the Creative Commons Attribution 4.0 License.
the Creative Commons Attribution 4.0 License.
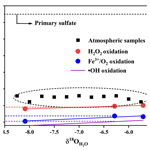
Quantifying SO2 oxidation pathways to atmospheric sulfate using stable sulfur and oxygen isotopes: laboratory simulation and field observation
Ziyan Guo
Pengxiang Qiu
Mingyi Xu
Zhaobing Guo
The formation of secondary sulfate in the atmosphere remains controversial, and it is an urgent need to seek a new method to quantify different sulfate formation pathways. Thus, SO2 and PM2.5 samples were collected from 4 to 22 December 2019 in the Nanjing region. Sulfur and oxygen isotopic compositions were synchronously measured to study the contribution of SO2 homogeneous and heterogeneous oxidation to sulfate. Meanwhile, the correlation of δ18O values between H2O and sulfate from SO2 oxidation by H2O2 and Fe3+ O2 was simulatively investigated in the laboratory. Based on isotope mass equilibrium equations, the ratios of different SO2 oxidation pathways were quantified. The results showed that secondary sulfate constituted higher than 80 % of total sulfate in PM2.5 during the sampling period. Laboratory simulation experiments indicated that the δ18O value of sulfate was linearly dependent on the δ18O value of water, and the slopes of linear curves for SO2 oxidation by H2O2 and Fe3+ O2 were 0.43 and 0.65, respectively. The secondary sulfate in PM2.5 was mainly ascribed to SO2 homogeneous oxidation by OH radicals and heterogeneous oxidation by H2O2 and Fe3+ O2. SO2 heterogeneous oxidation was generally dominant during sulfate formation, and SO2 oxidation by H2O2 predominated in SO2 heterogeneous oxidation reactions, with an average ratio around 54.6 %. This study provided an insight into precisely evaluating sulfate formation by combining stable sulfur and oxygen isotopes.
- Article
(1397 KB) - Full-text XML
- BibTeX
- EndNote
Sulfate is one of the prevalent components of PM2.5 (Brüggemann et al., 2021; Huang et al., 2014; Yang et al., 2023). Sulfate makes up approximately 25 % of PM2.5 mass in Shanghai, 23 % in Guangzhou, and 10 %–33 % in Beijing (Xue et al., 2016). The rapid sulfate formation is a crucial factor determining the explosive growth of fine particles and the frequent occurrence of severe haze events in China (Lin et al., 2022; Y. Y. Liu et al., 2020; Meng et al., 2023; Wang et al., 2021; Zhang et al., 2018). Sulfate plays an important role in the chemical and physical processes in the troposphere and lower stratosphere, and it significantly affects global climate change by scattering solar radiation and acting as cloud condensation nuclei (Gao et al., 2022; Ramanathan et al., 2001). Meanwhile, sulfate exerts a significant influence on air quality and public health (Abbatt et al., 2006).
In the past decades, numerous attempts have been made to evaluate SO2 oxidation pathways that are involved in homogeneous and heterogeneous reactions. Traditionally, sulfate formation mechanisms mainly include SO2 homogeneous oxidation by OH radicals and heterogeneous oxidation by H2O2, O3, and O2 catalysed by transition metal ions (TMIs) in cloud/fog water droplets. The relative importance of different sulfate formation pathways is strongly dependent on oxidant concentrations, the occurrence of fog/cloud events, and the pH of the aqueous phase (Kuang et al., 2022; Oh et al., 2023). Generally, SO2 homogeneous oxidation by OH radicals and heterogeneous oxidation by H2O2 are considered the most important pathways for sulfate production on the global scale (Seinfeld and Pandis, 1998). The photochemical reactivity during the winter in Beijing has been found to be relatively high, which favours the formation of reactive species such as OH radicals and H2O2, thereby facilitating SO2 oxidation (Zhang et al., 2020). Xue et al. (2014) suggested that SO2 oxidation by O3 and H2O2 in the aqueous phase contributed to the majority of total sulfate production. T. Liu et al. (2020) proposed that S(IV) oxidation by H2O2 in aerosol water could be an important pathway that considers the ionic strength effect. He et al. (2018) found that the contribution of SO2 oxidation by H2O2 could reach 88 % during the haze period in Beijing. Ye et al. (2018) observed that the SO2 oxidation rate by H2O2 was 2–5 times faster than the summed rate of the other three oxidation pathways. As a result, the actual contribution of SO2 oxidation by H2O2 during the winter might be underestimated in the previous studies.
