the Creative Commons Attribution 4.0 License.
the Creative Commons Attribution 4.0 License.
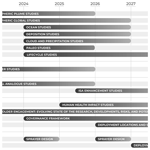
Opinion: A research roadmap for exploring atmospheric methane removal via iron salt aerosol
Katrine A. Gorham
Sam Abernethy
Tyler R. Jones
Peter Hess
Natalie M. Mahowald
Daphne Meidan
Matthew S. Johnson
Maarten M. J. W. van Herpen
Yangyang Xu
Alfonso Saiz-Lopez
Thomas Röckmann
Chloe A. Brashear
Erika Reinhardt
David Mann
The escalating climate crisis requires rapid action to reduce the concentrations of atmospheric greenhouse gases and lower global surface temperatures. Methane will play a critical role in near-term warming due to its high radiative forcing and short atmospheric lifetime. Methane emissions have accelerated in recent years, and there is significant risk and uncertainty associated with the future growth in natural emissions. The largest natural sink of methane occurs through oxidation reactions with atmospheric hydroxyl and chlorine radicals. Enhanced atmospheric oxidation could be a potential approach to remove atmospheric methane. One method proposes the addition of iron salt aerosol (ISA) to the atmosphere, mimicking a natural process proposed to occur when mineral dust mixes with chloride from sea spray to form iron chlorides, which are photolyzed by sunlight to produce chlorine radicals. Under the right conditions, lofting ISA into the atmosphere could potentially reduce atmospheric methane concentrations and lower global surface temperatures. Recognizing that potential atmospheric methane removal must only be considered an additive measure – in addition to, not replacing, crucial anthropogenic greenhouse gas emission reductions and carbon dioxide removal – roadmaps can be a valuable tool to organize and streamline interdisciplinary and multifaceted research to efficiently move towards understanding whether an approach may be viable and socially acceptable or if it is nonviable and further research should be deprioritized. Here we present a 5-year research roadmap to explore whether ISA enhancement of the chlorine radical sink could be a viable and socially acceptable atmospheric methane removal approach.
- Article
(795 KB) - Full-text XML
- BibTeX
- EndNote
Driven by anthropogenic greenhouse gas emissions, Earth's average surface temperature has increased by 1.1 °C since 1850 (Forster et al., 2021). As global temperature increases, irreversible changes in the Earth system will likely occur, such as ice sheet collapses in Greenland and Antarctica, coral reef die-off, abrupt permafrost thaw, mountain glacier loss, and Amazon rainforest dieback (Lenton et al., 2008; McKay et al., 2022). To mitigate both near-term and long-term warming we must undertake rapid, sustained action to establish a diverse portfolio of approaches to slow and then reverse the increase in atmospheric greenhouse gas concentrations, ideally reducing them to preindustrial levels. Emissions reductions must be prioritized. In addition, as near-term warming threatens to trigger climate tipping points, negative emissions approaches may be used to remove greenhouse gases already in the atmosphere and counter rising natural and uncontrollable emissions.
Methane (CH4) emissions have contributed roughly 0.5 °C to global warming relative to preindustrial times, second only to carbon dioxide (CO2) (IPCC, 2023). Methane lasts roughly a decade in the atmosphere (UNEP, 2021), with a global warming potential 83 times that of carbon dioxide over 20 years and 30 times that of carbon dioxide over 100 years (Forster et al., 2021). The concentration of methane in the atmosphere is over 2.5 times preindustrial levels, and the growth rate has accelerated since 2006, with record increases in 2020 and 2021 (NOAA, 2023). Methane emissions come from anthropogenic sources (fossil fuel use, agriculture, waste and wastewater, biomass burning, etc.) and natural sources (wetlands, oceans, freshwaters, termites, permafrost, etc.), both of which are increasing (Jackson et al., 2020; Nisbet et al., 2023; Saunois et al., 2020). As the planet continues to warm and precipitation patterns change, natural methane emissions are expected to increase from wetlands, as well as from permafrost due to abrupt thaw, thermokarst lake formation and expansion, and bacterial processes (Dean et al., 2018; Neumann et al., 2019; Paudel et al., 2016; Peng et al., 2022; Zhang et al., 2023). On our current trajectory, natural emissions are estimated to increase by ∼ 30–200 Tg CH4 yr−1 by 2100 (Zhang et al., 2023; Kleinen et al., 2021). There is evidence that wetland emissions increases are already underway, with roughly half of the 2020 atmospheric methane increase attributed to wetlands (Qu et al., 2022; Peng et al., 2022; Nisbet et al., 2023; Zhang et al., 2023).
The natural sinks for atmospheric methane are oxidation by gas-phase radicals (∼ 95 %) and uptake into soil by methanotrophic bacteria and archaea (∼ 5 %) (Saunois et al., 2020). The atmospheric lifetime of methane is thus mainly determined by the oxidative capacity of the atmosphere. Approximately 550 Tg CH4 yr−1 is removed by reactions with tropospheric hydroxyl radicals (OH•); tropospheric chlorine radicals (Cl•) destroy ∼ 11 Tg CH4 yr−1 (Saunois et al., 2020). However, the tropospheric chlorine radical sink estimate is poorly constrained, ranging from 1 to 35 Tg CH4 yr−1 (Saunois et al., 2020). Recent research suggests the tropospheric chlorine radical sink could be underestimated (van Herpen et al., 2023).
Complex and nonlinear atmospheric chemistry dictates the oxidation of methane, which results in the production of formaldehyde and carbon monoxide, which is further oxidized to carbon dioxide. Hydroxyl radical oxidation of methane can result in the formation or loss of ozone, depending on the nitrogen oxide (NOx) concentrations. Chlorine radicals react to remove both methane and ozone, the principal precursor of hydroxyl radical production (Lelieveld et al., 2002; Seinfeld and Pandis, 2016). Therefore, chlorine radicals may either increase or decrease the atmospheric lifetime of methane depending on the concentration of chlorine radicals and atmospheric conditions (Li et al., 2022; van Herpen et al., 2023; Q. Li et al., 2023). Volatile organic compounds and NOx will change the methane response since they directly and indirectly affect the concentrations of hydroxyl and chlorine radicals. Furthermore, since reactions with carbon monoxide and methane are the primary sinks for hydroxyl radicals, the abundance of methane impacts the oxidative character of the atmosphere in a self-feedback process (Lelieveld et al., 2002; Staniaszek et al., 2022; Holmes, 2018).
Enhanced atmospheric oxidative sinks could increase the rate of atmospheric methane removal and therefore reduce near-term warming. While it is not a replacement for much-needed anthropogenic emissions reductions, enhancing atmospheric oxidative sinks may be an important negative emission approach considering projected ongoing elevated natural methane emissions. One untested proposal involves iron salt aerosol (ISA). This potential approach involves lofting iron-based particles into the troposphere (e.g., from ships or towers) to catalytically produce chlorine radicals (Oeste, 2009; Oeste et al., 2017), mimicking a natural phenomenon proposed to occur when mineral dust combines with sea spray aerosols (van Herpen et al., 2023). Discussing natural analogues of this process and the current state of research, this paper presents a roadmap for research and development that is needed to understand whether ISA enhancement of the chlorine radical sink may be a feasible, scalable, and safe approach for atmospheric methane removal.
Currently, research into ISA falls into three categories: laboratory experiments to quantify the details of the mechanism; observational analysis of the natural analogue of ISA, known as mineral dust sea spray aerosol (MDSA); and numerical modeling evaluating the potential impacts of ISA.
