the Creative Commons Attribution 4.0 License.
the Creative Commons Attribution 4.0 License.
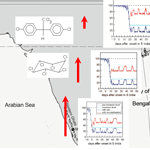
Revolatilisation of soil-accumulated pollutants triggered by the summer monsoon in India
Céline Degrendele
Sachin S. Gunthe
Qing Mu
Akila Muthalagu
Ondřej Audy
Chelackal V. Biju
Petr Kukučka
Marie D. Mulder
Mega Octaviani
Petra Příbylová
Pourya Shahpoury
Irene Stemmler
Aswathy E. Valsan
Persistent organic pollutants that have accumulated in soils can be remobilised by volatilisation in response to chemical equilibrium with the atmosphere. Clean air masses from the Indian Ocean, advected with the onset of the summer monsoon, are found to reduce concentrations of hexachlorocyclohexane (HCH), dichlorodiphenyltrichloroethane (DDT) and its derivatives, endosulfan and polychlorinated biphenyls (PCBs) in air at a mountain site (all in the range 5–20 pg m−3) by 77 %, 70 %, 82 % and 45 %, respectively. The analysis of fugacities in soil and air suggest that the arrival of summer monsoon triggers net volatilisation or enhances ongoing revolatilisation of the now-banned chemicals HCH and PCBs from background soils in southern India. The response of the air–soil exchange was modelled using a regional air pollution model, WRF-Chem PAH/POP. The results suggest that the air is increasingly polluted during transport by the south-westerly monsoon winds across the subcontinent. Using a multidecadal multimedia mass balance model, it is found that air–surface exchange of HCH and DDT have declined since the ban of these substances from agriculture, but remobilisation of higher chlorinated PCBs may have reached a historical high, 40 years after peak emission.
- Article
(2950 KB) -
Supplement
(1021 KB) - BibTeX
- EndNote
Persistent organic pollutants pose a hazard to humans and wildlife as they may reach harmful concentrations in biota upon accumulation along food chains. Semi-volatile substances (i.e. vapour pressure at 293 K in the range 10−6–10−2 Pa) diffuse across air–sea and air–land interfaces in both directions. They tend to net volatilise from land and sea surfaces on which they had previously been deposited once a level of contamination in chemical equilibrium with air pollution was reached (Bidleman, 1999; Cousins et al., 1999; Meijer et al., 2003; Kurt-Karakus et al., 2006; Wong et al., 2007; Růzičková et al., 2008; Degrendele et al., 2016). The potential to revolatilise is relevant to assessing risks from chemicals as it enhances the long-range transport potential and hence facilitates transport to and accumulation in remote areas, which are pristine with regard to primary (direct) contamination (Wania and Mackay, 1993; Semeena and Lammel, 2005; Wania and Westgate, 2008; Lammel and Stemmler, 2012). In the terrestrial environment, soils represent the main reservoir of the more lipophilic substances (log Koa≳6), while smaller mass fractions are stored in the atmosphere, vegetation and freshwater sources as suggested by field studies (Meijer et al., 2003) and modelling (Wania, 2006; Lammel et al., 2007; Lammel and Stemmler, 2012). Thus, understanding the dynamics of soil contamination and exchange with the overlying air is important for assessing spatio-temporal scales of the distribution and impact of local pollution. Air–soil dynamics occurs on various timescales, from multi-year long-term trends (Lammel and Stemmler, 2012) to seasonal cycling and short-term fluctuations (Bidleman, 1999). One key region, where persistent organic pollutants have been heavily used, is southern Asia. In India, high levels of organochlorine pesticides (OCPs) were found in both abiotic (Ramesh et al., 1989, 1991; Kumari et al., 1996; Rajendran et al., 1999; UNEP, 2002; Shunthirasingham et al., 2010; Pozo et al., 2011, Chakraborty and Zhang, 2012; Bajwa et al., 2016) and biotic (Ramesh et al., 1990, 1992; Senthilkumar et al., 2001; UNEP, 2002) environmental samples. The country is considered a hotspot for DDT and hexachlorocyclohexane (HCH) with no evidence of decline (Sharma et al., 2014). Besides OCPs, polychlorinated biphenyls (PCBs) and polybrominated diphenylethers (PBDEs) are of relevance in southern Asia, where they were used as flame retardants. High levels of PBDEs were reported in India (Zhang et al., 2008) and waste might be a significant ongoing source of penta- and hexachlorobenzene (PeCB, HCB), PCBs (Senthilkumar et al., 2001; Wong et al., 2010; Zhang et al., 2011; Sharma et al., 2014) and PBDEs (Breivik et al., 2012; Sharma et al., 2014). Thus far, studies on the environmental exposure of the Indian subcontinent have been mostly limited to urban areas (Chakraborty and Zhang, 2012; Sharma et al., 2014; Chakraborty et al., 2015), while the continental background was scarcely addressed. The air–soil dynamics of OCPs or other semi-volatile substances related to the monsoon have not been studied yet. In India, air pollution levels are expected to drop with the onset of the summer monsoon. Triggered by the seasonal shift of the intertropical convergence zone, the large-scale advection pattern switches from regional (southern Asia and adjacent seas) to intercontinental (from the Indian Ocean with influence from the relatively clean Southern Hemisphere (IMD, 2014).