In addition, the presence of NO2 is obviously favourable for SO2 oxidation under the conditions of high relative humidity (RH) and NH3. NH3 can promote the hydrolysis of NO2 dimers to HONO and result in more sulfate formation on the particle surface in humid conditions (He et al., 2021). However, this conclusion was doubted by Liu et al. (2017), who believed that the reaction on actual fine particles with the pH at 4.2 was too slow to account for sulfate formation. Li et al. (2020) deemed that SO2 oxidation by NO2 might not be a major oxidation pathway in China. Furthermore, a GEOS-Chem modelling study suggested that NO2 oxidation contributed less than 2 % of total sulfate production. It is found that the TMI pathway was very important in highly polluted regions, and the contribution of metal-catalysed SO2 oxidation to sulfate was as high as 49 ± 10 % in haze. Wang et al. (2021) also argued that SO2 oxidation via TMIs on the aerosol surface could be the dominant sulfate formation pathway. They found that manganese-catalysed oxidation of SO2 contributed 69.2 ± 5.0 % of sulfate production. Overall, the mechanisms for sulfate rapid growth remain unclear and controversial. Therefore, sulfate formation pathways need to be further explored, and it is urgent that a new method to quantify different sulfate formation processes is developed.
Generally, sulfur isotopes allow for investigating SO2 oxidation processes in the atmosphere because of distinctive isotope fractionation associated with different oxidation reactions (Harris et al., 2013). Harris et al. (2012) presented the respective sulfur isotope fractionation factors of SO2 oxidation by OH radicals, O3 H2O2, and iron catalysis. In addition, the observed sulfur isotope fractionation of SO2 oxidation by H2O2 and O3 appeared to have no significant difference. Therefore, the results were particularly useful to determine the importance of transition metal-catalysed oxidation pathway compared to other oxidation pathways. However, other main SO2 oxidation pathways could not be distinguished only based on stable sulfur isotope determination.
The oxygen isotope ratio (δ18O) can be used to deduce sulfate formation processes due to those SO2 oxidation pathways affecting oxygen isotopes of the sulfate product differently. In particular, mass-independent fractionation signals of oxygen isotopes (nonzero Δ17O, where Δ17O =δ18O O) in sulfate are usually adopted to investigate the contribution of different SO2 oxidation pathways. This method can identify the contribution of SO2+O3 pathway when a high Δ17O value (> 3 ‰) is measured in sulfate. However, there is obvious uncertainty when interpreting the sulfate with a low Δ17O value (< 1 ‰). Unfortunately, most sulfate samples in the atmosphere present Δ17O< 1 ‰, suggesting a limited contribution of the SO2+O3 pathway during sulfate formation. It is noteworthy that the contribution of SO2+ H2O2 and TMI pathway is unclear if solely using Δ17O (Li et al., 2020). Holt and Kumar (1984) found that the use of oxygen isotopes was a valuable and complementary method to determine probable mechanisms of SO2 oxidation to sulfate in the atmosphere. This provides us an insight into precisely evaluating sulfate formation pathways by combining oxygen and sulfur isotopes.
In this contribution, PM2.5 and SO2 were sampled from 4 to 22 December 2019 in Nanjing. Sulfur and oxygen isotopic compositions were measured to study the contribution of SO2 homogeneous and heterogeneous oxidation during sulfate formation. In addition, the linear relationships of δ18O values between H2O and sulfate from SO2 oxidation by H2O2 and Fe3+ O2 were synchronously investigated in the laboratory. Based on sulfur and oxygen isotope mass equilibrium equations, the ratios of different SO2 oxidation pathways during the sampling period were calculated. The study aims to seek a novel method to quantify different SO2 oxidation processes with sulfur and oxygen isotopes.
2.1 Sampling location
PM2.5 and SO2 in the atmosphere were sampled from 4 to 22 December 2019 in Nanjing, China. The sampling site was located on the roof of the library of Nanjing University of Information Science and Technology (NUIST; 32.1∘ N, 118.5∘ E), which is depicted in Fig. 1. The sampling location is on the side of Ningliu Road and close to Nanjing chemical industry park. There are some large-scale chemical enterprises present such as Nanjing steel plant, Nanjing thermal power plants, and Nanjing petrochemical company, which inevitably release lots of SO2 and iron metal into the atmosphere.
