the Creative Commons Attribution 4.0 License.
the Creative Commons Attribution 4.0 License.
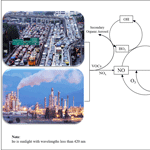
Assessment of gaseous criteria pollutants in the Bangkok Metropolitan Region, Thailand
Viney P. Aneja
Adel F. Hanna
The analysis of gaseous criteria pollutants in the Bangkok Metropolitan Region (BMR), Thailand, from 2010 to 2014 reveals that while the hourly concentrations of CO, SO2 and NO2 were mostly within the National Ambient Air Quality Standards (NAAQs) of Thailand, the hourly concentrations of O3 frequently exceeded the standard. The results reveal that the problem of high O3 concentration continuously persisted in this area. The O3 photolytic rate constant (j1) for BMR calculated based on assuming a photostationary state ranged from 0.008 to 0.013 s−1, which is similar to the calculated j1 using the NCAR TUV model (0.021±0.0024 s−1). Interconversion between O3, NO and NO2 indicates that crossover points between the species occur when the concentration of NOx (= NO + NO2) is ∼60 ppb. Under a low-NOx regime ([NOx] < 60 ppb), O3 is the dominant species, while, under a high-NOx regime ([NOx] > 60 ppb), NO dominates. Linear regression analysis between the concentrations of Ox (= O3 + NO2) and NOx provides the role of local and regional contributions to Ox. During O3 episodes ([O3]hourly > 100 ppb), the values of the local and regional contributions were nearly double of those during non-episodes. Ratio analysis suggests that the major contributors of primary pollutants over BMR are mobile sources. The air quality index (AQI) for BMR was predominantly good to moderate; however, unhealthy O3 categories were observed during episode conditions in the region.
- Article
(4027 KB) - Full-text XML
-
Supplement
(972 KB) - BibTeX
- EndNote
Over the last 3 decades, Thailand has experienced rapid industrialization, urbanization and economic growth (World Bank, 2018a). A majority of the country's development has occurred within and around Bangkok (BKK) (13.7∘ N, 100.5∘ E), the capital city of Thailand, and in the Bangkok Metropolitan Region (BMR). BMR is comprised of BKK and the five adjacent provinces of BKK (World Bank, 2018a, b). The increase in emissions is due to accelerated growth in automotive and industrial activities. As a major metropolitan area, BMR is dominated by mobile emissions sources, which contributes to the emissions of CO and NOx, precursors of ozone (O3) formation. The emissions from industrial activities also contribute to those emissions and to the emissions of sulfur dioxide (SO2) and the formation of particulate matter. Since 1995, BMR has begun to experience air quality degradation and experienced exceedances in Thailand National Ambient Air Quality Standards (NAAQs) for particulate matter (PM) and ozone (O3) (PCD, 2015) owing to strong solar radiation (peak density of direct radiation ∼1350 kWh m−2 yr−1), high temperature (yearly average ∼29 ∘C) and high humidity (yearly average ∼64 %) (Kumar et al., 2012).
The relationship between air pollution and public health in BMR has been examined in several published studies. Ruchirawat et al. (2007) reported that children who lived in BKK were exposed to high levels of carcinogenic air pollutants which might cause an elevated cancer risk. Buadong et al. (2009) reported that the exposure to elevated PM and O3 in elderly patients (≥65 years) was associated with an increasing in the number of hospital visits for cardiovascular diseases on the following day. Jinsart et al. (2002, 2012) reported the police personnel and drivers in BKK tended to be exposed to higher levels of PM concentrations compared with the general environment.
Several studies have demonstrated the role of atmospheric processes in elevating Thailand's O3. Long-range transport from the Asian continent has enhanced O3 concentrations in Thailand compared to the lesser O3 concentrations disbursed via long-range transports from the Indian Ocean (Pochanart et al., 2001). This regional transport, moreover, played an important role in seasonal fluctuations of O3 in this area (Zhang and Oahn, 2002). Another factor that enhanced O3 concentrations was the atmospheric chemistry of volatile organic compounds (VOCs). However, this process tended to be more important in enhancing O3 concentrations in suburban areas than in urban areas (Suthawaree et al., 2012).
Therefore, the availability and analysis of multiyear measurements of such gaseous criteria pollutants in the BMR will improve our understanding of how they contribute to the air quality of this area. In this study, we analyzed diurnal variations, seasonal variations and interannual trends of gaseous pollutants including carbon monoxide (CO), nitric oxide (NO), nitrogen dioxide (NO2), SO2 and O3 in BMR from 2010 to 2014. Chemical and physical processes associated with high O3 concentrations have been investigated. Since the monitoring station mostly measured concentrations of nitrogen oxide (NOx), O3 precursors in this study are referred to as NOx. The photochemical reaction for O3 was investigated during the photostationary state. The effects of local emission and regional contributions of Ox are presented. The severity of air pollution concentrations in BMR in relation to human health is assessed by using the air quality index (AQI).
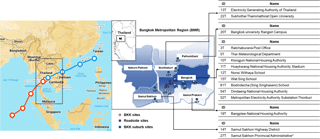
Figure 1Map of BMR, the location of monitoring stations and two major monsoons winds (from NOAA HYSPLIT back trajectory model). Three monitoring station types (BKK sites, roadside sites and BKK suburb sites) are shown as light blue dots, purple dots and blue dots, respectively. (Note: * the station has been closed since 1 October 2013.)
2.1 Study area
Figure 1 shows a map of BMR, the location of monitoring stations in this study and major monsoon winds over this region. BMR refers to BKK and the five adjacent provinces, i.e., Nakhon Pathom, Pathum Thani, Nonthaburi, Samut Prakan and Samut Sakhon. These provinces are linked to BKK in terms of traffic and industrial development (Zhang and Oanh, 2002). Thailand has three official seasons – local summer (February to May), rainy seasons (May to October) and local winter (October to February) as per the Thai Meteorological Department (TMD) (TMD, 2015). During the rainy season, this region's weather is influenced by southwest monsoon wind that travels from the Indian Ocean to Thailand. This marine air mass contains a large amount of moisture, resulting in the wet season in Thailand. During this season, Thailand is characterized by cloudy weather with high precipitation and high humidity. From October to April, this region is influenced by northeast monsoon wind that travels from the northeastern and the northern parts of Asia (China and Mongolia). This monsoon wind brings a cold and dry air mass, which leads to the dry season (local summer and local winter) in Thailand. The local winter in Thailand is characterized by cool and dry weather, while the local summer is characterized by hot (35 to 40∘) to extremely hot weather (> 40∘) due to strong solar radiation. During the dry season, storms may occur during the seasonal transition (TMD, 2015).