Here we study air and soil pollution in India, first time with focus on the impact of the summer monsoon on air–surface exchange. The hypothesis is tested, whether drop of concentrations in air at the onset of the summer monsoon mobilises pollutants stored in soils. To this end, (1) field observations in background soils in the Western Ghats, the first highlands that the south-westerly monsoon winds encounter, were performed before and during the onset of the monsoon (May–June 2014). These were complemented by (2) regional-scale chemistry-transport modelling of the monsoon onset on the Indian subcontinent using a 3-D air pollution model coupled to a soil compartment (3-D model WRF-Chem PAH/POP). Finally, (3) the long-term chemodynamics is assessed by multimedia mass balance modelling, forced by climate and 3-D modelling data (1-D model, series of two boxes).
2.1 Sites and sampling
Air samples were collected from 5 May to 10 June 2014, 90 km inland from the Arabian Sea coast, on a slope oriented south-west in the northern outskirts of the town Munnar (10.093∘ N,77.068∘ E; Fig. 4) at 1600 m a.s.l., with the mountain ridge's elevation in the area ranging from 1950 to 2450 m a.s.l. The site is reached freely, i.e. without topographic obstacles, by air masses that are advected through the sector 180–360∘ N. It is directly adjacent to tea plantations (south to west) and deciduous forest (north-west to north-east). Additional land cover includes shrubs (south, east) and, to a lesser extent, agricultural fields and residential areas (south to south-east). Twenty-four air samples were collected. The 2014 monsoon season in the area was characterised by scattered rainfall at the monsoon onset, after which rainfall became persistent from the last week of June (Valsan et al., 2016).
For air sampling a high volume sampler (Digitel DH-77) equipped with a quartz-fibre filter and two polyurethane foam (PUF) plugs (Gumotex Břeclav, density 0.030 g cm−3, 100 mm diameter, total depth 12 cm, cleaned by extraction in acetone and dichloromethane) was used. Soil samples were taken from one plot each in the tea plantation, in shrubs and in forest, at distances within 1 km from each other. The uppermost 5 cm of soil was collected (using a spade, Edelman auger and sieve). Each soil sample is a composite (pooled sample), produced from equal amounts of soil collected from six individual spots at distances of 1 m from each other. Three replicates of each composite sample were analysed. At all plots the samples were nitisol (GOI, 1985; FAO, 2014), horizon A, which had a brownish, loose, single grain structure, with fine roots in the shrubs and forest. Soil samples were homogenised by sieving and mixing. PUF samples were spiked to control analyte losses during handling, shipping and storage.
2.2 Chemical and data analysis
For organic analysis all samples were extracted with dichloromethane in an automatic extractor (Büchi B-811). Surrogate extraction standards (PCB30, PCB185,13C BDEs 28, 47, 99, 100, 153, 154, 183, 209) were spiked on each sample prior to extraction. The volume was reduced after extraction under a gentle nitrogen stream at ambient temperature, and clean-up was achieved on a Florisil column. Samples were analysed using a GC-MS/MS (gas chromatograph coupled with a tandem mass spectrometer) Agilent 7890 coupled to Agilent 7000B with a SGE HT-8 column () for α-, β-HCH, γ- and δ-HCH (i.e. four HCH isomers), o, p′- and p, p′-DDE, -DDD and -DDT (six DDX compounds), penta- and hexachlorobenzene (PeCB, HCB), PCB28, −52, −101, −118, −153, −138 and −180 (i.e. seven indicator PCBs), aldrin, dieldrin, endrin, α- and γ-chlordan, α- and β-endosulfan, endosulfan sulfate and mirex. More details are given in the Supplement Sect. S1.1.
The mean of three field blank values was subtracted from the air sample values. Values below the mean + 3 standard deviations of the field blank values were considered to be < LOQ. Field blank values of a number of analytes in air samples were below the instrument limit of quantification (ILOQ), which corresponded to 0.006–0.012 pg m−3 for PCB/OCPs and 0.50–5.2 pg m−3 for PBDEs (Table S1 in the Supplement). LOQs ranged 0.006–0.06 pg m−3 for PCBs, 0.006–0.12 pg m−3 for OCPs (with few exceptions higher) and 0.001–0.01 pg m−3 for PBDEs (Supplement Sect. S1.1, Table S1).
Organic and elemental carbon in filter samples, as well as total organic carbon in soil was determined by a thermal-optical method (Sunset Lab., USA; EUSAAR protocol).
The pollutant fugacities (Harner et al., 2001) have been derived from concentrations in soil and air (details in Supplement Sect. S1.2). The onset of the monsoon on site was dated with high temporal resolution based on air parcel history (back trajectory analysis, Supplement Sect. S1.3).
2.3 Modelling atmospheric transport, chemistry and air–soil exchange
The response of air–soil exchange to the drop in air concentration, subsequent to the monsoon onset, was studied by the regional-scale simulation of meteorology and chemistry using the WRF-Chem PAH/POP model. The WRF-Chem PAH/POP has been recently extended from the regional model WRF-Chem version 3.6.1 (Grell et al., 2005; Mu et al., 2018), to also represent the chemistry, in- and below-cloud scavenging, gas-particle partitioning and surface gas exchange of semi-volatile organics (described in Supplement Sect. S1.4.1, input data in S1.4.3). The simulation of the period 1–30 June 2014, with a spatial resolution of 27×27 km2; and a time step of 150 s of the southern Asian domain (5–32∘ N,69–89∘ E), was driven by NCEP reanalyses (6 h, resolution). Physical and chemical spin-up time was 4 days. Primary emissions were considered for DDT and PCBs (Supplement Sect. S1.4.1), while the secondary emissions were modelled based on initialising the soils of India uniformly by the observed levels in background soils (shrub, forest, Sect. 2.1). Non-zero air concentrations, observed before and during the monsoon at the site (see above), were advected continuously at all boundaries of the domain (Supplement Sect. S1.4.1). In the model experiment pre-monsoon levels were continuously replaced by monsoon levels according to the northward propagation of the monsoon, while in the control run pre-monsoon levels were kept constant at the boundaries.