2.2 PM2.5 and SO2 samples collection
PM2.5 and SO2 were sampled using a modified JCH-1000 sampler (Juchuang Co., Qingdao) with a flow rate of 1.05 m3 min−1 from 08:00 to 20:00 from 4 to 22 December 2019. PM2.5 and SO2 were collected with a quartz filter (203 × 254 mm, Munktell, Sweden) and a glass fibre filter (203 × 254 mm, Tisch Environment INC, USA), respectively. The filters were incinerated in a muffle furnace at 450 ∘C for 2 h and then preserved in the desiccators at room temperature. The glass fibre filters were firstly soaked in 2 % K2CO3 and 2 % glycerol solution for 2 h and dried in a DGG-9070A electric oven. SO2 can be changed into sulfite immediately during the sampling.
2.3 Extractions of water-soluble sulfate
PM2.5 sample filters were shredded and soaked in 400 mL of Milli-Q (18 MΩ) water for extractions of water-soluble sulfate. Filters were then isolated from solutions by centrifugation, and sulfate was precipitated as BaSO4 by adding 1 mol L−1 BaCl2. After the filtration with 0.22 µm acetate membrane, BaSO4 precipitate was rinsed with Milli-Q water to remove Cl−. Finally, BaSO4 powers were calcined at 800 ∘C for 2 h to obtain high-purity BaSO4. In addition, a small amount of H2O2 solution was added to oxidize sulfite to sulfate.
2.4 Laboratory simulation of SO2 oxidation by H2O2 and Fe3+ O2
For SO2 oxidation by H2O2, 30 mL min−1 Ar was firstly introduced into three kinds of different water for about 30 min to drive out air. Sulfate was produced by adding 10 mL H2O2 dilute solution (0.1 mL 30 % H2O2 in 50 mL water) to SO2 in the reaction chamber at 10 ∘C. H2O2 solution was agitated vigorously for 1 min before admission of air. For SO2 oxidation by Fe3+ O2, 2 mL min−1 SO2 and 2 mL min−1 O2 were simultaneously put into the Fe3+ dilute solution at 10 ∘C. Then, 10 mL 1 mL min−1 BaCl2 was added to prepare BaSO4. Oxygen isotopic compositions of product sulfate and three kinds of water were measured to study their linear relationships.
2.5 Sulfur and oxygen isotope determination
Sulfur isotopic compositions in sulfate were analysed using an elemental analyser (EA; Flash 2000, Thermo) and isotope mass spectrometer (IRMS; Delta V Plus, Finningan). High-purity BaSO4 was converted into SO2 in EA in the presence of Cu2O. SO2 from EA was ionized, and the δ34S value was measured using the IRMS. For the determination of δ18O, BaSO4 pyrolysis was conducted in a graphite furnace at 1450 ∘C, and the δ18O value was obtained in CO produced from the pyrolysis at continuous-flow mode. The results of δ34S and δ18O were with respect to the international standards V-CDT and V-SMOW, and the accuracy was better than ± 0.2 ‰ and ± 0.3 ‰, respectively.
3.1 Concentrations of PM2.5, sulfate and SO2
As described in Fig. 2, the mass concentrations of PM2.5, SO, and SO2 during the period from 4 to 22 December 2019 in NUIST changed from 28.1 to 67.0 µg m−3, 8.3 to 17.8 µg m−3, and 6.2 to 20.9 µg m−3, with an average and standard deviation at 45.7 ± 12.1, 12.7± 3.3 and 10.2 ± 4.4 µg m−3, respectively. It can be observed that PM2.5 average concentration was about 1.3 times the First Grade National Ambient Air Quality Standard (35 µg m−3) and beyond the safety standard of the World Health Organization (10 µg m−3). The photochemical reactivity during the winter in Beijing has been found to be relatively high (Zhang et al., 2020), which facilitates the formation of some photooxidants. The relatively clean days during the sampling period indicate the importance of photoinduced oxidation of SO2.