2.1 Laboratory experiments
In a series of papers, Wittmer et al. (2015a, b) and Wittmer and Zetzsch (2017) demonstrated the production of chlorine atoms from iron-doped salts and aerosols. Reproducing and expanding upon these laboratory studies are of the highest importance, since the mechanism of chlorine generation is poorly understood (van Herpen et al., 2023; Wittmer et al., 2015a, b; Wittmer and Zetzsch, 2017; Zhu et al., 1997). The ISA mechanism is catalytic in iron and may be catalytic in chlorine in natural environments (Wittmer and Zetzsch, 2017). Understanding of catalytic efficiency requires the study of dependencies on conditions such as the aerosol size distribution and number density, humidity, rate of supply of acidity to the system, rate of coagulation of aerosols, effects of organic chelating agents on iron activity, changes in chlorine escape probability due to aqueous chemical conditions, changes in iron activity due to aerosol chemistry, and understanding how the atmosphere behaves under high-ClOx conditions (Pennacchio et al., 2023). Furthermore, studies of real atmospheric conditions are needed to better understand suppression of the ISA mechanism in the presence of sulfate and oxalate (Wittmer et al., 2015a, b; Wittmer and Zetzsch, 2017).
2.2 The natural analogue of ISA: mineral dust sea spray aerosol
The natural mineral dust sea spray aerosol (MDSA) mechanism is proposed to occur when iron from mineral dust mixes with the chlorine in sea spray, forming iron chlorides which are photolyzed to produce chlorine radicals (van Herpen et al., 2023). ISA could mimic this natural MDSA phenomenon by only aerosolizing what is currently believed to be the key component of the mineral dust: the photoactive iron.
The first evidence that methane may be removed over the North Atlantic by chlorine radicals produced by the MDSA mechanism was provided by van Herpen et al. (2023). North African semi-arid regions are the dominant source of iron-containing mineral dust, with frequent transport over the North Atlantic (Prospero et al., 2021). Using air samples collected in Barbados during North African dust events (Mak et al., 2003), a model parameterized with the MDSA mechanism of chlorine radical production produced results consistent with a previously unexplained 13C depletion in the reaction product carbon monoxide (CO) (van Herpen et al., 2023). Carbon monoxide produced from chlorine radical oxidation of methane is extremely depleted in 13C, which makes δ13C(CO) a very sensitive indirect detection method of chlorine radicals (Röckmann et al., 1999). The variability of δ13C(CO) in atmospheric air is the main evidence that the MDSA process is occurring, as there is no other mechanism proposed that can explain the carbon monoxide isotope signature (Mak et al., 2003).
As a proxy for methane oxidation by chlorine radicals, studies of the isotopic composition of carbon monoxide in the mid-Atlantic boundary layer are underway to further confirm the MDSA mechanism. This includes a regular air sampling program at four ground-based stations in the North Atlantic (located in Barbados, the Canary Islands, Cape Verde, and Brazil), as well as Atlantic transect sampling on board commercial vessels. Time series at the ground-based stations provide high-resolution observations at the longitudinal margins of trans-Atlantic dust transport, including the site where seasonal depletions in δ13C(CO) were previously observed (Mak et al., 2003). Air samples collected in north–south ship transects may allow for spatial correlation of the δ13C(CO) excursions with North African dust plumes. Together, these samples provide an opportunity to investigate the formation process of MDSA, as well as the seasonal and spatial influence of North African dust on tropospheric chlorine radical oxidation.
2.3 Modeling
Early modeling studies indicate that atmospheric chlorine additions can increase or decrease methane concentrations, depending on the concentration of chlorine that is present in the atmosphere (Horowitz et al., 2020; Li et al., 2022; Saiz-Lopez et al., 2023). Chlorine radicals readily oxidize ozone, the main precursor of hydroxyl radicals, which results in less methane oxidation via hydroxyls; at low atmospheric concentrations, chlorine radicals reduce ozone concentrations without having a commensurate impact on methane. Even though chlorine radicals react 16× faster with methane than the reaction of hydroxyl radicals with methane (Atkinson, 2006), hydroxyl radicals dominate the methane oxidation sink because they are much more abundant than chlorine radicals. As more chlorine is emitted, ozone concentrations will be reduced so that proportionally more chlorine radicals react with methane. The increased methane destruction by chlorine radicals will eventually outcompete decreased destruction by hydroxyl. In an initial, highly simplified model scenario, Q. Li et al. (2023) found that a reduction in methane concentration could be achieved if more than 90 Tg Cl yr−1 (3 times the estimated present-day emission rate) was added evenly to the atmosphere over all ocean surfaces, and lowering the global methane burden by 2000 Tg would require the emission of an additional 1000 Tg Cl2 yr−1. However, assuming a uniform increase in chlorine over all ocean surfaces may underestimate the potential effectiveness of local chlorine radical generation where efficiency may be condition dependent (e.g., NOx, CO, and chlorine concentrations, as well as humidity, temperature, and altitude) (Meidan et al., 2024). For example, considering the MDSA natural analogue of ISA, van Herpen et al. (2023) found that high dust concentrations in the North Atlantic corresponded with net methane removal, while globally lower dust concentrations led to a net increase in methane.
Current models may not accurately capture the speed and efficiency of producing chlorine radicals via the ISA mechanism due to assumptions of the percentage of photoactive iron in the emitted iron, aerosol pH, aerosol mixing rates, and more. Another challenge with Earth system models is that they instantaneously dilute emissions to model grid dimensions, which could lead to underestimates or overestimates of the effectiveness of the ISA mechanism, especially when considering iron emission additions from point sources like ships (e.g., Meidan et al., 2024). For example, the mixing of the iron and sea salt within the aerosol is modeled to occur instantaneously (Meidan et al., 2024; van Herpen et al., 2023); however, in reality it would likely take hours to days, leading the global model to overestimate the rate of chlorine radical production. Furthermore, the ISA mechanism is likely to occur faster in high-NOx environments (Oeste et al., 2017) but could be less efficient in high-sulfate environments (Bondy et al., 2017; Chen et al., 2020; Legrand et al., 2017; Pio and Lopes, 1998), and both NOx and sulfate may be co-emitted with iron (e.g., from a ship plume). Thus, models that instantaneously dilute emissions across the grid dimensions may misrepresent the ISA mechanism. Overall, it is unclear whether current Earth system models overestimate or underestimate the efficiency of the oxidation mechanism. Additional detailed box modeling focusing on deployment sites and constrained by field observations are necessary to assess the effectiveness of the mechanism. However, local box models are less reliable over timescales where mixing between different air masses is relevant. This motivates the need for high-resolution regional and seasonal modeling over ocean basins and variable-resolution configurations embedded in global models.
Considering the difficulty of simulating variable atmospheric chemical conditions (e.g., atmospheric chemical composition, solar radiation, wind mixing) across different geographic locations, it is important to develop an ensemble of models that enable uncertainty assessment. Such a model ensemble will allow for a comprehensive exploration of different iron salt aerosol deployment scenarios (magnitude each year), aerosol particle sizes, and deployment location and timing.