2.4 Multi-decadal simulation of pollution of air and soil in India
The air–soil mass exchange flux of the semi-volatile organic compounds studied were simulated by a non-steady state one-dimensional (series of 7 two-boxes) model of inter-compartmental mass exchange (multimedia mass balance model (Lammel, 2004; Fig. S1 in the Supplement). The boxes represent 7 zones in the north–south direction in India, 7.4–33.4∘ N, each 3.75∘ wide. For each box the mass balances for the two compartments planetary, boundary layer and top soil, were solved. The processes considered in air are wet and dry (particle) deposition, chemical removal from air by reaction with the hydroxyl radical, air–surface mass exchange flux (dry gaseous deposition and volatilisation) and loss by transport to the free troposphere, while in the soil atmospheric deposition fluxes, air–surface mass exchange flux and degradation (as first order process) were considered (Supplement Sect. S1.4.2, input data in Sect. S1.4.3). In addition to a 50-year model run, the sensitivity of soil pollution to a number of input parameters as well as under a hypothetic no-monsoon scenario was studied.
3.1 Field observations
Relatively low pollution levels in soils (0.07–0.11 ng g−1 for Σ4HCH, 0.18–0.43 ng g−1 for Σ6DDX, 0.25–0.28 ng g−1 for Σ7PCB and 8.1–12.7 pg g−1 for Σ9PBDEs) confirm the classification as a background site (Table 1). Actually, these HCH and DDX levels are lower than ever reported from soils in India, which previously ranged 1.6–835 for HCH (excluding hotspots; Sharma et al., 2014), 14–934 ng g−1 for DDX (Sharma et al., 2014) and 30 (0–149) pg g−1 (rural sites, same congeners; Li et al., 2016). The soil sample from a tea plantation showed elevated levels of DDT and its metabolites (27.9 ng g−1 Σ6DDX), pointing to previous application (Table S2).
Table 1Observed concentrations in soil, cs (ng g−1; together with standard deviation based on 3 replicates) of (a) pesticides, (b) PCBs and (c) PBDEs (quantified species only). TOC is total organic carbon content (% of dry mass).
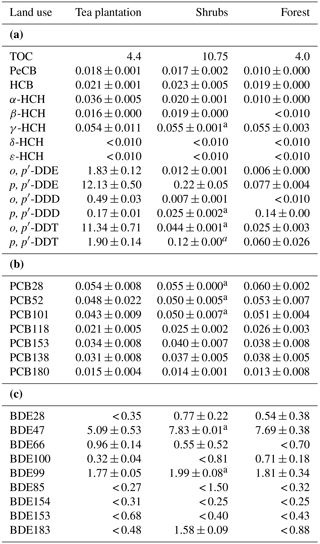
a Based on two replicates.
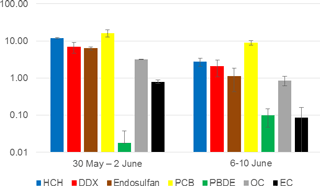
Figure 1Observed concentrations in air, ca, of pesticides (Σ4HCH, Σ6DDX, Σ3Endosulfan), Σ7PCB, Σ9PBDE (pg m−3), OC and EC (µg m−3) before and after the onset of the south-westerly monsoon in Munnar, India, 2014. Error bars reflect standard deviations. All concentration changes are significant (p < 0.05 level, t test) except for PBDE.
Indeed, measured air concentrations of carbonaceous aerosol and organic pollutants reach a distinctly lower level during the monsoon, dropping by a factor of 2–10, except for PBDEs, which apparently increased (Fig. 1, Table S3). These concentration changes, i.e. 77 %, 70 %, 82 % and 45 % for Σ4HCH, Σ6DDX, Σ3Endosulfan and Σ7PCBs from before to after (Fig. 1) were all significant on the p < 0.05 level, most on the p < 0.01 level, except for PBDEs, which were insignificant even on the p < 0.1 level (unpaired Student t test). Precipitation increased by a factor of ≈2 at the monsoon onset (from 3.8 to 8.0 mm day−1), associated with convective activity (Valsan et al., 2016). With 2.3–17.7 pg m−3 Σ4HCH and 0.36–10.4 pg m−3 Σ6DDX (Table S3) the measurements at Munnar range at the lower end of the range reported from rural sites in India in years after a ban in agriculture (listed in Table S6c). 1.3–8.5 pg m−3 endosulfan (including endosulfan sulfate) measured in Munnar in 2014, shortly after the ban of the pesticide is 3 orders of magnitude below what was reported from 2006 to 2007 (i.e. 1000–9200 pg m−3 at rural locations in southern India; Pozo et al., 2011). Similarly, the range of 2.8–70 pg m−3 Σ7PCBs measured in 2014 at Munnar lies distinctly below 32–440 pg m−3, reported for the same substances at rural coastal sites in 2006 (Zhang et al., 2008).
