylic acids, and related polar compounds to water-soluble organic carbon in the western Pacific aerosols in relation to photochemical oxidation reactions, Global Biogeochem. Cy., 17, 1069, https://doi.org/10.1029/2002gb001980, 2003.
Shaw, S. L., Gantt, B., and Meskhidze, N.: Production and emissions of marine isoprene and monoterpenes: a review, Adv. Meteorol., 2010, 408696, https://doi.org/10.1155/2010/408696, 2010.
Simoneit, B. R., Kobayashi, M., Mochida, M., Kawamura, K., Lee, M., Lim, H. J., Turpin, B. J., and Komazaki, Y.: Composition and major sources of organic compounds of aerosol particulate matter sampled during the ACE-Asia campaign, J. Geophys. Res.-Atmos., 109, D19S10, https://doi.org/10.1029/2004JD004598, 2004.
Song, J., Zhao, Y., Zhang, Y., Fu, P., Zheng, L., Yuan, Q., Wang, S., Huang, X., Xu, W., Cao, Z., Gromov, S., and Lai, S.: Influence of biomass burning on atmospheric aerosols over the western South China Sea: Insights from ions, carbonaceous fractions and stable carbon isotope ratios, Environ. Pollut., 242, 1800–1809, https://doi.org/10.1016/j.envpol.2018.07.088, 2018.
Sorooshian, A., Varutbangkul, V., Brechtel, F. J., Ervens, B., Feingold, G., Bahreini, R., Murphy, S. M., Holloway, J. S., Atlas, E. L., and Buzorius, G.: Oxalic acid in clear and cloudy atmospheres: Analysis of data from International Consortium for Atmospheric Research on Transport and Transformation 2004, J. Geophys. Res.-Atmos., 111, D23S45, https://doi.org/10.1029/2005JD006880, 2006.
Sorooshian, A., Lu, M.-L., Brechtel, F. J., Jonsson, H., Feingold, G., Flagan, R. C., and Seinfeld, J. H.: On the source of organic acid aerosol layers above clouds, Environ. Sci. Technol., 41, 4647–4654, 2007.
Sorooshian, A., Padró, L. T., Nenes, A., Feingold, G., McComiskey, A., Hersey, S. P., Gates, H., Jonsson, H. H., Miller, S. D., and Stephens, G. L.: On the link between ocean biota emissions, aerosol, and maritime clouds: Airborne, ground, and satellite measurements off the coast of California, Global Biogeochem. Cy., 23, GB4007, https://doi.org/10.1029/2009GB003464, 2009.
Sorooshian, A., Murphy, S. M., Hersey, S., Bahreini, R., Jonsson, H., Flagan, R. C., and Seinfeld, J. H.: Constraining the contribution of organic acids and AMS m∕z44 to the organic aerosol budget: On the importance of meteorology, aerosol hygroscopicity, and region, Geophys. Res. Lett., 37, L21807, https://doi.org/10.1029/2010gl044951, 2010.
Tan, S. C., Shi, G. Y., Shi, J. H., Gao, H. W., and Yao, X. H.: Correlation of Asian dust with chlorophyll and primary productivity in the coastal seas of China during the period from 1998 to 2008, J. Geophys. Res.-Biogeo., 116, G02029, https://doi.org/10.1029/2010jg001456, 2011.
Turpin, B. J. and Huntzicker, J. J.: Identification of secondary organic aerosol episodes and quantitation of primary and secondary organic aerosol concentrations during SCAQS, Atmos. Environ., 29, 3527–3544, 1995.
Vergara-Temprado, J., Murray, B. J., Wilson, T. W., O'Sullivan, D., Browse, J., Pringle, K. J., Ardon-Dryer, K., Bertram, A. K., Burrows, S. M., Ceburnis, D., DeMott, P. J., Mason, R. H., O'Dowd, C. D., Rinaldi, M., and Carslaw, K. S.: Contribution of feldspar and marine organic aerosols to global ice nucleating particle concentrations, Atmos. Chem. Phys., 17, 3637–3658, https://doi.org/10.5194/acp-17-3637-2017, 2017.
Volkamer, R., San Martini, F., Molina, L. T., Salcedo, D., Jimenez, J. L., and Molina, M. J.: A missing sink for gas-phase glyoxal in Mexico City: Formation of secondary organic aerosol, Geophys. Res. Lett., 34, L19807, https://doi.org/10.1029/2007GL030752, 2007.