Transportation and industrial sectors are considered to be the major sources of air pollutants in the study area (Watcharavitoon et al., 2013). In 2014, ∼36 million new vehicles were registered in Thailand, and 29 % of these cars were registered in BKK (DLT, 2015). About 56 % and 28 % of the registered vehicles in BKK were gasoline and diesel engines, respectively. The remaining 16 % were run on compressed natural gas (CNG) (DLT, 2017). There are a variety of metal, auto parts, paper, plastic, food and chemical manufacturing facilities and power plants in the outskirts of BKK (DIW, 2016a, b, c, d, e).
2.2 Data collection and data analysis
Over the 5-year period (1 January 2010 to 31 December 2014), hourly observations from 15 Pollution Control Department (PCD) monitoring stations were analyzed. The monitoring stations are assigned to three categories: BKK sites, roadside sites and BKK suburb sites. BKK sites refer to the monitoring stations that are located within BKK's residential, commercial, industrial and mixed areas. They are within ∼50 to 100 m of the road. Roadside sites refer to the monitoring stations that are located in BKK within 2 to 5 m of the road (Zhang and Oanh, 2002). BKK suburb sites refer to the monitoring stations that are located in the provinces adjacent to BKK (Fig. 1). Quality assurance and quality control on the data set were performed by PCD prior to receiving the data. Hourly observations of the gaseous pollutants and meteorological parameters were automatically collected with autocalibration at the monitoring stations. Manual quality control was performed when unusual observations were found. An external audit of the equipment and monitoring stations was done every year. The data availability and details of equipment calibrations are provided in Fig. S1, Sect. S1, Supplement.
Gaseous species were measured at 3 m above ground level (a.g.l.). CO was measured using nondispersive infrared detection (Thermo Scientific 48i). NO and NO2 were measured using chemiluminescence detection (Thermo Scientific 42i). SO2 was measured using ultraviolet (UV) fluorescence detection (Thermo Scientific 43i), and O3 is measured by using UV absorption photometry detection (Thermo Scientific 49i). The meteorological parameters including wind speed (WS) and wind direction (WD) were measured at 10 m a.g.l. by a cup anemometer and potentiometer wind vanes. Temperature (T) and relative humidity (RH) were measured at 2 m a.g.l. by a thermistor and thin film capacitor, respectively (Watchravitoon et al., 2013). All the meteorological measurements were made by Met One or an equivalent method.
Data analysis, statistical analysis and plots were developed using Excel 2016. Predominant wind directions related to O3 concentrations are obtained using the Openair package (tool for the analysis of air pollution data) on the RStudio program (https://www.rstudio.com/, last access: 6 February 2018).
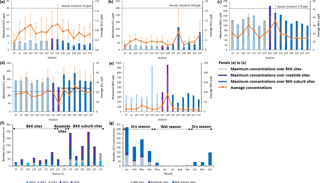
Figure 2Maximum (vertical bars) and average (solid line) concentrations of (a) CO, (b) SO2, (c) NO2 (d) O3 and (e) NO from the 15 monitoring stations, from 2010 to 2014, are compared with the hourly NAAQs (dotted line) of Thailand (except NO, which is not a criteria pollutant). The number of hourly O3 exceedances is shown by (f) locations and (g) seasons.
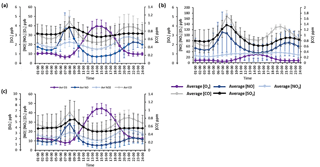
Figure 3Diurnal variations in gaseous species. The plots provide the average concentrations of O3, NO and NO2 in ppb, the average concentrations of CO in ppm, and the average concentrations of SO2 in ppb at (a) BKK sites, (b) roadside sites and (c) BKK suburb sites. Vertical bars provide ±1 standard deviation of the species concentrations.
3.1 Status of pollution in BMR from 2010 to 2014
Figure 2a to e show the maximum and average concentrations of gaseous pollutants, from 2010 to 2014 from the 15 monitoring stations. These concentrations are compared with the hourly NAAQs of Thailand (NAAQs of Thailand for hourly CO, NO2, SO2 and O3 are 30 ppm, 170 ppb, 300 ppb and 100 ppb, respectively (PCD, 2018)). Since NO is not a criteria pollutant, only the maximum and average concentrations are presented. During the study period, the maximum concentrations of CO, NO2 and SO2 were mostly at their hourly standards (an exceedance of NO2 was found at monitoring station 52T during 2013). However, the maximum concentrations of O3 exceeded its standard. Elevated CO, NO and NO2 concentrations were observed more frequently at roadside sites than other sites. The average concentrations of CO, NO and NO2 at roadside sites were ppm, ppb, and ppb, respectively. Elevated SO2 was more commonly observed at BKK suburb sites than other sites. The average concentrations of SO2 at BKK suburb sites were ppb. The average concentrations of O3 during the daytime (06:00 to 18:00 LT) over BKK sites, roadside sites and BKK suburb sites were , and ppb, and their values during the nighttime (18:00 to 6:00 LT) were , and ppb, respectively. The 24 h average O3 concentrations were highest at BKK suburb sites ( ppb), followed by BKK sites (18.6±2.3 ppb) and roadside sites (13.9±8.6 ppb). Statistical analyses of the concentrations of gaseous pollutants from the three monitoring station types are provided in Table S1, Sect. B, Supplement.