The fugacity ratio fs∕fa is used to characterise air–soil exchange (Supplement Sect. S1.2). Calculations indicate both downward (PCB180, DDT and metabolites over forest and shrub soils, BDE99) and upward (PCB101, PeCB, DDT and metabolites over tea garden soils, BDE28, BDE47; Fig. 2) diffusive air–soil exchange fluxes prior to the monsoon. With the monsoon onset fs∕fa generally increases (except for PBDEs, for which concentrations in monsoon air were somewhat elevated compared to pre-monsoon air; Table S3). This can trigger a change in flux direction for the tri- to hexachlorinated PCBs (i.e. all targeted except PCB180) and α− and β-HCH (Fig. 2). For example, α− and γ-HCH were close to phase equilibrium before onset, but net volatilisation occurred during the monsoon, while β-HCH changed from net-depositional to near phase equilibrium.
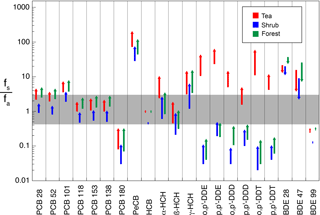
Figure 2Change in air–soil chemical equilibrium with the monsoon onset. Arrows denote direction and amount of change in the fugacity ratio, fs∕fa, over various soils from prior to posterior onset. fs∕fa < 1 denotes downward (net deposition) while fs∕fa > 1 denotes upward (net volatilisation) flux. The shaded zone (0.33 < fs∕fa < 3) indicates insignificance of deviation from 1 due to input data uncertainties.
3.2 Response of air–soil gas exchange of pollutants to monsoon onset
Findings from the field campaign were used to constrain the regional WRF-Chem model simulations for the Indian subcontinent. In the model experiment the pre-contaminated soil (as observed at the background site, mean of soil samples) is exposed to a drop of atmospheric concentrations, forced from the domain boundaries along with the monsoon onset and its northward propagation. In a control experiment pre-monsoon air concentrations are prescribed at the boundaries throughout the simulation (detailed in Methods and Supplement Sect. S1.4.1).
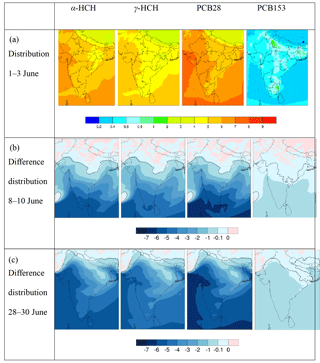
Figure 3Air pollutant distributions. α-HCH, γ-HCH, PCB28 and PCB153 (pg m−3) predicted concentrations in near-ground air prior to monsoon onset (1–3 June, a) and difference distributions due to monsoon advection (experiment – control; b: 8–10 June, c: 28–30 June 2014). The difference is significant (p < 0.05, t test) south of the dotted line.
Within a few days after the monsoon onset in southern India, the advection of air from the Indian Ocean reduced atmospheric levels of HCH and PCB over southern India and the Bay of Bengal, and to a lesser extent over central India (Fig. 3, centre panels). Three weeks after onset in southern India, the northern monsoon boundary has passed over India except the north-western states of Gujarat and Rajasthan (i.e. north of ≈22∘ N and west of ≈77∘ E; Valsan et al., 2016), but the distributions of HCH and PCB in air maintain significant gradients with high, i.e. only moderately reduced (by < 1 pg m−3) levels in the north and east, and low levels after a decline of > 3 pg m−3 of HCH isomers and > 5 pg m−3 of PCB28, respectively, in the south and south-west. The response of the air–soil system to the monsoon leads to a spatially inhomogeneous distribution of pollutants across India. It is dominated by clean air advection in the south and south-west but only moderately decreased air pollution in northern and eastern parts of the subcontinent, as the air receives secondary emissions from the soils. The secondary emissions increase with distance from the coasts after the monsoon onset. The differences in concentrations before and during the monsoon are significant (p < 0.05, t test) in southern and central India and parts of the north (Fig. 3). The model results show that HCH isomers and PCB28 concentrations drop by ≈80 %, ≈20 % and ≈4 % at 9, 22 and 29∘ N, respectively, PCB153 by ≈40 % and ≈10 % at 9 and 22∘ N, respectively, while they increase by ≈1 % at 29∘ N (Table S5a). The model realistically reproduces the decline in atmospheric concentration at the field site (southern India, 9∘ N; Table S6a, b). In the model, the HCH and PCB volatilisation fluxes are enhanced in the south (by 0.02–0.78 pg m−2 h−1 i.e. 3–11 %; Table S5b) by the drop in air pollution, to a lesser degree in central India (0.002–0.19 pg m−2 h−1), and by an even smaller or negligible amount at a site in northern India (< 0.0001–0.007 pg m−2 h−1).
The south-westerly summer monsoon is associated with strong convection that effectively lifts air pollution to high altitudes in the troposphere. The monsoon outflow from India is predominantly directed towards western Asia, Africa and the Mediterranean, while a smaller fraction is transported towards eastern Asia (Lawrence and Lelieveld, 2010).