3.1 Roadmap framework
Roadmaps are used in climate research to define knowledge gaps, needs, and associated outputs as they relate to interdependencies and timelines, particularly in instances that benefit from integrated, interdisciplinary research. Recent examples include geochemical carbon dioxide removal (Maesano et al., 2022), ocean-based carbon dioxide removal (Ocean Visions, 2023), ice sheet contributions to sea level rise (Aschwanden et al., 2021), and solar radiation management (Wanser et al., 2022). A coordinated, thorough, and science-based approach is needed to ensure that resources are used efficiently, stakeholders and interdisciplinary teams are engaged on appropriate timelines, and efforts are focused towards sequenced research questions and milestones.
3.2 Viability assessment
The viability of an atmospheric methane removal approach can be assessed by considering its potential for feasibility, scalability, and social license to operate. A feasible approach must be climate beneficial, safe, acceptable considering its side effects, and cost-plausible. Determination of scalability will be approach-specific, acknowledging that the scale of increased natural emissions is anticipated to be tens of millions of tonnes of methane per year (Kleinen et al., 2021).
The key milestone questions below can help determine the viability of ISA and whether it should continue to be prioritized. The research that informs the key milestone questions should be pursued in parallel (Table 1 and Fig. 1).
- 1.
Is enhancement of the chlorine radical oxidative sink of methane via the ISA mechanism effective and climate beneficial? If so, at what scale?
- 2.
What impacts could the ISA approach have on Earth systems and human systems, both positive and negative? Is there a cost-plausible ISA deployment method?
- 3.
What is needed to advance a structure of ethical governance and social license for utilizing atmospheric intervention to reduce atmospheric methane concentrations?
Table 1Key research and development activities for a 5-year timeline beginning in 2023. Funding is needed for all research and development activities, including those that are already underway.
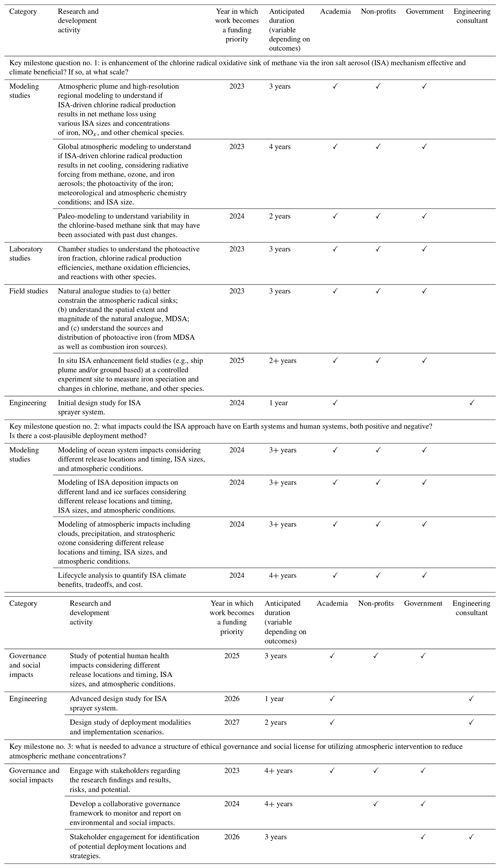
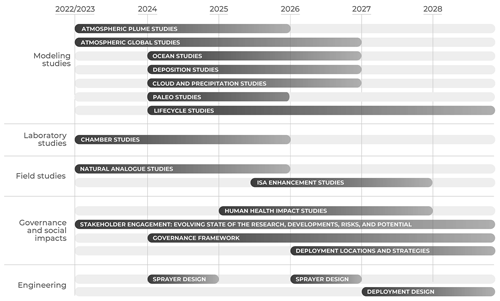
Figure 1Roadmap of ISA research and development needs. Duration of research and development timelines may be longer or shorter than depicted and/or exceed the 5-year horizon.
3.2.1 Milestone question no. 1: is the ISA mechanism effective and climate beneficial? If so, at what scale?
The complexity and nonlinearity of atmospheric chemistry and meteorology require laboratory, field, and plume and global modeling studies of the efficiency of chlorine radical production, its dependence on atmospheric conditions and other gases, and the impact on methane removal.
An important assumption in previous studies is that only 1.8 % of iron is photoactive (van Herpen et al., 2023; Meidan et al., 2024). However, the amount of ISA that is photoactive may vary by emission source (e.g., ship emissions may have more photoactive iron relative to mineral dust; Ito, 2013; Rodriguez et al., 2021), geographical location, aerosol pH, the presence of other chemical constituents (e.g., sulfate and NOx), and altitude (Mahowald et al., 2018). Furthermore, the rate of chlorine production – and subsequent rate of methane oxidation – per mass of photoactive iron is estimated to result in the removal of 45 CH4 molecules per iron atom per day (van Herpen et al., 2023) but has many uncertainties, including the time that iron remains in the atmosphere, which may be impacted by large regional variability in deposition rates (Meidan et al., 2024). The efficiency, cost, safety (e.g., air quality), and net radiative forcing of ISA will depend on the percentage of iron that is photoactive, the rates of chlorine production and methane oxidation per mass of photoactive iron, and the lifetime of photoactive-iron-based aerosol.
Current studies assume that the chlorine radicals released from the photochemical reaction with iron will react (e.g., with methane) to form hydrochloric acid, which will then be reabsorbed back into the aerosol and thus recycled (van Herpen et al., 2023; Oeste et al., 2017). It is unclear under which atmospheric conditions this cycle occurs, but if some chlorine radicals are lost then the cycling would be less efficient. One potential mechanism by which the cycling efficiency could be reduced is if hydrochloric acid is produced and deposited into the ocean (Wang et al., 2019). Therefore, further laboratory measurements and detailed box models are needed to further study hydrochloric acid recycling efficiency by ISA.
A better understanding of how sulfur dioxide and NOx concentrations impact the ISA mechanism is also needed (Oeste et al., 2017). Sulfur dioxide and NOx concentrations vary regionally and locally, and their emissions may sometimes coincide with those of iron.
Smaller ISA particles have greater surface area to mass ratios and stay longer in the atmosphere, likely increasing the efficiency of the ISA mechanism. Furthermore, smaller particles tend to be more acidic (Pye et al., 2020), which is required for the mechanism to be active (Wittmer et al., 2015a, b; Wittmer and Zetzsch, 2017). However, smaller aerosols may be transported further to coastal or inland locations where these particles could contribute directly to negative human health effects or deposit on terrestrial or ice-covered surfaces with unintended consequences. The aerosol size may also affect marine cloud cover, thereby influencing local radiative forcing. In addition, there tends to be more sulfate in smaller aerosols, which may reduce the effectiveness of the ISA mechanism (Bondy et al., 2017; Chen et al., 2020; Legrand et al., 2017; Pio and Lopes, 1998).
Studying MDSA (the natural analogue of ISA) through field studies is essential to understand the effectiveness of this mechanism under different atmospheric conditions and its geographical extent, thereby better constraining atmospheric and Earth system models. Early MDSA field studies are underway to explore the seasonality and spatial extent of methane oxidation by chlorine radicals that may occur in natural dust plumes through proxy measurements of δ13C(CO). Further studies – both natural analogue and in situ ISA enhancement studies – would benefit from alternative ISA detection and quantification approaches, including direct chlorine measurements or additional proxy measurements to reinforce existing observations.
Ideally, models will be developed to include the entire MDSA mechanism, including implementation of the isotope effect in the chlorine radical reaction with methane, thus enabling direct comparison of model results to observations of δ13C(CO). At present, some models (EMAC; Gromov, 2014) include complete carbon monoxide isotope representation but not the MDSA mechanism, whereas other models (CAM-CHem; van Herpen et al., 2023) include an initial representation of the MDSA mechanism but do not incorporate the isotopic effects.