The seasonal variations in the gaseous pollutants reveal that, in general, elevated concentrations were observed during dry seasons and they decreased during wet seasons (Fig. S2, Sect. S3). Interannual variations in the gaseous pollutants reveal that while the concentrations of CO, NO2 and SO2 decreased or remained constant, the concentration of O3 tended to increase during the study period (Fig. S3, Sect. S4).
An O3 exceedance was recorded when an hourly concentration of O3 was greater than 100 ppb (hourly O3 standard). Figure 2f and g illustrate the number of hourly O3 exceedances, which are shown by location and by seasons. The hourly O3 exceedances at BKK suburb sites were more frequently observed than at the other sites. The average number of hourly O3 exceedances was ∼16 h yr−1 at BKK sites, ∼9 h yr−1 at roadside sites and ∼43 h yr−1 at BKK suburb sites. The hourly O3 exceedances were commonly observed during the dry season, less so during the transitional period between the seasons (May) and rarely during the wet season.
3.2 Diurnal variation in the gaseous species
Diurnal variations in gaseous pollutant are shown in Fig. 3a to c. The diurnal variations in O3 show a single-peak pattern (Aneja et al., 2001) with the concentrations increasing after sunrise and reaching the peak ∼ 15:00 local time (LT). The concentrations begin to decline in the evening and reach the minimum concentrations ∼ 07:00 LT the next morning. The concentrations of O3 at the peaks were ∼40 ppb at BKK sites, ∼30 ppb at roadside sites and ∼45 ppb at BKK suburb sites. The diurnal variations in NO show a bimodal pattern with the concentrations reaching the first and the second peak ∼ 07:00 to 09:00 and ∼ 21:00 to 22:00 LT, respectively. The concentrations of NO at the first and the second peak were ∼40 and ∼23 ppb at BKK sites, ∼110 and ∼73 ppb at roadside sites, and ∼30 and ∼13 ppb at BKK suburb sites. The concentrations of NO2 at the first and the second peak were ∼23 and ∼28 ppb at BKK sites, ∼33 and ∼37 ppb at roadside sites, and ∼20 and ∼22 ppb at BKK suburb sites. Even the diurnal variations in NOx show a bimodal pattern; at roadside sites the pattern was flatter than at other sites. The flatter pattern of NOx at roadside sites reveals that this monitoring station type was affected by a high concentration of NOx all day. The diurnal variations in CO show a bimodal pattern with the first and the second peak occurring ∼ 08:00 and 21:00 LT, respectively. The concentrations of CO at the first and the second peak were ∼1 ppm (both peaks) at BKK sites, ∼2 and ∼1.5 ppm at roadside sites, and ∼1 ppm (both peaks) at BKK suburb sites. The first peak of the diurnal variations in NO, NO2 and CO corresponds with the morning rush hour in BKK (07:00 to 09:00 LT). The second peak occurred ∼3 to 5 h after the evening traffic rush hour (16:00 to 18:00 LT) (Leong et al., 2002), due to a combination of pollutant emissions and the collapse of the planetary boundary layer (weak turbulence and diffusion) during this time. The diurnal variations in SO2 show a bimodal pattern with the first and the second peak of SO2 occurring ∼ 08:00 and 21:00 LT, respectively. The concentrations of SO2 at the first and the second peak were ∼4 and ∼3 ppb at BKK sites and roadside sites and ∼6 and ∼3 ppb at BKK suburb sites. At the roadside sites, the peaks are more obvious than at the other sites. The result indicates that at this monitoring station type, SO2 is primarily influenced by emissions from vehicle exhaust using a high sulfur content fuel (Henschel et al., 2013). It is noteworthy that BKK has a large diesel engine fleet (an estimated 25 % of registered vehicles) (DLT, 2015). Diesel fuel contains ∼0.035 % wt sulfur (DOEB, 2017). The season-wise distributions of the diurnal variations are provided in Fig. S4, Sect. S5.
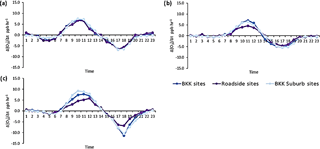
Figure 4Diurnal variations in the rate of change in O3 concentration (Δ[O3]/dt) during (a) local summers, (b) wet seasons and (c) local winters.
Figure 4a to c shows diurnal variations in the rate of change in O3 concentration (Δ[O3]/dt) during dry seasons (local summer and local winter) and wet seasons at the three monitoring station types (the data have been averaged for each monitoring station type to capture the rate of change in O3 concentration characteristics). The diurnal variations in Δ[O3]/dt are a combination of O3 chemistry and meteorology. In general, Δ[O3]/dt during the wet season were lower than those during dry season. However, during local winter, the rates of change in O3 concentration were the highest. The Δ[O3]/dt at the three monitoring station types, from 10:00 to 11:00 LT, were 4.5 to 7.0 ppb h−1 during wet seasons, 6.7 to 7.5 ppb h−1 during local summers and 5.7 to 9.2 ppb h−1 during local winters. The Δ[O3]/dt became negative from 14:00 to 15:00 LT. As expected, the rate of change in O3 concentration was nearly constant during the nighttime. Rapid changes in the mixing height and solar insolation during the morning increases Δ[O3]/dt. After sunset, the formation of O3 is inhibited and the planetary boundary layer becomes more stable resulting in O3 reduction through chemical reactions (for example, the oxidation of O3 by NOx) and physical processes (for example, dry deposition to the earth surface) (Naja and Lal, 2002).
3.3 Photochemical reaction and interconversion between O3, NO and NO2
The primary precursors for tropospheric O3, in the urban environment, are NOx and non-methane volatile organic compounds (VOCs), methane or CO (The Royal Society, 2008; Monks et al., 2009; Cooper et al., 2014). While NOx was measured continuously at all the monitoring sites, VOCs were measured periodically only at one monitoring station, limiting its usefulness as part of this study. In this study, the photostationary state (PSS) is applied through the chemical reactions of O3 formation from 10:00 to 16:00 LT. This time window is chosen due to the fully developed planetary boundary layer with well-mixed condition (Pochanart et al., 2001) to avoid the accumulation of air pollutants by surface inversion. Analysis and calculation are performed only during the dry season to eliminate the effects of the removal process by wet deposition.