3.3 Multidecadal air–surface cycling of POPs and historic trends
To put the above described seasonal feature into historical context, with long-term trends of air–soil contamination, a multimedia mass balance box model was developed and applied to several measured contaminants.
As a result of historical applications in agriculture and industry, POPs have been accumulating in soils in India over decades (Fig. S3), partly continuing beyond peak emission. The atmospheric concentrations of PCBs have decreased since ≈1974 and those of α-HCH and DDT since ≈1989, but soil concentrations only decreased for p, p′-DDT and levelled off for α-HCH (Fig. S3a, b), or are even still on the rise (PCB153; Fig. S3d). Apart from changes over time, in general related to substance usage, the spatial variation in the concentrations of pollutants in mostly agricultural soil in India (Ramesh et al., 1991; Kumari et al., 1996; Sharma et al., 2014) is very large, i.e. ≥2 orders of magnitude. No data from background sites are available (Table S6c). The simulated pesticide values, 0.5–20 ng g−1 α-HCH and 50–5000 and 1–200 ng g−1 DDT in the 1990s and 2000s, respectively (Fig. S2), fall into the ranges spanned by the observations (Table S6c). For PCBs, no soil data were reported (UNEP, 2002).
A north–south gradient is predicted for the pollutants (Fig. S3), which is certainly influenced by the emission distribution (maximum in northern India, in the Indo-Gangetic Plain) as well as the direction of advection in air (prevailing westerly, with northerly component). For α-HCH, this gradient was also reflected in soil distributions in India, which were based on a gridded mass balance model (Xu et al., 2013). While PCB28 had net volatilisation after a few years upon release into the environment, this was much later for the highly lipophilic PCB153, with ≈1 decade in southern India, ≈2 decades in central and even longer in northern India (Fig. 4d). Nowadays, the diffusive air–surface exchange flux of the pesticides α-HCH and DDT is expected in the 0.1–1 fg m−2 h−1 range, several orders of magnitude lower than before or shortly after the ban (Fig. 4a, b). In contrast and related to ongoing emissions from old industrial facilities, the strong decrease in PCB usage did not strongly impact air–surface cycling. The magnitude of fluxes remained within the same order of magnitude, 0.1–1 fg m−2 h−1, being even on the rise in the case of PCB153 (Fig. 4c, d). The air–ground fluctuations are expectedly mediated by the storage of part of the pollutant burden in vegetation (not resolved in the model).
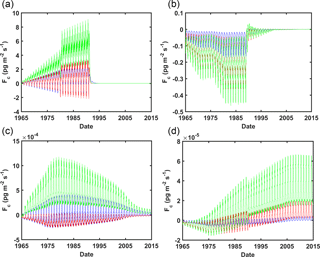
Figure 4Predicted multidecadal diffusive air–surface exchange fluxes. 1-D model. Fc (positive is upward, negative is downward; lower) of (a) α-HCH, (b) p, p′-DDT, (c) PCB28, (d) PCB153 in the northern (29.7–33.4∘ N, blue), central (18.5–22.3∘ N, red) and southern (7.4–11.2∘ N, green) zones of India during 1965–2014. Predicted concentrations in air and soil are shown in Fig. S3 in the Supplement.
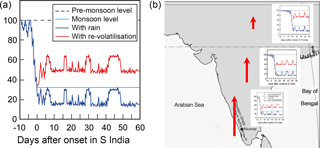
Figure 5Illustration of temporal (a) and spatial (b) variation in semi-volatile and persistent substance advection over southern, central and northern India in response to the monsoon onset and its northward propagation. Field site is Munnar.
The results of a simulation of a fictive no-monsoon scenario suggest that the effects of the monsoon have been limiting pollution of soils by HCH and PCB28 somewhat (< 20 % in 2014), while they have been contributing to DDT and PCB153 in soils by ≈50 % and ≈10 %, respectively (Supplement Sect. S2.2.3, Table S8). This suggests that the monsoon's effect on revolatilisation of soil burdens in response to drop in air concentrations at its onset is a secondary effect for DDT and PCB153, while the monsoon's enhancement of air-to-soil transfer by wet deposition is the primary effect. This trend could be explained by the higher significance of wet deposition for DDT and PCB153, which partition the particulate phase more than HCH and PCB28, whereas the efficiency of gas scavenging is generally low for POPs (Atlas and Giam, 1988; Bidleman, 1988; Shahpoury et al., 2015).
Both the field measurements and modelling results of this study indicate a so-far overlooked mechanism of pollutant cycling over the Indian subcontinent, i.e. monsoon-driven mobilisation of POPs from previously contaminated soils. The decline of POP levels in the south-westerly flow at the monsoon onset is partly related to the advection of clean air from the Indian Ocean (seasonal shift of the ITCZ), and partly by the washout of particulate pollutants (Fig. 5), as well as deepening of the planetary boundary layer. In contrast, washout of gaseous organic pollutants is very limited, because of low water solubility (Atlas and Giam, 1988; He and Balasubramanian, 2010; Shahpoury et al., 2015). Because of the convective vertical transport during the monsoon, pollutants can be released at the cloud top and subsequently undergo long-range transport in the upper troposphere over and beyond southern Asia. During transport over the Indian subcontinent near the surface, air masses collect pollution emitted from primary and secondary sources at the ground in urban and rural areas. We have shown here that secondary sources are partly triggered by the low concentrations in relatively pristine air, which are most pronounced in areas that receive marine background air, i.e. in south-western India. This corresponds to a seasonal decrease in the soil burden by a few percent relative to the annual mean. This secondary source (revolatilisation) weakens as a function of distance from the coast, as the monsoon advection propagates across the subcontinent (Table S4b, Fig. 5). The 2014 south-westerly monsoon was relatively weak (South Asian summer monsoon index; Li and Zeng, 2002) compared to the long-term mean. For strong monsoon events, more efficient air-to-soil transfer of pollutants by wet deposition could result. The simulation under a no-monsoon scenario suggests that the latter process dominates for the least water soluble and least volatile (high partitioning to the particulate phase) pollutants. Scavenging and air-to-soil transfer of POPs under monsoon rain had hardly been studied in the field and should be addressed.