Meanwhile, the change trends of PM2.5, SO, and SO2 concentrations were found to be basically the same during the sampling period, indicating that sulfate was mainly from SO2 oxidation. In particular, PM2.5, SO, and SO2 concentrations increased to the maximum values on 10 December. It is noted that NO2 and CO concentrations were 85 and 1.60 µg m−3 on 10 December, which were also the maximum values during the sampling period. Based on the wind speed being lower than 3 m s−1 and there being static weather during the sampling period, we believe that high CO concentration was mainly from local emissions. However, O3 concentration on 10 December had a minimum value at 24 µg m−3, which preliminarily indicated that SO2 oxidation by NO2 might be a major pathway in sulfate formation. Previous studies showed that SO2 oxidation by NO2 in aerosol water dominated heterogeneous sulfate formation during wintertime at neutral aerosol pH (Wang et al., 2016; Cheng et al., 2016). However, subsequent studies showed that the calculated aerosol pH was in the range of 4.2–4.7, and the reactions between SO2 and NO2 during this pH range were too slow to produce sulfate. Considering low aerosol pH in the Nanjing region, we suggested that SO2 oxidation by NO2 was not a dominant pathway for sulfate formation during the sampling period.
In contrast, PM2.5, SO, and SO2 concentrations were observed to be at minimum values on 6 December. Similarly, NO2 and CO concentrations were also at minimum values of 36 and 0.6 mg m−3, respectively. However, O3 concentration on 6 December had a maximum value at 50 µg m−3. In addition, the rate of SO2 oxidation with O3 becomes fast only when pH > 5, and the reaction rate of SO2 with O3 is one-hundredth of that with H2O2 or TMIs when pH < 5. Therefore, pH values of actual fine particles at 4–5 in the Nanjing region could markedly restrain SO2 oxidation by O3. The lowest SO concentration on 6 December further demonstrated that SO2 oxidation by O3 played an insignificant role in sulfate formation.
Generally, aqueous-phase oxidation is deemed to be a main process of sulfate formation in atmospheric environment. Shao et al. (2018) believed that heterogeneous sulfate production on aerosols occurred when RH was higher than 50 %. The RH values of the atmosphere ranging from 50.7 % to 88.9 % during the sampling period indicated that sulfate formation was closely related to SO2 heterogeneous oxidation.
3.2 Sulfur isotopic compositions in sulfate and SO2
It can be observed from Fig. 3 that the values of δ34S-SO were generally higher compared to those of δ34S-SO2 during the sampling period except on 16 December. The δ34S-SO values ranged from 3.1 ‰ to 4.7 ‰, with an average and standard deviation at 4.0 ± 0.6 ‰, while δ34S-SO2 values changed from −2.9 ‰ to 4.7 ‰, with an average and standard deviation at −0.2 ± 2.3 ‰. The discrepancy between the values of δ34S-SO and δ34S-SO2 was mainly related to the sulfur isotope fractionation effect during SO2 oxidation to secondary sulfate.
It is noteworthy that δ34S-SO values were similar to those in PM2.5, with an average at 4.2 ‰ during the Youth Olympic Games in August 2014 in Nanjing (Guo et al., 2016). However, the average value of δ34S-SO during the sampling period was lower than 5.6 ‰ in Nanjing during a typical haze event from 21 December 2015 to 1 January 2016 (Guo et al., 2019). The higher δ34S values of sulfate in haze were possibly ascribed to SO2 heterogeneous oxidation, which typically enriched heavy sulfur isotope in sulfate. In this study, the average concentrations of PM2.5 were 45.7 µg m−3, indicating a time interval that is not heavily polluted. In addition, the relatively high temperature during the sampling period was favourable for photochemical reactions and OH radicals' formation. As a result, the contribution of SO2 homogenous oxidation increased during sulfate formation, which enriched light sulfur isotope compared to that in haze. Han et al. (2017) determined δ34S values in Beijing PM2.5, with an average at 6.0 ‰. It is observed that there was a regional difference in δ34S-SO values. The δ34S-SO value in Nanjing was generally lower than that in Beijing. The discrepancy of δ34S-SO value illustrated different sulfur sources and SO2 oxidation pathways in these regions. In addition, δ34S-SO values presented a seasonal change. δ34S values in Beijing aerosol sulfate varied from 3.4 ‰ to 7.0 ‰, with an average of 5.0 ‰ in summer, and from 7.1 ‰ to 11.3 ‰, with an average of 8.6 ‰ in winter. Generally, SO2 homogeneous oxidation dominated in summer compared to that in winter due to strong solar irradiation (Han et al., 2016). SO2 oxidation might lead to sulfur isotope fractionation, which was mainly attributed to equilibrium or kinetic discrimination between SO2 and sulfate. The influence of different oxidants on sulfur isotope fractionation needed to be further investigated.