The relationship among NO, NO2 and O3 under PSS is presented by Eq. (1) (Seinfeld and Pandis, 1998).
where [O3]PSS is the concentration of O3 at PSS and j1 and k3 are the reaction rate coefficient of the photochemical reaction of NO2 and the reaction rate coefficient of the chemical reaction between NO and O3, respectively.
The values for k3 (ppm−1 min−1) are calculated by Eq. (2) (Seinfeld and Pandis, 1998; Tiwari et al., 2015).
During dry seasons, the values of j1 ranged from 0.12 to 1.22 min−1, and the average of those at BKK sites, roadside sites and BKK suburb sites were 0.74±0.2, 0.64±0.3 and 0.55±0.3 min−1, respectively. The rate coefficients are calculated using the NCAR TUV model for 10:00 to 16:00 LT during the 2010 dry season at the latitude and longitude of 13.76∘ N and 100.50∘ E. The average j1 value calculated from the NCAR TUV model is 0.021±0.0024 s−1, which is similar to the calculated j1 values from Eq. (1) (j1 ranges from 0.008 to 0.013 s−1). The values of j1 from this study are similar to those values at an urban background site in Delhi, India (values of j1 ranged from 0.4 to 1.8 min−1 and the average was 0.8 min−1) (Tiwari et al., 2015) and those values collected during daytime in November in the UK (value of j1 was ∼0.14 min−1) (Clapp and Jenkin, 2001).
The values of k3, during dry seasons, ranged from 28.3 to 30.9 ppm−1 min−1, and the average of those at BKK sites, roadside sites and BKK suburb sites were 29.8±0.7, 29.7 and 29.8±0.7 ppm−1 min−1, respectively. The ratio of [NO2] and [NO] was ∼1.9. The statistical analysis of j1 (min−1 and s−1) and k3 (ppm−1 min−1 and cm3 molecule−1 s−1) at the three monitoring station types using Eq. (1) and the average j1 calculated from the NCAR TUV model are provided in Table S2, Sect. F.
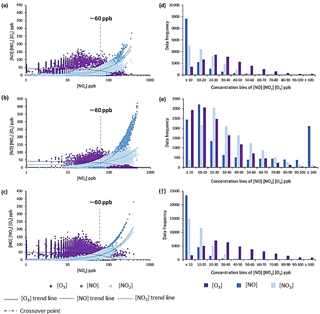
Figure 5Relationships and crossover points of NO, NO2 and O3 at (a) BKK sites, (b) roadside sites and (c) BKK suburb sites and concentration distributions of those species at (d) BKK sites, (e) roadside sites and (f) BKK suburb sites.
Figure 5a to c shows the relationships between NO, NO2 and O3, their crossover points, and concentration distributions. The crossover point among species occurs when the concentration of NOx is ∼60 ppb. At this point, two regimes are identified, including a low-NOx regime and a high-NOx regime. Under the low-NOx regime ([NOx] < 60 ppb), O3 is the dominant species and NO2 concentrations are higher than NO for NOx species. Conversely, under the high-NOx regime ([NOx]> 60 ppb), NO and NO2 increase and the concentrations of O3 rapidly decrease. Under the high-NOx regime, the decline in O3 trend lines may describe the O3 removal process through the titration of O3 by NO.
3.4 Local and regional contribution to Ox
The Ox concentration is the summation of O3 and NO2 concentration. Under the PSS condition, the concentration of NO, NO2 and O3 approaches an equilibrium and the concentration of Ox may be considered constant (Keuken et al., 2009). Since the conversion between O3 and NO2 in the urban and suburban atmosphere is rapid, the use of Ox to represent the production of oxidants is more appropriate than only using O3 (Lu et al, 2010). The local or NOx-dependent contribution refers to Ox concentration that are influenced by a concentration of the local pollutants. The regional or NOx-independent contribution refers to the background concentration of Ox that is not influenced by changes in the local pollutants (Clapp and Jenkin, 2001; Tiwari et al., 2015).
Table 1The comparison of fitted linear regression lines from this study, including at BKK sites, roadside sites and BKK suburb sites with fitted linear regression lines from other studies.
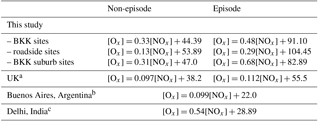
a Clapp and Jenkin (2001). b Mazzeo et al. (2005). c Tiwari et al. (2015).
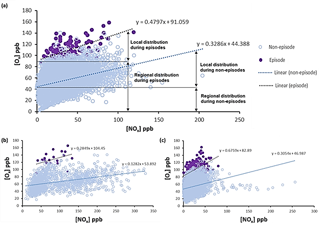
Figure 6The effects of local and regional contributions on Ox during non-episode and episode days at (a) BKK sites, (b) roadside sites and (c) BKK suburb sites.
Figure 6a to c show the local and regional contributions of Ox at the three monitoring station types. The effects of the local and regional contributions to Ox concentration are analyzed by plotting Ox concentrations against NOx concentrations and fitting the plot with a linear regression (). The concentrations of NOx and Ox are referred to as x and y, respectively. The slope of the linear regression (m) implies the local contribution, and the intercept with the y axis (c) implies the regional (background) contribution (Aneja et al., 2000; Clapp and Jerkin, 2001; Notario et al., 2012). Table 1 shows the comparison between fitted linear regressions from this study and fitted linear regression lines from other studies. The average background Ox concentrations over BMR during non-episodes ([O3]hourly < 100 ppb) and episodes ([O3]hourly > 100 ppb) were ∼48 and ∼95 ppb, respectively. The local and regional contributions during the episode days, in general, were about double of those during the non-episode days. The results reveal that elevated O3 concentrations during the episode days are influenced by both the local and regional contributions of Ox. It is noteworthy that the pattern of the local and regional contributions at roadside sites during non-episode periods is composed of two NOx concentration regimes. The low-NOx regime (NOx < 60 ppb) resembles the local and regional contributions during non-episodes over BKK suburb sites. The high-NOx regime (NOx > 60 ppb) may represent the typical characteristics of air quality near roads.