Secondary emissions, originating from past deposition to soils, also contribute to the long-range transport of atmospheric POPs to remote areas in central Asia (Sheng et al., 2013; Gong et al., 2015). A similar trend of pollutant release from soils can be expected for other semi-volatile organic substances such as polycyclic aromatic hydrocarbons (actually indicated by observations on site, not reported here) and brominated chemicals.
All data needed to evaluate the conclusions in the paper are present in the paper and/or the Supplement. Additional data related to this paper may be requested from the authors.
The supplement related to this article is available online at: https://doi.org/10.5194/acp-18-11031-2018-supplement.
GL conceived the study. SSG, GL, AM, PS, and AEV conducted the air and soil sampling and field measurements. OA, PK and PP did the chemical analysis of samples. GL and SSG did the field data analysis. CVB, and CD and MDM provided on-site support and meteorological analyses, respectively. CD, QM, MO and IS prepared model input. CD and GL designed the 1-D model. CD and QM incorporated model parameterizations, and performed and analysed the 1-D and 3-D model runs, respectively. CD, SSG, GL, and IS discussed the results. GL wrote the manuscript with input from all co-authors.
The authors declare that they have no conflict of interest.
We thank Tabish Umar Ansari (IIT) and Roman Prokeš (MU)
for on-site and logistic support, Milán Sáňka (MU) for
assistance with soil sampling and data, and Jos Lelieveld (MPIC), Andreas
Fink (Karlsruhe Institute of Technology) and Fei Ge (MPIM) for discussion.
The MU RECETOX Research Infrastructure was supported by the Czech Ministry of Education, Youth and Sports
(LM2015051 and CZ.02.1.01/0.0/0.0/16_013/0001761).
Sachin S. Gunthe acknowledges
the financial support from DST-Max Planck Partner Group on Bioaerosol
Research at IIT.
The article processing charges for this open-access
publication were covered by the Max Planck
Society.
Edited by: Paul O. Wennberg
Reviewed by: two anonymous referees
Atlas, E. and Giam, C. S.: Ambient concentration and precipitation scavenging of atmospheric organic pollutants, Water Air Soil Poll., 38, 19–36, 1988.
Bajwa, A., Ali, U., Mahmood, A., Chaudhry, M. J. I., Jabir, H. S., Li, J., Zhang, G., Jones, K. C., and Malik, R. N.: Organochlorine pesticides (OCPs) in the Indus River catchment area, Pakistan: Status, soil-air exchange and black carbon mediate distribution, Chemosphere, 152, 292–300, 2016.
Bidleman, T. F.: Atmospheric processes wet and dry deposition of organic compounds are controlled by their vapor-particle partitioning, Environ. Sci. Technol., 22, 361–367, 1988.
Bidleman, T. F.: Atmospheric transport and air-surface exchange of pesticides, Water Air Soil Poll., 115, 115–166, 1999.
Breivik, K., Gioia, R., Chakraborty, P., Zhang, G., and Jones, K. C.: Are reductions in industrial organic contaminants emissions in rich countries achieved partly by export of toxic wastes?, Environ. Sci. Technol., 45, 9154–9160, 2012.
Chakraborty, P. and Zhang, G.: Organochlorine pesticides, polychlorinated biphenyls, and polybrominated diphenylethers in the Indian atmosphere, in: Global contamination trends of persistent organic chemicals, edited by: Loganathan, B. G. and Lam, P. K. S., CRC Press, Boca Raton, FL, USA, 179–202, 2012.
Chakraborty, P., Zhang, G., Li, J., Sivakumar, A., and Jones, K. C.: Occurrence and sources of selected organochlorine pesticides in the soil of seven major Indian cities: assessment of air-soil exchange, Environ. Pollut., 204, 74–80, 2015.
Cousins, I. T., Beck, A. J., and Jones, K. C.: A review of the processes involved in the exchange of semivolatile organic compounds (SVOC) across the air-soil interface, Sci. Total Environ., 228, 5–24, 1999.
Degrendele, C., Audy, O., Hofman, J., Kučerik, J., Kukučka, P., Mulder, M. D., Přibylová, P., Prokeš, R., Sáňka, M., Schaumann, G. E., and Lammel, G.: Diurnal variations of air-soil exchange of semi-volatile organic compounds (PAHs, PCBs, OCPs and PBDEs) in a central European receptor area, Environ. Sci. Technol., 50, 4278–4288, 2016.
FAO: World reference base for soil resources 2014, United Nations Food and Agricultural Organisation World Soil Resources Reports Vol. 106, FAO, Rome, Italy, 191 pp., 2014.