Figure 4 presents the relationship between the δ34S-SO value and atmospheric temperature during the sampling period. It can be observed that there was an obviously negative correlation. The higher temperature generally corresponded to the lower δ34S-SO value. This is mainly ascribed to the kinetic effect of sulfur isotope fractionation during SO2 oxidation. At high temperature, more OH radicals were produced, and the contribution of SO2 homogeneous oxidation increased. It is reported that sulfur isotope fractionation about SO2 was −9 ‰ for homogeneous oxidation process (Tanaka et al., 1994). Therefore, a low δ34S value in sulfate at high temperature was chiefly due to the elevated SO2 homogeneous oxidation.
3.3 Sulfur isotope fractionation during SO2 oxidation
The secondary sulfate was generally from SO2 homogeneous and heterogeneous oxidation (Seinfeld and Pandis, 1998). The homogeneous and heterogeneous oxidation of SO2 might lead to sulfur isotope fractionation, which is described using the fractionation coefficient: (α)
Sulfate enriched heavy sulfur isotope (α>1) during SO2 heterogeneous oxidation for the presence of isotope equilibrium fractionation and kinetic fractionation. However, the light sulfur isotope enriched by sulfate (α<1) during SO2 homogeneous oxidation due to this process was only related to kinetic fractionation. As described in Fig. 5, α values ranged from 0.9988 to 1.0201, indicating there was SO2 homogeneous and heterogeneous oxidation during the sampling period. The α value was at a minimum of 0.9988 on 16 December, which showed SO2 homogeneous oxidation played a crucial role.
It is reported that the sulfur isotope fractionation during SO2 heterogeneous and homogeneous oxidation to sulfate was 16.5 ‰ and −9 ‰, respectively (Tanaka et al., 1994). Consequently, the contribution of SO2 heterogeneous and homogeneous oxidation to sulfate could be calculated by sulfur isotope mass equilibrium in Eqs. (2) and (3).
where x and y represent the contribution of SO2 heterogeneous and homogeneous oxidation, respectively.
It is observed from Fig. 6 that most of the days (7 out of 11) had more than 50 % contributions from SO2 heterogeneous oxidation, which indicated that SO2 heterogeneous oxidation was generally dominant during sulfate formation. He et al. (2018) presented the observations of oxygen-17 excess of PM2.5 sulfate collected in Beijing haze from October 2014 to January 2015 and found the contribution of heterogeneous sulfate production was about 41 %–54 % with a mean of 48 ± 5 %. The contribution of SO2 heterogeneous oxidation reached a high level during 5–7 December and on 19 December, which was closely related to the temperature of the atmosphere. The low temperature of about 5 ∘C during these days was favourable for SO2 dissolution in water and further oxidized to sulfate. On 16 December, the contribution of SO2 heterogeneous oxidation was at a minimum of 31.4 %. The highest temperature of 15 ∘C on 16 December restrained SO2 solubility in the aqueous solution and facilitated production of lots of gaseous oxidants such as OH radicals to promote SO2 homogeneous oxidation.
Overall, the temperature was an important factor in controlling SO2 oxidation pathways. High temperature facilitated kinetic fractionation of sulfur isotope during SO2 oxidation to sulfate, thereby decreasing the δ34S value in sulfate. In addition, there was a lack of positive correlation between the contribution of SO2 heterogeneous oxidation and O3 or NO2 concentration. This further demonstrated that SO2 oxidation by O3 and NO2 was not an important pathway during the sampling period. Consequently, we mainly focused on SO2 heterogeneous oxidation by H2O2 and Fe3+ O2 in the following study.
3.4 The correlation of δ18O values between H2O and SO from SO2 oxidation by H2O2 and Fe3+ O2
It is known that SO2 rapidly equilibrates with ambient water for very high molar ratios of H2O to SO2 in the atmosphere. As a result, the δ18O value of SO2 is dynamically controlled by the δ18O value of water, and the δ18O value of SO2 has no obvious effect on the δ18O value of sulfate produced from different oxidation pathways. Meanwhile, sulfate is very stable with respect to O atom exchange with ambient water. Consequently, δ18O can be adopted to distinguish SO2 oxidation processes due to the δ18O value of product sulfate reflecting the distinctive signals of different oxidants.