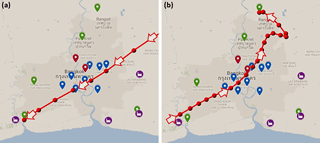
Figure 7Backward trajectories from the HYSPLIT model reveal a (a) NE wind direction (13 January 2010) and (b) SW wind direction (1 January 2010).
The local contributions from the fitted linear regressions are compared with the local contribution that is calculated from the delta O3 method. A delta O3 (ΔO3) analysis was performed to reflect on the intensity of O3 production in the BMR area (Lindsay and Chameides, 1988). Lindsay et al. (1989) analyzed high-O3 events in Atlanta, GA, USA, and showed that rural background O3 during high O3 concentrations ([O3] > 80 ppb) in the Atlanta metropolitan area was higher than its average and the concentration of O3 increased from ∼15 to 20 ppb when the air mass traveled across the city. This enhanced the total O3 concentration from 80 to 85 ppb. In our study, the differences in the concentrations of O3 at the upwind and downwind monitoring stations (monitoring stations 20T and 27T) are averaged. The conditions to calculate ΔO3 in this study are as follows (1) high O3 concentrations ([O3] > 80 ppb) were observed at at least one of the two monitoring stations; (2) the calculation is performed from 10:00 to 16:00 LT during the dry season to avoid the accumulation of air pollutants by surface inversion and the effects of the removal process by wet deposition; (3) National Oceanic and Atmospheric Administration (NOAA) HYSPLIT model backward trajectories revealed N–NE, S–SW wind directions (Fig. 7). Even the O3 concentrations at the downwind monitoring stations are expected to be greater than the O3 concentrations at the upwind monitoring stations, a negative ΔO3 may be found. The negative ΔO3 suggests the deposition of O3 and/or that O3 was consumed as it passes over the city and/or that there may have been a wind reversal so that air already polluted by the metropolitan area was brought back in to the city (Lindsay et al., 1989). The ΔO3 in BMR ranged from −53 to 86 ppb (average ∼10.4 ppb) and ranged from −66 to 96 ppb (average ∼9.4 ppb) when the predominant wind directions advecting into the city were from NE and SW, respectively. Thus, we find that there was a ∼10 ppb enhancement of the O3 concentration during the air pollution high O3 concentration in BMR ([O3] > 80 ppb), which corroborates local O3 production analysis based on linear regression.
3.5 Correlation of air pollutants
3.5.1 Local sources analysis
The characteristics of emission sources are often determined by the ratios between CO and NOx (CO ∕ NOx) and SO2 and NOx (SO2 ∕ NOx). In general, the major sources of NOx are point sources and mobile sources. However, NOx from point sources is more likely correlated with SO2. NOx from mobile sources is more likely correlated with CO (Parrish et al., 1991). Therefore, the characteristics of mobile sources are high CO ∕ NOx ratios and low SO2 ∕ NOx ratios. In contrast to mobile sources, the characteristics of point sources are low CO ∕ NOx ratios and high SO2 ∕ NOx ratios (Parrish et al., 1991; Rasheed et al., 2014).
Table 2The comparison of CO ∕ NOx and SO2 ∕ NOx ratios from this study with other studies (modified from Rasheed et al., 2014).
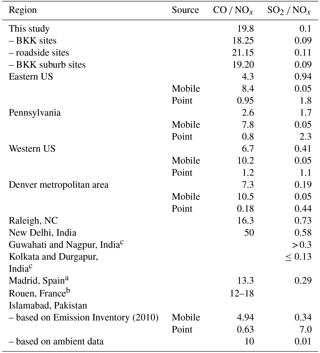
a Fernandez-Jiménez et al. (2003). b Coppalle et al. (2001). c Mallik and Lal (2014).
Table 2 shows the comparison between the CO ∕ NOx and SO2 ∕ NOx ratios from this study when compared with other studies. The ratio of CO ∕ NOx is 19.8, and the ratio of SO2 ∕ NOx is 0.1 over BMR. This suggests that the major contributors of primary pollutants over the BMR are mobile sources. However, this region may be influenced by manufacturing facilities' point sources (SO2 contributor) on the outskirts of the BKK. These point sources will impact the concentrations of SO2, NOx and CO. Correlations among species are provided in Table S3, Sect. G.
3.5.2 Effects of pollutant transport
In general, O3 has a short lifetime in the polluted urban atmosphere (approximately hours). However, O3 has a longer lifetime of several weeks in the free troposphere. This occurrence may allow O3 to be transported over continental scales (Stevenson et al., 2006: CO ∕ NOx; Young et al., 2013: CO ∕ NOx; Monks et al., 2015). Figure 8 shows O3 concentrations, during episodes and non-episodes, with predominant wind directions and wind speeds. The results show that O3 exceedances are associated with low wind speed and predominant wind directions, i.e., the origins of the air masses. In general, elevated O3 concentrations were observed with a wind speed lower than 4 ms−1 with northerly winds (station 22T), southerly winds (stations 3T, 10T, 19T, 20T and 61T) and westerly winds (station 52T). It is noteworthy that the southerly winds, generally, bring cleaner marine air mass to the land. However, under a stagnant condition (i.e., low wind speed), elevated O3 concentrations were observed during southerly winds (Sahu et al., 2013a, b).
3.6 Air quality index for O3 management
In the US, the AQI for air pollutants is divided into six categories (good, moderate, unhealthy for sensitive groups, unhealthy, very unhealthy and hazardous). These categories are nonlinear and relate to human health (U.S. EPA, 2017a, b, c). In Thailand, the NAAQs for the air pollutant species are pegged at an AQI value of 100. In this study, the severity of O3 concentrations in BMR is evaluated by AQI for O3. Table 3 provides the ambient air quality over BMR from 2010 to 2014 based on the AQI of O3. Based on the AQI for O3, during the study period, the majority of air quality over BMR was in the good AQI category (∼97 %) followed by the moderate air quality category (∼2.3 %). However, the unhealthy for sensitive groups (∼0.7 %), unhealthy (∼0.3 %) and very unhealthy (∼0.04 %) O3 air quality categories were observed. Generally, BKK suburb sites have a higher number of hours that were categorized as unhealthy for sensitive groups, unhealthy and very unhealthy than BKK and roadside sites. The average number of hours that were categorized as unhealthy for sensitive groups, unhealthy and very unhealthy over BKK suburb sites were 425.8, 146.7 and 28.7 h. The calculation of the AQI for O3 can be found in Figs. S5 and S6, Sect. S8.