Isotopic signatures and dust from ice core paleo-records may elucidate evidence of historical MDSA activity. Methane isotope measurements of air trapped in polar ice cores have been used to constrain the methane budget in the past (Bock et al., 2017; Sapart et al., 2012; Fischer et al., 2008; Ferretti et al., 2005), but possible variations in the chlorine-based methane sink have not been taken into account in these studies. Dust levels have undergone strong changes in the past (e.g., Fischer et al., 2007; Han et al., 2018; Yuan et al., 2020; Y. Li et al., 2023), and associated changes in MDSA may have affected the paleo-records of δ13C(CH4).
If modeling, laboratory studies, and natural analogue field studies prove that a promising, safe mechanism exists to produce chlorine radicals at sufficient scale and under diverse atmospheric conditions, it may be appropriate to consider field studies with intentional enhancement of ISA, using a broad suite of atmospheric measurements to understand how the mechanism performs in the real atmosphere (see Sect. 3.2.3). Prior to performing any ISA enhancement field studies – even at a small and controlled scale – it is essential to engage and work collaboratively with potentially impacted communities, policy and science leaders, governmental bodies, NGOs, media, and other stakeholders to ensure that actions are conducted with social license and an appropriate governance framework with free, prior, and informed consent (FAO, 2016). For example, a study could involve controlled enhancement of dust or emitted aerosolized iron or could investigate existing anthropogenic emissions of iron (e.g., from a ship plume, power plant, iron foundry). The scale of the study should be suitable to accommodate a likely nonlinear atmospheric response, where substantial increases in chlorine – thus iron emissions – may be needed before there is a decrease in methane.
3.2.2 Milestone question no. 2: what are the potential Earth system and human system impacts of ISA, and is it cost-plausible?
Lifecycle analyses are necessary to assess the potential benefits, tradeoffs, risks, uncertainties, and costs of ISA. As part of this analysis, understanding the potential impacts of ISA enhancement on the Earth system and human system is imperative before considering large-scale deployment. Human system impacts may include human health outcomes, as well as indirect human impacts from Earth system changes. For example, if ISA resulted in ocean acidification there could be marine life implications, resulting in economic, biodiversity, and cultural impacts on coastal communities. Furthermore, if chlorine drifts into urban areas it could stimulate ozone formation and cause negative human health impacts (Wang et al., 2020).
Earth system modeling must be conducted to understand the impact of atmospheric conditions and aerosol size as well as release locations, magnitudes, and timing on ocean systems, terrestrial systems, the cryosphere, the stratosphere, and clouds and precipitation. Results from atmospheric modeling (plume and global), laboratory studies, and initial field studies will inform priorities and research directions for Earth system studies and could potentially inform the design of field studies to verify model results.
The potential broader impacts of ISA deployment beyond removing methane are not well understood. These may include co-benefits such as reductions in atmospheric black carbon (Li et al., 2021; Oeste et al., 2017) and ozone (Q. Li et al., 2023; van Herpen et al., 2023), both of which are climate-warming agents. However, there are also potential negative impacts that must be further explored, including ocean acidification, stratospheric ozone loss, adverse chemical side reactions (such as COCl2 formation), and albedo changes from deposition on ice surfaces (Q. Li et al., 2023). Iron aerosols absorb light and thus tend to warm the planet, offsetting some of the lowered radiative forcing from oxidized methane (Matsui et al., 2018; Li et al., 2021; Meidan et al., 2024). There are also potential side effects, such as indirect radiative forcing due to marine cloud brightening and carbon dioxide absorption by ocean iron fertilization (Emerson, 2019), which could be either favorable or unfavorable.
The potential effects of ISA on air quality and human health are also poorly understood. Enhanced chlorine could lead to beneficial reductions in tropospheric ozone. However, the reduced hydroxyl radical production may increase lifetimes of atmospheric trace species that may be detrimental to human health. Moreover, iron aerosols themselves present a human health risk, especially when they are small (O'Day et al., 2022). Therefore, more research is required to determine under which conditions (including deployment location and timing, particle composition, and ISA size) the air quality impact is beneficial or detrimental.
Engineering deployment modalities and implementation scenarios are one of the later steps in this roadmap, only to be pursued if earlier dependencies are addressed and ISA proves to be effective and climate beneficial with acceptable side effects. Nevertheless, to avoid delaying potential future deployment readiness and to iteratively refine design in advance of any in situ field studies, development and engineering of a nozzle sprayer delivery system could begin in parallel to early research activities.
As part of a lifecycle analysis, the cost of materials (e.g., iron), infrastructure, and other implementation resources must be assessed. To be cost-plausible, the cost per tonne of methane removed must have a viable path to becoming lower than the social cost of methane, a monetary valuation that estimates the socioeconomic impact caused by an additional metric tonne of methane (Azar et al., 2023).
3.2.3 Milestone question no. 3: what is needed to advance ethical governance and social license?
It is imperative that governance and social impacts be considered in parallel to and iteratively with the development of any atmospheric methane removal approach. Addressing the climate crisis requires engagement beyond technical solutions; collaboration and transparency between physical scientists, behavioral scientists, media, the public, policy-makers, NGOs, Indigenous peoples, and other stakeholders are essential to ensure that an ethical governance framework is established (Dowell et al., 2020; Diamond et al., 2022; Data for Progress, 2023; Carbon180, 2022). There must be effective engagement and education to co-create research questions and iteratively communicate research findings and results, as well as risks and co-benefits. Failure to do so jeopardizes the trust and sound decision making of communities and governments (The Arctic Institute, 2021), threatening our ability to critically and openly assess potential climate solution approaches through a scientific process. Ideally, an external governance framework would be developed, which is enforceable and legally binding; however, there is also value in internal governance frameworks, which may, for example, be based around a code of conduct, advisory or review boards, or other non-binding structures.
3.3 Priorities and timeline
It may be advantageous to pursue multiple research questions in parallel because the output from one research question may inform the inputs for other research questions. As such, activities can be sequenced using a prioritized timeline (Table 1 and Fig. 1), where later research activities and action areas often have multiple dependencies on earlier activities. For example, the engineering design study is suggested to begin in year 4 (2027) because it can start prior to having complete Earth system modeling results, human health study outcomes, or conclusions from ISA enhancement field studies. However, the engineering design study cannot advance to its later stages until earlier activities have been thoroughly addressed. This expedited schedule advances possible timelines to avoid delaying potential deployment readiness but is not meant to accelerate the timeline beyond appropriate caution and due diligence. Setbacks in addressing early research questions and action areas will likely result in timeline delays.
The activities outlined in this roadmap require coordinated efforts across multiple government agencies to financially support research and development, to ensure robust assessment and governance processes, and to foster international engagement. This work is valuable for multiple reasons:
- 1.
We need to understand if ISA is a feasible, scalable, and safe methane removal method or if it is nonviable and further research should be deprioritized.
- 2.
Though this roadmap is ISA-specific, the research and development needs identified here contribute to fundamental understanding of processes and mechanisms that are broadly applicable to exploration of other methane removal approaches.
- 3.
The research outlined in this roadmap will contribute to constraining the global methane budget and oxidative character of the atmosphere, which will improve our understanding of atmospheric chemistry, Earth system dynamics, and air quality.