Wang, G., Kawamura, K., Cheng, C., Li, J., Cao, J., Zhang, R., Zhang, T., Liu, S., and Zhao, Z.: Molecular distribution and stable carbon isotopic composition of dicarboxylic acids, ketocarboxylic acids, and alpha-dicarbonyls in size-resolved atmospheric particles from Xi'an City, China, Environ. Sci. Technol., 46, 4783–4791, https://doi.org/10.1021/es204322c, 2012.
Wang, G., Cheng, C., Meng, J., Huang, Y., Li, J., and Ren, Y.: Field observation on secondary organic aerosols during Asian dust storm periods: Formation mechanism of oxalic acid and related compounds on dust surface, Atmos. Environ., 113, 169–176, https://doi.org/10.1016/j.atmosenv.2015.05.013, 2015.
Wang, H. and Kawamura, K.: Stable carbon isotopic composition of low-molecular-weight dicarboxylic acids and ketoacids in remote marine aerosols, J. Geophys. Res.-Atmos., 111, D07304, https://doi.org/10.1029/2005JD006466, 2006.
Wang, H., Kawamura, K., and Yamazaki, K.: Water-soluble dicarboxylic acids, ketoacids and dicarbonyls in the atmospheric aerosols over the Southern Ocean and western Pacific Ocean, J. Atmos. Chem., 53, 43–61, 2006.
Warneck, P.: In-cloud chemistry opens pathway to the formation of oxalic acid in the marine atmosphere, Atmos. Environ., 37, 2423–2427, 2003.
Yang, L., Ray, M. B., and Liya, E. Y.: Photooxidation of dicarboxylic acids – Part II: Kinetics, intermediates and field observations, Atmos. Environ., 42, 868–880, 2008.
Yao, T., Wu, F., Ding, L., Sun, J., Zhu, L., Piao, S., Deng, T., Ni, X., Zheng, H., and Ouyang, H.: Multispherical interactions and their effects on the Tibetan Plateau's earth system: a review of the recent researches, Nat. Sci. Rev., 2, 468–488, 2015.
Zhang, M., Chen, J., Wang, T., Cheng, T., Lin, L., Bhatia, R., and Hanvey, M.: Chemical characterization of aerosols over the Atlantic Ocean and the Pacific Ocean during two cruises in 2007 and 2008, J. Geophys. Res.-Atmos., 115, D22302, https://doi.org/10.1029/2010JD014246, 2010.
Zhao, D. F., Buchholz, A., Kortner, B., Schlag, P., Rubach, F., Fuchs, H., Kiendler-Scharr, A., Tillmann, R., Wahner, A., Watne, Å. K., Hallquist, M., Flores, J. M., Rudich, Y., Kristensen, K., Hansen, A. M. K., Glasius, M., Kourtchev, I., Kalberer, M., and Mentel, Th. F.: Cloud condensation nuclei activity, droplet growth kinetics, and hygroscopicity of biogenic and anthropogenic secondary organic aerosol (SOA), Atmos. Chem. Phys., 16, 1105–1121, https://doi.org/10.5194/acp-16-1105-2016, 2016.
Zhao, W. Y., Kawamura, K., Yue, S. Y., Wei, L. F., Ren, H., Yan, Y., Kang, M. J., Li, L. J., Ren, L. J., Lai, S. C., Li, J., Sun, Y. L., Wang, Z. F., and Fu, P. Q.: Molecular distribution and compound-specific stable carbon isotopic composition of dicarboxylic acids, oxocarboxylic acids and alpha-dicarbonyls in PM2.5 from Beijing, China, Atmos. Chem. Phys., 18, 2749–2767, https://doi.org/10.5194/acp-18-2749-2018, 2018.
Zhao, Y., Zhang, Y. Y., Fu, P. Q., Ho, S. S. H., Ho, K. F., Liu, F. B., Zou, S. C., Wang, S., and Lai, S. C.: Non-polar organic compounds in marine aerosols over the northern South China Sea: Influence of continental outflow, Chemosphere, 153, 332–339, https://doi.org/10.1016/j.chemosphere.2016.03.069, 2016.
Zhuang, G. S., Yi, Z., Duce, R. A., and Brown, P. R.: Link between Iron and Sulfur Cycles Suggested by Detection of Fe(II) in Remote Marine Aerosols, Nature, 355, 537–539, 1992.