This study provides measurements and analysis for the gaseous criteria pollutants. However, in order to provide a well-established air quality management policy, the integration of multidisciplinary analysis is needed. This will include scientific, socioeconomic and policy analysis (Aneja et al, 2001). The results from this study revealed evidence of O3 air quality standards being breached. This resulted in adverse health effects, human welfare, economics, and environment over BMR. Ratio analysis suggests that the first priority should be controlling pollution emissions from local sources that are primarily mobile. The complex relationship between O3 and its precursors and the effects of pollution transport show that decreasing only NOx emissions and/or local emissions may not be an effective policy to reduce O3 because of regional air pollution transport (i.e., ozone and its precursors contribute to O3 exceedances). To identify the proportional contribution between local and regional sources of O3 concentrations during selected O3 episode days, atmospheric modeling is needed to quantify various processes that contribute to the ambient concentration at specific locations. This scientific analysis provides a framework for the process of establishing an air quality policy while analyzing socioeconomic impacts.
Among measured gaseous criteria pollutants, O3 is the only species whose concentrations frequently exceed the NAAQs of Thailand. The O3 exceedances occur during the dry season (local summer and local winter) and more frequently occur over BKK sites and BKK suburb sites than roadside sites. On average, the number of hourly O3 exceedances at BKK sites, roadside sites and BKK suburb sites was ∼16, ∼9 and ∼43 h yr−1, respectively. The lower number of O3 exceedances at roadside sites demonstrates the effects of the titration of O3 by NO due to high concentrations of NO that were generally observed at this monitoring station type (average [NO] ppb). Under the photostationary state assumption, during the dry season, the values of the reaction rate coefficient of the photochemical reaction of NO2 (j1) and the reaction rate coefficient of the chemical reaction between NO and O3 (k3) from 0.12 to 1.22 min−1 and range from 28.3 to 30.9 ppm−1 min−1, respectively. NOx values of ∼60 ppb mark the threshold for the interconversion between O3, NO and NO2. Under the low-NOx regime ([NOx] < 60 ppb), O3 is the dominant species. On the other hand, under the high-NOx regime ([NOx] > 60 ppb), the concentrations of O3 rapidly decrease. The decrease in O3 under the high-NOx regime describes the important role of NO in destroying O3 in the atmosphere in polluted environments. The local and regional contributions of Ox concentrations under stagnant conditions (wind speed < 4 m s−1) and the origin of air masses containing O3 and its precursors are associated with elevated O3 concentrations in this area. During O3 episodes, the values of the local and regional contributions were about double those during non-episodes. The air quality index for O3 reveals evidence of air quality standards being breached in BMR, resulting in potentially adverse health effects. To achieve O3 reduction, control strategies may be needed. Emissions from mobile sources may be the first priority to manage O3, since BMR is more likely to be affected by mobile sources than point sources (CO ∕ NOx =19.8 and SO2 ∕ NOx =0.1). Due to the highly nonlinear physical and chemical processes governing the atmosphere, control strategies need to be evaluated in a more comprehensive approach. Air quality modeling of pollution episodes in the BMR would be an appropriate approach to accurately quantify various atmospheric processes contributing to high O3 concentrations in BMR.
The data may be obtained upon request from the Director of the Air Quality and Noise (AQNIS) Management Bureau, Pollution Control Department, Ministry of Natural Resources and Environment, Phahonyothin Rd, Samsen Nai, Phaya Thai, Bangkok, Thailand, 10400 (aqnis.web@gmail.com); tel: +66 2 298 2318; fax: +66 2 298 5389.
The supplement related to this article is available online at: https://doi.org/10.5194/acp-18-12581-2018-supplement.
PU is a PhD graduate student who developed the idea, analyzed the data, and performed the computations. VPA is a Professor, and AFH, Research Professor are advising the student. All the co-authors contributed in the development of the manuscript.
The authors declare that they have no conflict of interest.
We thank the Royal Thai Government for providing a fellowship to Pornpan Uttamang (ref. no.1018.2/4440).
We thank Surat Bualert, Dean of Faculty of Environment, Kasetsart University,
Bangkok, Thailand and Air Quality and Noise Management Bureau, Pollution
Control Department, Ministry of Natural Resources and Environment, Bangkok,
Thailand, for providing QA/QC air pollution and meteorology data. We also
thank Elizabeth Adams and Kurt Thurber for their assistance in the editorial
review of the paper. We also thank Dennis Mikel and one anonymous reviewer
for their constructive comments.
Edited by:
Manvendra K. Dubey
Reviewed by: Dennis Mikel and one anonymous
referee
Aneja, V. P., Agarwal, A., Roelle, P. A., Phillips, S. B., Tong, Q., Watkins, N., and Yablonsky, R.: Measurements and Analysis of Criteria Pollutants in New Delhi, India, Environ. Int., 27, 35–42, https://doi.org/10.1016/s0160-4120(01)00051-4, 2001.
Buadong, D., Jinsart, W., Funatagawa, I., Karita, K., and Yano, E.: Association Between PM10 and O3 Levels and Hospital Visits for Cardiovascular Diseases in Bangkok, Thailand, J. Epidemiol., 19, 182–188, https://doi.org/10.2188/jea.je20080047, 2009.
Clapp, L. J. and Jenkin, M. E.: Analysis of the relationship between ambient levels of O3, NO2 and NO as a function of NOx in the UK, Atmos. Environ., 35, 6391–6405, https://doi.org/10.1016/S1352-2310(01)00378-8, 2001.