Addressing the climate crisis requires a diverse portfolio of climate solutions. Atmospheric methane removal approaches should only be researched in addition to, not replacing, crucial anthropogenic greenhouse gas emission reductions and carbon dioxide removal. Atmospheric methane removal approaches could play a future role in overall climate change mitigation alongside aggressive anthropogenic emissions reductions, for example by dampening the impacts of anthropogenically amplified natural methane emissions (e.g., from wetlands or permafrost thawing), particularly if they become uncontrollable due to climate change. All atmospheric methane removal approaches are at a very early stage (Jackson et al., 2021; Ming et al., 2022; Spark Climate Solutions, 2023); all require further research, and none are ready for deployment. We hope that this ISA roadmap, and other atmospheric methane removal roadmaps that follow, will help accelerate, prioritize, and parallelize research that is essential to understanding which climate solutions to pursue.
No data sets were used in this article.
KAG and SA wrote the manuscript draft. TRJ, PH, NMM, DM, MSJ, MMJWvH, YX, ASL, TR, CAB, ER, and DM contributed to, reviewed, and edited the paper.
Spark Climate Solutions has made research grants in the past, and plans to continue making research grants in the future, that align with this roadmap.
Publisher’s note: Copernicus Publications remains neutral with regard to jurisdictional claims made in the text, published maps, institutional affiliations, or any other geographical representation in this paper. While Copernicus Publications makes every effort to include appropriate place names, the final responsibility lies with the authors.
We thank the two anonymous reviewers whose thoughtful and constructive comments improved the paper. We also thank Romany Webb, Sabine Fuss, Jean-François Lamarque, Paige Brocidiacono, Eric Davidson, Rob Jackson, and Celina Scott-Buechler for helpful discussions during the preparation of the manuscript.
This paper was edited by Andreas Hofzumahaus and Barbara Ervens and reviewed by two anonymous referees.
Aschwanden, A., Bartholomaus, T. C., Brinkerhoff, D. J., and Truffer, M.: Brief communication: A roadmap towards credible projections of ice sheet contribution to sea level, The Cryosphere, 15, 5705–5715, https://doi.org/10.5194/tc-15-5705-2021, 2021.
Atkinson, R., Baulch, D. L., Cox, R. A., Crowley, J. N., Hampson, R. F., Hynes, R. G., Jenkin, M. E., Rossi, M. J., Troe, J., and IUPAC Subcommittee: Evaluated kinetic and photochemical data for atmospheric chemistry: Volume II – gas phase reactions of organic species, Atmos. Chem. Phys., 6, 3625–4055, https://doi.org/10.5194/acp-6-3625-2006, 2006.
Azar, C., Martin, J. G., Johansson, D. J. A., and Sterner, T.: The social cost of methane, Climatic Change, 176, 71, https://doi.org/10.1007/s10584-023-03540-1, 2023.
Bock, M., Schmitt, J., Beck, J., Seth, B., Chappellaz, J., and Fischer, H.: Glacial/interglacial wetland, biomass burning, and geologic methane emissions constrained by dual stable isotopic CH4 ice core records, P. Natl. Acad. Sci. USA, 114, E5778–E5786, https://doi.org/10.1073/pnas.1613883114, 2017.
Bondy, A. L., Wang, B., Laskin, A., Craig, R. L., Nhliziyo, M. V., Bertman, S. B., Pratt, K. A., Shepson, P. B., and Ault, A. P.: Inland Sea Spray Aerosol Transport and Incomplete Chloride Depletion: Varying Degrees of Reactive Processing Observed during SOAS, Environ. Sci. Technol., 51, 9533–9542, https://doi.org/10.1021/acs.est.7b02085, 2017.
Carbon180: Setting DAC on Track: Strategies for Hub Implementation, https://static1.squarespace.com/static/5b9362d89d5abb8c51d474f8/t/6261d1890b76863f1047a2dd/1650577901659/Carbon180-SettingDAConTrack.pdf (last access: 7 December 2023), 2022.
Chen, Y., Cheng, Y., Ma, N., Wei, C., Ran, L., Wolke, R., Größ, J., Wang, Q., Pozzer, A., Denier van der Gon, H. A. C., Spindler, G., Lelieveld, J., Tegen, I., Su, H., and Wiedensohler, A.: Natural sea-salt emissions moderate the climate forcing of anthropogenic nitrate, Atmos. Chem. Phys., 20, 771–786, https://doi.org/10.5194/acp-20-771-2020, 2020.
Data for Progress: Advancing Equitable Deployment of Regional DAC Hubs, https://www.filesforprogress.org/memos/advancing-equitable-deployment-of-regional-dac-hubs.pdf (last access: 7 December 2023), 2023.
Dean, J. F., Middelburg, J. J., Röckmann, T., Aerts, R., Blauw, L. G., Egger, M., Jetten, M. S. M., de Jong, A. E. E., Meisel, O. H., Rasigraf, O., Slomp, C. P., in't Zandt, M. H., and Dolman, A. J.: Methane Feedback to the Global Climate System in a Warmer World, Rev. Geophys., 56, 207–250, https://doi.org/10.1002/2017RG000559, 2018.
Diamond, M. S., Gettelman, A., Lebsock, M. D., McComiskey, A., Russell, L. M., Wood, R., and Feingold, G.: To assess marine cloud brightening's technical feasibility, we need to know what to study – and when to stop, P. Natl. Acad. Sci. USA, 119, e2118379119, https://doi.org/10.1073/pnas.2118379119, 2022.
Dowell, G., Niederdeppe, J., Vanucchi, J., Dogan, T., Donaghy, K., Jacobson, R., Mahowald, N., Milstein, M., and Zelikova, T. J.: Rooting carbon dioxide removal research in the social sciences, Interface Focus, 10, 20190138, https://doi.org/10.1098/rsfs.2019.0138, 2020.
Emerson, D.: Biogenic Iron Dust: A Novel Approach to Ocean Iron Fertilization as a Means of Large Scale Removal of Carbon Dioxide From the Atmosphere, Front. Mar. Sci., 6, 22, https://doi.org/10.3389/fmars.2019.00022, 2019.
FAO (Food and Agriculture Organization): Free Prior and Informed Consent: An indigenous peoples' right and a good practice for local communities, FAO, United Nations, https://www.fao.org/3/i6190e/i6190e.pdf (last access: 7 December 2023), 2016.
Ferretti, D. F., Miller, J. B., White, J. W., Etheridge, D. M., Lassey, K. R., Lowe, D. C., Meure, C. M., Dreier, M. F., Trudinger, C. M., Van Ommen, T. D., and Langenfelds, R. L.: Unexpected changes to the global methane budget over the past 2000 years, Science, 309, 1714–1717, https://doi.org/10.1126/science.1115193, 2005.
Fischer, H., Siggaard-Andersen, M. L., Ruth, U., Rothlisberger, R., and Wolff, E.: Glacial/interglacial changes in mineral dust and sea-salt records in polar ice cores: sources, transport, and deposition. Rev. Geophys., 45, RG1002, https://doi.org/10.1029/2005RG000192, 2007.
Fischer, H., Behrens, M., Bock, M., Richter, U., Schmitt, J., Loulergue, L., Chappellaz, J., Spahni, R., Blunier, T., Leuenberger, M., and Stocker, T. F.: Changing boreal methane sources and constant biomass burning during the last termination, Nature, 452, 864–867, https://doi.org/10.1038/nature06825, 2008.