GOI: India soil map, Government of India, National Bureau of Soil Survey and Land Use Planning, Nagpur, India, 1985.
Gong, P., Wang, X. P., Xue, Y. G., Sheng, J. J., Gao, S. P., Tian, L. D., and Yao, T. D.: Influence of atmospheric circulation on the long-range transport of organochlorine pesticides to the western Tibetan Plateau, Atmos. Res., 166, 157–164, 2015.
Grell, G. A., Peckham, S. E., Schmitz, R., McKeen, S. A., Frost, G., Skamarock, W. C., and Eder, B.: Fully coupled “online” chemistry within the WRF model, Atmos. Environ., 39, 6957–6975, 2005.
Harner, T., Bidleman, T. F., Jantunen, L. M. M., and Mackay, D.:. Soil-air exchange model of persistent pesticides in the U.S. Cotton Belt, Environ. Toxicol. Chem., 20, 1612–1621, 2001.
He, J. and Balasubramanian, R.: The exchange of SVOCs across the air-sea interface in Singapore's coastal environment, Atmos. Chem. Phys., 10, 1837–1852, https://doi.org/10.5194/acp-10-1837-2010, 2010.
IMD: Climate diagnostic bulletin of India – Monsoon season (June–September) 2014, National Climate Centre, Indian Meteorological Department, Pune, India, 23 pp., 2014.
Kumari, B., Singh, R., Madan, V. K., Kumar, R., and Kathpal, T. S.: DDT and HCH compounds in soils, ponds and drinking water of Haryana, India, B. Environ. Contam. Tox., 57, 787–793, 1996.
Kurt-Karakus, P. B., Bidleman, T. F., Staebler, R. M., and Jones, K. C.: Measurement of DDT fluxes from a historically treated agricultural soil in Canada, Environ. Sci. Technol., 40, 4578–4585, 2006.
Lammel, G.: Effects of time-averaging climate parameters on predicted multicompartmental fate of pesticides and POPs, Environ. Pollut., 128, 291–302, 2004.
Lammel, G. and Stemmler, I.: Fractionation and current time trends of PCB congeners: evolvement of distributions 1950–2010 studied using a global atmosphere-ocean general circulation model, Atmos. Chem. Phys., 12, 7199–7213, https://doi.org/10.5194/acp-12-7199-2012, 2012.
Lammel, G., Klöpffer, W., Semeena, V. S., Schmidt, E., and Leip, A.: Multicompartmental fate of persistent substances: Comparison of predictions from multi-media box models and a multicompartment chemistry-atmospheric transport model, Environ. Sci. Pollut. R., 14, 153–165, 2007.
Lawrence, M. G. and Lelieveld, J.: Atmospheric pollutant outflow from southern Asia: a review, Atmos. Chem. Phys., 10, 11017–11096, https://doi.org/10.5194/acp-10-11017-2010, 2010.
Li, J. P. and Zeng, Q. P.: A unified monsoon index, Geophys. Res. Lett., 29, 1274, https://doi.org/10.1029/2001GL013874, 2002.
Li, W. L., Ma, W. L., Jia, H. L., Hong, W. J., Moon, H. B., Nakata, H., Minh, N. H., Sinha, R. K., Chi, K. H., Kannan, K., Sverko, E., and Li, Y. F.: Polybrominated diphenyl ethers (PBDEs) in surface soils across five Asian countries: Levels, spatial distribution, and source contribution, Environ. Sci. Technol., 50, 12779–12788, 2016.
Meijer, S. N., Shoeib, M., Jantunen, L. M. M., Jones, K. C., and Harner, T.: Air-soil exchange of organochlorine pesticides in agricultural soils – 1. Field measurements using a novel in situ sampling device, Environ. Sci. Technol., 37, 1292–1299, 2003.
Mu, Q., Shiraiwa, M., Octaviani, M., Ma, N., Ding, A. J., Su, H., Lammel, G., Pöschl, U., Cheng, Y. F.: Temperature effect on phase state and reactivity controls atmospheric multiphase chemistry and transport of PAHs, Sci. Adv., 4, aap7314, https://doi.org/10.1126/sciadv.aap7314, 2018.
Pozo, K., Harner, T., Lee, S. C., Sinha, R. K., Sengupta, B., Loewen, M., Geethalakshmi, V., Kannan, K., and Volpi, V.: Assessing seasonal and spatial trends of persistent organic pollutants (POPs) in Indian agricultural regions using PUF disk passive air samplers, Environ. Pollut., 159, 646–653, 2011.
Rajendran, R. B., Venugopalan, V. K., and Ramesh, R.: Pesticide residues in air from coastal environment, South India, Chemosphere, 39, 1699–1706, 1999.
Ramesh, A., Tanabe, S., Tatsukawa, R., and Subramanian, A. N.: Seasonal variations of organochlorine insecticides residues in air from Porto Novo, South India, Environ. Pollut., 62, 213–222, 1989.
Ramesh, A., Tanabe, S., Subramanian, A., Mohan, D., Venugoplan, V. K., and Tatsukawa, R.: Persistent organochlorine residues in green mussels from coastal waters of South India, Mar. Pollut. Bull., 21, 587–590, 1990.