Cooper, O. R., Parrish, D. D., Ziemke, J., Balashov, N. V., Cupeiro, M., Galbally, I. E., Gilge, S., Horowitz, L., Jensen, N. R., Lamarque, J. F., Naik, V., Oltmans, S. J., Schwab, J., Shindell, D. T., Thompson, A. M., Thouret, V., Wang, Y., and Zbinden, R. M.: Global distribution and trends of tropospheric ozone: An observation-based, Elementa, 2, 1–28, https://doi.org/10.12952/journal.elementa.000029, 2014.
Coppalle, A., Delmas, V., and Bobbia, M.: Variability of NOx and NO2 concentrations observed at pedestrian level in the city center of a medium size urban area, Atmos. Environ., 35, 5361–5369, 2001.
DIW: Manufacturing plant statistics, Nakhon Pathom, Thailand, available at: http://userdb.diw.go.th/results1.asp?pageno=1&provname, last access: December 2016a.
DIW: Manufacturing plant statistics, Pathum Thani, Thailand, available at: http://userdb.diw.go.th/results1.asp?pageno=1&provname, last access: December 2016b.
DIW: Manufacturing plant statistics, Nonthaburi, Thailand, available at: http://userdb.diw.go.th/results1.asp?pageno=1&provname=, last access: December 2016c.
DIW: Manufacturing plant statistics, Samut Prakan, Thailand, available at: http://userdb.diw.go.th/results1.asp?pageno=1&provname=, last access: December 2016d.
DIW: Manufacturing plant statistics, Samut Sakhon, Thailand, available at: http://userdb.diw.go.th/results1.asp?pageno=1&provname=, last access: December 2016e.
DLT: Transportation statistics, Department of land transport, Thailand, available at: http://apps.dlt.go.th/statistics_web/statistics.html, last access: December 2015.
DLT: Statistic of registered vehicle in Bangkok, categorized by fuel types, Department of land transport, Thailand, available at: http://apps.dlt.go.th/statistics_web/fuel.html, last access: June 2017.
DOEB: Notice of Department of Energy Business on determination of characteristic and quality of diesel fuel (volume 5), 2011, available at: http://elaw.doeb.go.th/document_doeb/319_0001.pdf, last access: June 2017.
Fernandez-Jiménez, M. T., Climent-Font, A., and Sánchez-Antón, J. L.: Long-term atmospheric pollution study at Madrid City (Spain), Water Air Soil Pollut., 142, 243–260, 2003.
Henschel, S., Querol, X., Atkinson, R., Pandolfi, M., Zeka, A., Tertre, A. L., Analistis, A., Katsouyanni, K., Chanel, O., Pascal, M., Bouland, C., Haluza, D., Medina, S., and Goodman, P. G.: Ambient air SO2 patterns in 6 European cities, Atmos. Environ.,79, 236–247, https://doi.org/10.1016/j.atmosenv.2013.06.008, 2013.
Jinsart, W., Tamura, K., Loetkamonwit, S., Thepanondh, S., Karita, K., and Yano, E.: Roadside Particulate Air Pollution in Bangkok, J. Air Waste Manage. Assoc., 52, 1102–1110, https://doi.org/10.1080/10473289.2002.10470845, 2002.
Jinsart, W., Kaewmanee, C., Inoue, M., Hara, K., Hasegawa, S., Karita, K., Tamura, K., and Yano, E.: Driver exposure to particulate matter in Bangkok, J. Air Waste Manage. Assoc., 62, 64–71, https://doi.org/10.1080/10473289.2011.622854, 2012.
Keuken, M., Roemer, M., and Elshout, S. V.: Trend analysis of urban NO2 concentrations and the importance of direct NO2 emissions versus ozone/NOx equilibrium, Atmos. Environ., 43, 4780–4783, https://doi.org/10.1016/j.atmosenv.2008.07.043, 2009.
Kumar, R., Naja, M., Pfister, G. G., Barth, M. C., Wiedinmyer, C., and Brasseur, G. P.: Simulations over South Asia using the Weather Research and Forecasting model with Chemistry (WRF-Chem): chemistry evaluation and initial results, Geosci. Model Dev., 5, 619–648, https://doi.org/10.5194/gmd-5-619-2012, 2012.
Leong, S. T., Muttamara, S., and Laortanakul, P.: Air Pollution and Traffic Measurements in Bangkok Streets, Asian J. Energy Environ, 3, 185–213, 2002.
Lindsay, R. W. and Chameides, W. L.: High-ozone events in Atlanta, Georgia, in 1983 and 1984, Environ. Sci. Technol., 22, 426–431, https://doi.org/10.1021/es00169a010, 1988.
Lindsay, R. W., Richardson, J. L., and Chameides, W. L.: Ozone trends in Atlanta, Georgia: Have emission controls been effective? JAPCA, 39, 40–43, 1989.
Lu, K., Zhang, Y., Su, H., Brauers, T., Chou, C. C., Hofzumahaus, A., Liu, S. C., Kita, K., Kondo, Y., Shao, M., Wahner, A., Wang, J., Wang, X., and Zhu, T.: Oxidant (O3+NO2) production processes and formation regimes in Beijing, J. Geophys. Res., 15, D07303, https://doi.org/10.1029/2009JD012714, 2010.
Mallik, C. and Lal, S.: Seasonal characteristics of SO2, NO2, and CO emissions in and around the Indo-Gangetic Plain, Environ. Monit. Assess., 186, 1295–1310, https://doi.org/10.1007/s10661-013-3458-y, 2014.
Mazzeo, N., Venegas, L., and Choren, H.: Analysis of NO, NO2, O3 and NOx concentrations measured at a green area of Buenos Aires City during wintertime, Atmos. Environ., 39, 3055–3068, https://doi.org/10.1016/j.atmosenv.2005.01.029, 2005.
Monks, P. S., Granier, C., Fuzzi, S., Stohl, A., Williams, M., et al.: Atmospheric Composition Change – Global and Regional Air Quality, Atmos. Environ, 43, 5268–5350, 2009.