Forster, P., Storelvmo, T., Armour, K., Collins, W., Dufresne, J.-L., Frame, D., Lunt, D. J., Mauritsen, T., Palmer, M. D., Watanabe, M., Wild, M., and Zhang, H.: The Earth's Energy Budget, Climate Feedbacks, and Climate Sensitivity, Climate Change 2021: The Physical Science Basis, Contribution of Working Group I to the Sixth Assessment Report of the Intergovernmental Panel on Climate Change, edited by: Masson-Delmotte, V., Zhai, P., Pirani, A., Connors, S. L., Péan, C., Berger, S., Caud, N., Chen, Y., Goldfarb, L., Gomis, M. I., Huang, M., Leitzell, K., Lonnoy, E., Matthews, J. B. R., Maycock, T. K., Waterfield, T., Yelekçi, O., Yu, R., and Zhou, B., Cambridge University Press, Cambridge, United Kingdom and New York, NY, USA, 923–1054, https://doi.org/10.1017/9781009157896.009, 2021.
Gromov, S. S.: Stable isotope composition of atmospheric carbon monoxide: a modeling study, dissertation, Johannes Gutenberg-Universität Mainz, https://openscience.ub.uni-mainz.de/handle/20.500.12030/4250 (last access: 7 December 2023), 2014.
Han, C., Do Hur, S., Han, Y., Lee, K., Hong, S., Erhardt, T., Fischer, H., Svensson, A. M., Steffensen, J. P., and Vallelonga, P.: High-resolution isotopic evidence for a potential Saharan provenance of Greenland glacial dust, Sci. Rep., 8, 15582, https://doi.org/10.1038/s41598-018-33859-0, 2018.
Holmes, C. D.: Methane Feedback on Atmospheric Chemistry: Methods, Models, and Mechanisms, J. Adv. Model Earth Sy., 10, 1087–1099, https://doi.org/10.1002/2017MS001196, 2018.
Horowitz, H. M., Holmes, C., Wright, A., Sherwen, T., Wang, X., Evans, M., Huang, J., Jaegle, L., Chen, Q., Zhai, S., and Alexander, B.: Effects of sea salt aerosol emissions for marine cloud brightening on atmospheric chemistry: Implications for radiative forcing, Geophys. Res. Lett., 47, e2019GL085838, https://doi.org/10.1029/2019GL085838, 2020.
IPCC: AR6 Synthesis Report: Climate Change 2023, https://www.ipcc.ch/report/sixth-assessment-report-cycle/ (last access: 7 December 2023), 2023.
Ito, A.: Global modeling study of potentially bioavailable iron input from shipboard aerosol sources to the ocean, Global Biogeochem. Cy., 27, 1–10, https://doi.org/10.1029/2012GB004378, 2013.
Jackson, R. B., Saunois, M., Bousquet, P., Canadell, J. G., Poulter, B., Stavert, A. R., Bergamaschi, P., Niwa, Y., Segers, A., and Tsuruta, A., Increasing anthropogenic methane emissions arise equally from agricultural and fossil fuel sources, Environ. Res. Lett., 15, 071002, https://doi.org/10.1088/1748-9326/ab9ed2, 2020.
Jackson, R. B., Abernethy, S., Canadell, J. G., Cargnello, M., Davis, S. J., Feron, S., Fuss, S., Heyer, A. J., Hong, C., Jones, C. D., Matthews, H. D., O'Conner, F. M., Pisciotta, M., Rhoda, H. M., de Richter, R., Solomon, E. I., Wilcox, J. L., and Zickfeld, K.: Atmospheric methane removal: a research agenda, Philos. T. Roy. Soc. A, 379, 20200454, https://doi.org/10.1098/rsta.2020.0454, 2021.
Kleinen, T., Gromov, S., Steil, B., and Brovkin, V.: Atmospheric methane underestimated in future climate projections. Environ. Res. Lett., 16, 119502, https://doi.org/10.1088/1748-9326/ac1814, 2021.
Legrand, M., Preunkert, S., Wolff, E., Weller, R., Jourdain, B., and Wagenbach, D.: Year-round records of bulk and size-segregated aerosol composition in central Antarctica (Concordia site) – Part 1: Fractionation of sea-salt particles, Atmos. Chem. Phys., 17, 14039–14054, https://doi.org/10.5194/acp-17-14039-2017, 2017.
Lelieveld, J., Peters, W., Dentener, F. J., and Krol, M. C.: Stability of tropospheric hydroxyl chemistry, J. Geophys. Res., 107, ACH 17-1–ACH 17-11, https://doi.org/10.1029/2002JD002272, 2002.
Lenton, T. M., Held, H., Kriegler, E., Hall, J. W., Lucht, W., Rahmstorf, S., and Schellnhuber, H. J.: Tipping elements in the Earth's climate system, P. Natl. Acad. Sci. USA, 105, 1786–1793, https://doi.org/10.1073/pnas.0705414105, 2008.
Li, L., Mahowald, N. M., Miller, R. L., Pérez García-Pando, C., Klose, M., Hamilton, D. S., Gonçalves Ageitos, M., Ginoux, P., Balkanski, Y., Green, R. O., Kalashnikova, O., Kok, J. F., Obiso, V., Paynter, D., and Thompson, D. R.: Quantifying the range of the dust direct radiative effect due to source mineralogy uncertainty, Atmos. Chem. Phys., 21, 3973–4005, https://doi.org/10.5194/acp-21-3973-2021, 2021.
Li, Q., Fernandez, R. P., Hossaini, R., Iglesias-Suarez, F., Cuevas, C. A., Apel, E. C., Kinnison, D. E., Lamarque, J. F., and Saiz-Lopez, A.: Reactive halogens increase the global methane lifetime and radiative forcing in the 21st century, Nat. Commun., 13, 2768, https://doi.org/10.1038/s41467-022-30456-8, 2022.
Li, Q., Meidan, D., Hess, P., Anel, J. A., Cuevas, C. A., Doney, S., Fernandez, R. P., van Herpen, M., Hoglund-Isaksson, L., Johnson, M. S., Kinnison, D. E., Lamarque, J. F., Röckmann, T., Mahowald, N. M., and Saiz-Lopez, A.: Global environmental implications of atmospheric methane removal through chlorine-mediated chemistry-climate interactions, Nat. Commun., 14, 4045, https://doi.org/10.1038/s41467-023-39794-7, 2023.
Li, Y., Song, Y., Li, X., Kaskaoutis, D. G., Gholami, H., and Li, Y: Disentangling variations of dust concentration in Greenland ice cores over the last glaciation: An overview of current knowledge and new initiative, Earth-Sci. Rev., 242, 104451, https://doi.org/10.1016/j.earscirev.2023.104451, 2023.
Maesano, C. N., Campbell, J. S., Foteinis, S., Furey, V., Hawrot, O., Pike, D., Aeschlimann, S., Reginato, P. L., Goodwin, D. R., Looger, L. L., Boyden, E. S., and Renforth, P.: Geochemical Negative Emissions Technologies: Part II. Roadmap, Front. Clim., 4, 945332, https://doi.org/10.3389/fclim.2022.945332, 2022.
Mahowald, N. M., Hamilton, D. S., Mackey, K. R. M., Moore, J. K., Baker, A. R., Scanza, R. A., and Zhang, Y.: Aerosol trace metal leaching and impacts on marine microorganisms, Nat. Commun., 9, 2614, https://doi.org/10.1038/s41467-018-04970-7, 2018.