Ramesh, A., Tanabe, S., Murase, H., Subramanian, A., and Tatsukawa, R.: Distribution and behaviour of persistent organochlorine insecticides in paddy soil and sediments in the tropical environment: a case study in South India, Environ. Pollut., 74, 293–307, 1991.
Ramesh, A., Tanabe, S., Kannan, K., Subramanian, A., Kumaran, P. L., and Tatsukawa, R.:Characteristic trend of persistent organochlorine contamination in wildlife from a tropical agricultural watershed, South India, Arch. Environ. Con. Tox., 23, 26–36, 1992.
Růžičková, P., Klánová, J., Čupr, P., Lammel, G., and Holoubek, I.: An assessment of air-soil exchange of polychlorinated biphenyls and organochlorine pesticides across central and southern Europe, Environ. Sci. Technol., 42, 179–185, 2008.
Semeena, V. S. and Lammel, G.: The significance of the grasshopper effect on the atmospheric distribution of persistent organic substances, Geophys. Res. Lett., 32, L07804, https://doi.org/10.1029/2004GL022229, 2005.
Senthilkumar, K., Kannan, K., Subramanian, A., and Tanabe, S.: Accumulation of organochlorine pesticides and polychlorinated biphenyls in sediments, aquatic organisms, birds, bird eggs and bat collected from southern India, Environ. Sci. Pollut. R., 8, 35–47, 2001.
Shahpoury, P., Lammel, G., Holubová Šmejkalová, A., Klánová, J., Přibylová, P., and Váňa, M.: Polycyclic aromatic hydrocarbons, polychlorinated biphenyls, and chlorinated pesticides in background air in central Europe – investigating parameters affecting wet scavenging of polycyclic aromatic hydrocarbons, Atmos. Chem. Phys., 15, 1795–1805, https://doi.org/10.5194/acp-15-1795-2015, 2015.
Sharma, B. M., Bharat, G. K., Tayal, S., Nizzetto, L., Čupr, P., and Larssen, T.: Environment and human exposure to persistent organic pollutants (POPs) in India: A systematic review of recent and historical data, Environ. Int., 66, 48–64, 2014.
Sheng, J. J., Wang, X. P., Gong, P., Joswiak, D. R., Tian, L. D., Yao, T. D., and Jones, K. C.: Monsoon-driven transport of organochlorine pesticides and polychlorinated biphenyls to the Tibetan Plateau: Three years atmospheric monitoring study, Environ. Sci. Technol., 47, 3199–3208, 2013.
Shunthirasingham, C., Oyiliagu, C. E., Cao, X. S., Gouin, T., Wania, F., Lee, S. C., Pozo, K., Harner, T., and Muir, D. C. G.: Spatial and temporal pattern of pesticides in the global atmosphere, J. Environ. Monitor., 12, 1650–1657, 2010.
UNEP: Regionally based assessment of persistent toxic substances – Indian Ocean regional report, United Nations Environment Programme, Châtelaine, Switzerland, 104 pp., 2002.
Valsan, A. E., Ravikrishna, R., Biju, C. V., Pöhlker, C., Després, V. R., Huffman, J. A., Pöschl, U., and Gunthe, S. S.: Fluorescent biological aerosol particle measurements at a tropical high-altitude site in southern India during the southwest monsoon season, Atmos. Chem. Phys., 16, 9805–9830, https://doi.org/10.5194/acp-16-9805-2016, 2016.
Wania, F.: Potential of degradable organic chemicals for absolute and relative enrichment in the Arctic, Environ. Sci. Technol., 40, 569–577, 2006.
Wania, F. and Mackay, D.: Global fractionation and cold condensation of low volatile organic chlorine compounds in polar regions, Ambio, 22, 10–18, 1993.
Wania, F. and Westgate, J. N.: On the mechanism of mountain cold-trappoing of organic chemicals, Environ. Sci. Technol., 42, 9092–9098, 2008.
Wong, F., Alegria, H. A., and Bidleman, T. F.: Organochlorine pesticides in soils of Mexico and the potential for air-soil exchange, Environ. Pollut., 158, 749–755, 2010.
Wong, M. H., Wu, S. C., Deng, W. J., Yu, X. Z., Luo, Q., Leung, A. O. W., Wong, C. S. C., Luksemburg, W. J., and Wong, A. S.: Export of toxic chemicals – a review of the case of uncontrolled electronic waste recycling, Environ. Pollut., 149, 131–140, 2007.
Xu, Y., Tian, C. G., Zhang, G., Ming, L. L., Wang, Y., Chen, Y. J., Tang, J. H., Li, J., and Luo, C. L.: Influence of monsoon system on α-HCH fate in Asia: A model study from 1948 to 2008, J. Geophys. Res., 118, 6764–6770, 2013.
Zhang, G., Chakraborty, P., Li, J., Balasubramanian, T., Kathiresan, K., Takahashi, S., Subramanan, A., Tanabe, S., and Jones, K. C.: Passive sampling of organochlorine pesticides, polychlorinated biphenyls, and polybrominated diphenyl ethers in urba, rural and wetland sites along the coastal length of India, Environ. Sci. Technol., 42, 8218–8223, 2008.
Zhang, T. T., Fiedler, H., Yu, G., Solorzano Ochoa, G., Carroll, W. F., Gullett, B. K., Marklund, S., and Touati, A.: Emissions of unintentional persistent organic pollutants from open burning of municipal solid waste from developing countries, Chemosphere, 84, 994–1001, 2011.