Monks, P. S., Archibald, A. T., Colette, A., Cooper, O., Coyle, M., Derwent, R., Fowler, D., Granier, C., Law, K. S., Mills, G. E., Stevenson, D. S., Tarasova, O., Thouret, V., von Schneidemesser, E., Sommariva, R., Wild, O., and Williams, M. L.: Tropospheric ozone and its precursors from the urban to the global scale from air quality to short-lived climate forcer, Atmos. Chem. Phys., 15, 8889–8973, https://doi.org/10.5194/acp-15-8889-2015, 2015.
Naja, M. and S. Lal: Surface ozone and precursor gases at Gadanki (13.5∘ N, 79.2∘ E), a tropical rural site in India, J. Geophys. Res., 107, 4197, https://doi.org/10.1029/2001JD000357, 2002.
Notario, A., Bravo, I., Adame, J. A., Díaz-de-Mera, Y., Aranda, A., Rodríguez, A., and Rodríguez, D.: Analysis of NO, NO2, NOx, O3 and oxidant (Ox = O3+NO2) levels measured in a metropolitan area in the southwest Iberian Peninsula, Atmos. Res., 104–105, 217–226, https://doi.org/10.1016/j.atmosres.2011.10.008, 2012.
Parrish, D. D., Trainer, M., Buhr, M. P., Watkins, B. A., and Fehsenfeld, F. C.: Carbon monoxide concentrations and their relation to concentrations of total reactive oxidized nitrogen at two rural U.S. sites, J. Geophys. Res., 96, 9309–9320, https://doi.org/10.1029/91JD00047, 1991.
PCD: Thailand State of Environment 1998, Pollution Control Department, Thailand, available at: http://www.pcd.go.th/public/Publications/print_report.cfm? task=report2538, last access: November 2015.
PCD: National ambient air quality and Noise standards of Thailand, Pollution Control Department, Thailand, available at: http://www.pcd.go.th/info_serv/reg_std_airsnd01.html, last access: April 2018.
Pochanart, P., Kreasuwun, J., Sukasem, P., Geeratithadaniyom, W., Tabucanon, M. S., Hirokawa, J., Kajii, Y., and Akimoto, H.: Tropical tropospheric ozone observed in Thailand. Atmos. Environ., 35, 2657–2668. https://doi.org/10.1016/s1352-2310(00)00441-6, 2001.
Rasheed, A., Aneja, V. P., Aiyyer, A., and Rafique, U.: Measurements and analysis of air quality in Islamabad, Pakistan, Earth's Future, 2, 303–314, https://doi.org/10.1002/2013EF000174, 2014.
Ruchirawat, M., Settachan, D., Navasumrit, P., Tuntawiroon, J., and Autrup, H.: Assessment of potential cancer risk in children exposed to urban air pollution in Bangkok, Thailand, Toxicol. Lett., 168, 200–209, https://doi.org/10.1016/j.toxlet.2006.09.013, 2007.
Sahu, L. K., Sheel, V., Kajino, M., and Nedelec, P.: Variability in tropospheric carbon monoxide over an urban site in Southeast Asia, Atmos. Environ., 68, 243–255, https://doi.org/10.1016/j.atmosenv.2012.11.057, 2013a.
Sahu, L. K., Sheel, V., Kajino, M., Gunthe, S. S., Thouret, V., Nedelec, P., and Smit, H. G.: Characteristic of tropospheric ozone variability over an urban site in Southeast Asia: A study based on MOZAIC and MOZART vertical profiles, J. Geophys. Res.-Atmos., 118, 8729–8747, https://doi.org/10.1002/jgrd.50662, 2013b.
Seinfeld, J. H. and Pandis, S. N.: Atmospheric chemistry and physics: from air pollution to climate change, Wiley, New York, USA, 1998.
Suthawaree, J., Tajima, Y., Khunchorn, A., Kato, S., Sharp, A., and Kajii, Y.: Identification of volatile organic compounds in suburban Bangkok, Thailand and their potential for ozone formation, Atmos. Res., 104–105, 245–254, https://doi.org/10.1016/j.atmosres.2011.10.019, 2012.
The Royal Society.: Ground-level Ozone in the 21st century: Future Trends, Impacts and Policy Implications, Royal Society policy document 15/08, RS1276, https://royalsociety.org/~/media/Royal_Society_Content/policy/publications/2008/7925.pdf, 2008.
Tiwari, S., Dahiya, A., and Kumar, N.: Investigation into relationships among NO, NO2, NOx, O3, and CO at an urban background site in Delhi, India, Atmos. Res., 157, 119–126, https://doi.org/10.1016/j.atmosres.2015.01.008, 2015.
TMD: Climate of Thailand, available at: https://www.tmd.go.th/info/climate_of_thailand-2524-2553.pdf, last access: November 2015.
U.S. EPA: Air Quality Guide for Ozone, available at: https://airnow.gov/index.cfm?action=pubs.aqiguideozone, last access: April 2017a.
U.S. EPA: Air Quality Index (AQI) Basics, available at: https://airnow.gov/index.cfm?action=aqibasics.aqi, last access: April 2017b.
U.S. EPA: Daily and Hourly AQI – Ozone, available at: https://forum.airnowtech.org/t/daily-and-hourly-aqi-ozone/170, last access: April 2017c.
Watcharavitoon, P., Chio, C. P., and Chan, C. C.: Temporal and Spatial Variations in Ambient Air Quality during 1996–2009 in Bangkok, Thailand, Aerosol Air Qual. Res., 13, 1741–1754, https://doi.org/10.4209/aaqr.2012.11.0305, 2013.
World Bank: Industrial change in the Bangkok urban region, available at: https://openknowledge.worldbank.org/handle/10986/27380?locale-attribute=en, last access: April 2018a.
World Bank: Urbanization in Thailand is dominated by the Bangkok urban area, available at: http://www.worldbank.org/en/news/feature/2015/01/26/urbanization-in-thailand-is-dominated-by-the-bangkok-urban-area, last access: April 2018b.
Zhang, B. and Oanh, N. K.: Photochemical smog pollution in the Bangkok Metropolitan Region of Thailand in relation to O3 precursor concentrations and meteorological conditions, Atmos. Environ., 36, 4211–4222, https://doi.org/10.1016/S1352-2310(02)00348-5, 2002.