Mak, J., Kra, G., Sandomenico, T., and Bergamaschi, P.: The seasonality varying isotopic composition of the sources of carbon monoxide at Barbados, West Indies, J. Geophys. Res., 108, 4635, https://doi.org/10.1029/2003JD003419, 2003.
Matsui, H., Mahowald, N. M., Moteki, N., Hamilton, D. S., Ohata, S., Yoshida, A., Koike, M., Scanza, R. A., and Flanner, M. G.: Anthropogenic combustion iron as a complex climate forcer, Nat. Commun., 9, 1593, https://doi.org/10.1038/s41467-018-03997-0, 2018.
McKay, D. I. A., Stall, A., Abrams, J. F., Winkelmann, R., Sakschewski, B., Loriani, S., Fetzer, I., Cornell, S. E., Rockstrom, J., and Lenton, T. M.: Exceeding 1.5 °C global warming could trigger multiple climate tipping points, Science, 377, eabn7950, https://doi.org/10.1126/science.abn7950, 2022.
Meidan, D., Li, Q., Cuevas, C. A., Doney, S. C., Fernandez, R. P., van Herpen, M. M. J. W., Johnson, M. S., Kinnison, D. E., Li, L., and Hamilton, D. S.: Evaluating the potential of iron-based interventions in methane reduction and climate mitigation, Environ. Res. Lett., 19, 054023, https://doi.org/10.1088/1748-9326/ad3d72, 2024.
Ming, T., Li, W., Yuan, Q., Davies, P., de Richter, R., Peng, C., Deng, Q., Yuan, Y., Caillol, S., and Zhou, N.: Perspectives on removal of atmospheric methane, Advances in Applied Energy, 5, 100085, https://doi.org/10.1016/j.adapen.2022.100085, 2022.
Neumann, R. B., Moorberg, C. J., Lundquist, J. D., Turner, J. C., Waldrop, M. P., McFarland, J. W., Euskirchen, E. S., Edgar, C. W., and Turetsky, M. R.: Warming Effects of Spring Rainfall Increase Methane Emissions From Thawing Permafrost, Geophys. Res. Lett., 46, 1393–1401, https://doi.org/10.1029/2018GL081274, 2019.
Nisbet, E. G., Manning, M. R., Dlugokencky, E. J., Michel, S. E., Lan, X., Röckmann, T., Denier van der Gon, H. A. C., Schmitt, J., Palmer, P. I., Dyonisius, M. N., Oh, Y., Fisher, R. E., Lowry, D., France, J. L., White, J. W. C., Brailsford, G., and Bromley, T.: Atmospheric Methane: Comparison Between Methane's Record in 2006–2022 and During Glacial Terminations, Global Biogeochem. Cy., 37, e2023GB007875, https://doi.org/10.1029/2023GB007875, 2023.
NOAA: Trends in Atmospheric Methane, NOAA Global Monitoring Laboratory, Earth System Research Laboratories, https://gml.noaa.gov/ccgg/trends_ch4/, last access: 7 December 2023.
Ocean Visions: Ocean-Based Carbon Dioxide Removal: Road Maps, https://www2.oceanvisions.org/roadmaps/, last access: 7 December 2023.
O'Day, P. A., Pattammattel, A., Aronstein, P., Leppert, V. J., and Forman, H. J.: Iron Speciation in Respirable Particulate Matter and Implications for Human Health, Environ. Sci. Technol., 56, 7006–7016, https://doi.org/10.1021/acs.est.1c06962, 2022.
Oeste, F. D.: Method for cooling the troposphere, https://patentimages.storage.googleapis.com/ac/64/72/49f3c79762e44f/AU2010203265B2.pdf (last access: 7 December 2023), 2009.
Oeste, F. D., de Richter, R., Ming, T., and Caillol, S.: Climate engineering by mimicking natural dust climate control: the iron salt aerosol method, Earth Syst. Dynam., 8, 1–54, https://doi.org/10.5194/esd-8-1-2017, 2017.
Paudel, R., Mahowald, N. M., Hess, P. G. M., Meng, L., and Riley, W. J.: Attribution of changes in global wetland methane emissions from pre-industrial to present using CLM4.5-BGC, Environ. Res. Lett., 11, 034020, https://doi.org/10.1088/1748-9326/11/3/034020, 2016.
Pennacchio, L., van Herpen, M., Meidan, D., Saiz-Lopez, A., and Johnson, M. S.: Catalytic efficiencies for atmospheric methane removal in the high-chlorine regime, ChemRxiv [preprint], https://doi.org/10.26434/chemrxiv-2023-3r8sf, 12 October 2023.
Peng, S., Lin, X., Thompson, R.L., Xi, Y., Liu, G., Hauglustaine, D., Lan, X., Poulter, B., Ramonet, M., Saunois, M., Yin, Y., Zhang, Z., Zheng, B., and Ciais, P.: Wetland emission and atmospheric sink changes explain methane growth in 2020, Nature, 612, 477–482, https://doi.org/10.1038/s41586-022-05447-w, 2022.
Pio, C. A. and Lopes, D. A.: Chlorine loss from marine aerosol in a coastal atmosphere, J. Geophys. Res., 103, 25263–25272, https://doi.org/10.1029/98JD02088, 1998.
Prospero, J. M., Delany, A. C., Delany, A. C., and Carlson, T. N.: The Discovery of African Dust Transport to the Western Hemisphere and the Saharan Air Layer, B. Am. Meteorol. Soc., 102, E1239–E1260, https://doi.org/10.1175/BAMS-D-19-0309.1, 2021.
Pye, H. O. T., Nenes, A., Alexander, B., Ault, A. P., Barth, M. C., Clegg, S. L., Collett Jr., J. L., Fahey, K. M., Hennigan, C. J., Herrmann, H., Kanakidou, M., Kelly, J. T., Ku, I.-T., McNeill, V. F., Riemer, N., Schaefer, T., Shi, G., Tilgner, A., Walker, J. T., Wang, T., Weber, R., Xing, J., Zaveri, R. A., and Zuend, A.: The acidity of atmospheric particles and clouds, Atmos. Chem. Phys., 20, 4809–4888, https://doi.org/10.5194/acp-20-4809-2020, 2020.
Qu, Z., Jacob, D. J., Zhang, Y., Shen, L., Varon, D. J., Lu, X., Scarpelli, T., Bloom, A., Worden, J., and Parker, R. J.: Attribution of the 2020 surge in atmospheric methane by inverse analysis of GOSAT observations, Envion. Res. Lett., 17, 094003, https://doi.org/10.1088/1748-9326/ac8754, 2022.
Röckmann, T., Brenninkmeijer, C. A. M., Crutzen, P. J., and Platt, U.: Short term variations in the ratio of CO as a measure of Cl activation during tropospheric ozone depletion events in the Arctic, J. Geophys. Res., 104, 1691–1697, https://doi.org/10.1029/1998JD100020, 1999.
Rodriguez, S., Prospero, J. M., Lopez-Darias, J., Garcia-Alvarez, M. I., Zuidema, P., Nava, S., Lucarelli, F., Gaston, C. J., Galindo, L., and Sosa, E.: Tracking the changes of iron solubility and air pollutants traces as African dust transits the Atlantic in the Saharan dust outbreaks, Atmos. Environ., 246, 118092, https://doi.org/10.1016/j.atmosenv.2020.118092, 2021.
Saiz-Lopez, A., Fernandez, R. P., Li, Q., Cuevas, C. A., Fu, X., Kinnison, D. E., Times, S., Mahajan, A. S., Martin, J. C. G., Iglesias-Suerez, F., Hossaini, R., Plane, J. M. C., Myhre, G., and Lamarque, J. F.: Natural short-lived halogens exert an indirect cooling effect on climate, Nature, 618, 967–973, https://doi.org/10.1038/s41586-023-06119-z, 2023.
Sapart, C. J., Monteil, G., Prokopiou, M., van de Wal, R. S. W., Kaplan, J. O., Sperlich, P., Krumhardt, K. M., Van der Veen, C., Houweling, S., Krol, M. C., Blunier, T., Sowers, T., Martinerie, P., Witrant, E., Dahl-Jensen, D., and Röckmann, T.: Natural and anthropogenic variations in methane sources during the past two millennia, Nature, 490, 85–88, https://doi.org/10.1038/nature11461, 2012.
Saunois, M., Stavert, A. R., Poulter, B., Bousquet, P., Canadell, J. G., Jackson, R. B., Raymond, P. A., Dlugokencky, E. J., Houweling, S., Patra, P. K., Ciais, P., Arora, V. K., Bastviken, D., Bergamaschi, P., Blake, D. R., Brailsford, G., Bruhwiler, L., Carlson, K. M., Carrol, M., Castaldi, S., Chandra, N., Crevoisier, C., Crill, P. M., Covey, K., Curry, C. L., Etiope, G., Frankenberg, C., Gedney, N., Hegglin, M. I., Höglund-Isaksson, L., Hugelius, G., Ishizawa, M., Ito, A., Janssens-Maenhout, G., Jensen, K. M., Joos, F., Kleinen, T., Krummel, P. B., Langenfelds, R. L., Laruelle, G. G., Liu, L., Machida, T., Maksyutov, S., McDonald, K. C., McNorton, J., Miller, P. A., Melton, J. R., Morino, I., Müller, J., Murguia-Flores, F., Naik, V., Niwa, Y., Noce, S., O'Doherty, S., Parker, R. J., Peng, C., Peng, S., Peters, G. P., Prigent, C., Prinn, R., Ramonet, M., Regnier, P., Riley, W. J., Rosentreter, J. A., Segers, A., Simpson, I. J., Shi, H., Smith, S. J., Steele, L. P., Thornton, B. F., Tian, H., Tohjima, Y., Tubiello, F. N., Tsuruta, A., Viovy, N., Voulgarakis, A., Weber, T. S., van Weele, M., van der Werf, G. R., Weiss, R. F., Worthy, D., Wunch, D., Yin, Y., Yoshida, Y., Zhang, W., Zhang, Z., Zhao, Y., Zheng, B., Zhu, Q., Zhu, Q., and Zhuang, Q.: The Global Methane Budget 2000–2017, Earth Syst. Sci. Data, 12, 1561–1623, https://doi.org/10.5194/essd-12-1561-2020, 2020.
Seinfeld, J. H. and Pandis, S. N.: Atmospheric chemistry and physics: from air pollution to climate change, John Wiley & Sons, ISBN 0471720186, 2016.
Spark Climate Solutions: Approaches to Atmospheric Methane Removal, https://www.sparkclimate.org/methane-removal/101/approaches, last access: 7 December 2023.
Staniaszek, Z., Griffiths, P. T., Folberth, G. A., O'Connor, F. M., Abraham, N. L., and Archibald, A. T.: The role of future anthropogenic methane emission in air quality and climate, npj Clim. Atmos. Sci, 5, 21, https://doi.org/10.1038/s41612-022-00247-5, 2022.
The Arctic Institute: Sami Council resistance to SCoPEX highlights the complex questions surrounding geoengineering and consent, https://www.thearcticinstitute.org/sami-council-resistance- scopex-highlights-complex-questions-geoengineering-consent/ (last access: 7 December 2023), 2021.
UNEP (United Nations Environment Programme): Global Methane Assessment: Benefits and Costs of Mitigating Methane Emissions, https://www.unep.org/resources/report/global-methane- assessment-benefits-and-costs-mitigating-methane-emissions (last access: 7 December 2023), 2021.
van Herpen, M. M. J. W., Li, Q., Saiz-Lopez, A., Liisberg, J. B., Röckmann, T., Cuevas, C. A., Fernandez, R. P., Mak, J. E., Mahowald, N. M., Hess, P., Meidan, D., Stuut, J.-B. W., and Johnson, M. S.: Photocatalytic Chlorine Atom Production on Mineral Dust-Sea Spray Aerosols over North Atlantic, P. Natl. Acad. Sci. USA, 120, e2303974120, https://doi.org/10.1073/pnas.2303974120, 2023.
Wang, X., Jacob, D. J., Eastham, S. D., Sulprizio, M. P., Zhu, L., Chen, Q., Alexander, B., Sherwen, T., Evans, M. J., Lee, B. H., Haskins, J. D., Lopez-Hilfiker, F. D., Thornton, J. A., Huey, G. L., and Liao, H.: The role of chlorine in global tropospheric chemistry, Atmos. Chem. Phys., 19, 3981–4003, https://doi.org/10.5194/acp-19-3981-2019, 2019.
Wang, X., Jacob, D. J., Fu, X., Wang, T., Le Breton, M., Hallquist, M., Liu, Z., McDuffie, E. E., and Liao, H.: Effects of Anthropogenic Chlorine on PM2.5 and Ozone Air Quality in China, Environ. Sci. Technol., 54, 9908–9916, https://doi.org/10.1021/acs.est.0c02296, 2020.
Wanser, K., Doherty, S. J., Hurrell, J. W., and Wong, A.: Near-term climate risks and sunlight reflection modification: a roadmap approach for physical science research, Climatic Change, 174, 23, https://doi.org/10.1007/s10584-022-03446-4, 2022.
Wittmer, J. and Zetzsch, C.: Photochemical activation of chlorine by iron oxide aerosol, J. Atmos. Chem., 74, 187–204, https://doi.org/10.1007/s10874-016-9336-6, 2017.
Wittmer, J., Bleicher, S., Ofner, J., and Zetzsch, C.: Iron (III)-induced activation of chloride from artificial sea-salt aerosol, Environ. Chem., 12, 461–475, https://doi.org/10.1071/EN14279, 2015a.
Wittmer, J., Bleicher, S., and Zetzsch, C.: Iron (III)-induced activation of chloride and bromide from modeled salt pans, J. Phys. Chem. A, 119, 4373–4385, https://doi.org/10.1021/jp508006s, 2015b.
Yuan, T., Yu, H., Chin, M., Remer, L. A., McGee, D., and Evan, A.: Anthropogenic decline of African dust: Insights from the Holocene records and beyond, Geophys. Res. Lett., 47, e2020GL089711, https://doi.org/10.1029/2020GL089711, 2020.
Zhang, Z., Poulter, B., Feldman, A. F., Ying, Q., Ciais, P., Peng, S., and Li, X.: Recent intensification of wetland methane feedback, Nat. Clim. Change, 13, 430–433, https://doi.org/10.1038/s41558-023-01629-0, 2023.
Zhu, X. R., Prospero, J. M., and Millero, F. J.: Diel variability of soluble Fe(II) and soluble total Fe in North African dust in the trade winds at Barbados, J. Geophys. Res., 102, 21297–21305, https://doi.org/10.1029/97JD01313, 1997.