We simulatively studied SO2 heterogeneous oxidation by H2O2 and Fe3+ O2 in the laboratory, which aims to clarify the relationship of δ18O values between product sulfate and three kinds of water at 10 ∘C. It can be observed from Fig. 7 that the δ18O value of sulfate was linearly dependent on the δ18O value of water, and the slope of linear curve for H2O2 oxidation approximates a ratio of 0.43, indicating that the isotopy of about two of four oxygen atoms in sulfate was controlled by the δ18O value of water. The other two oxygen atoms were from H2O2 molecules, whose O–O bond remained intact during SO2 oxidation. In addition, we noted from Fig. 7 that the slope of the linear curve for Fe3+ O2 oxidation was about 0.65, which represented that the isotopy of about three of four oxygen atoms in sulfate was related to the δ18O value of water. A control of sulfate oxygens by water is also characteristic of heterogeneous oxidation mechanisms in which HSO isotopically equilibrated with water prior to significant oxidation to SO. The other one oxygen atom in sulfate was from O2. The higher slope suggested a higher dependence of the δ18O value of sulfate on the δ18O value of water during SO2 heterogeneous oxidation by Fe3+ O2. The discrepancy of the slopes for different SO2 heterogeneous oxidation processes provides us with a potential method to distinguish SO2 oxidation pathways.
3.5 δ18O-SO values in PM2.5 and SO2 main oxidation pathways
As depicted in Fig. 8, δ18O values of sulfate in PM2.5 ranged from 11.09 ‰ to 12.93 ‰, with an average and standard deviation of 12.35 ± 0.68 ‰. δ18O values of sulfate focused on a narrow scope, except those on 5 and 22 December. It should be pointed out that the δ18O value of secondary sulfate was a comprehensive result from different SO2 oxidation processes. Sulfate in PM2.5 usually consisted of primary sulfate and secondary sulfate. The δ18O value of primary sulfate is about 38 ‰ (Holt and Kumar, 1984), which is significantly higher than the values of secondary sulfate. The contribution of primary and secondary sulfate in the atmosphere can be calculated by the oxygen isotope mass equilibrium in Eq. (4) (Ben et al., 1982):
where δ18O, δ18OPS, and δ18OSS mean δ18O values of PM2.5, primary sulfate, and secondary sulfate, respectively, and fSS is the contribution of secondary sulfate in PM2.5.
It is noteworthy from Fig. 9 that there is a linear relationship between δ18O values in water and secondary sulfate from different SO2 oxidation pathways, and this can be described by Eqs. (5)–(7), where the value of δ18Owater is about −6.2 ‰ in the Nanjing region. As discussed above, secondary sulfate was mainly ascribed to SO2 homogeneous oxidation by OH radicals and heterogeneous oxidation by H2O2 and Fe3+ O2. Therefore, the δ18OSS value in Eq. (4) can be obtained based on Eqs. (5)–(7), respectively. As a result, the average contribution of primary and secondary sulfate in PM2.5 is presented in Table 1. It can be observed that the majority of sulfate in PM2.5 was secondary sulfate, which appears to constitute from 79.9 % to 86.2 % of total sulfate during the sampling period. It is admirable to quantitatively describe these formation pathways of secondary sulfate in PM2.5.
According to the percentages of SO2 heterogeneous and homogeneous oxidation to sulfate in Fig. 6 and the average contributions of primary sulfate and secondary sulfate in PM2.5 in Table 1, we can further calculate the ratios of different SO2 oxidation pathways at 10 ∘C via the oxygen isotope mass equilibrium in Eqs. (8)–(10), and the corresponding results are depicted in Table 2.
where δ18O and δ18OPS are δ18O values of total sulfate and primary sulfate in PM2.5; δ18OSS−OH, δ18O and δ18O are δ18O values of secondary sulfate from SO2 oxidation by OH radicals, Fe3+ O2, and H2O2, respectively; fPS and fSS are the contribution of primary and secondary sulfate; and fSS−OH, , and are the ratios of secondary sulfate from SO2 oxidation by OH radicals, Fe3+ O2, and H2O2, respectively.
Unlike heavily polluted days with reduced solar irradiation, the photochemical reactivity can remain high in clean days during the observation period because of relatively intense solar irradiation. As a result, some photochemical reactive species such as OH radicals and H2O2 are deemed to be the major oxidants for sulfate formation. Generally, H2O2 production in the relatively clean atmosphere is ascribed to self-reaction of HO2 radicals that mainly comes from the reactions of OH radicals with CO and volatile organic compounds. It is observed from Table 2 that the ratios of SO2 oxidation by OH radicals ranged from 38 % to 68 %, with an average and standard deviation at 48 ± 9.7 %. The ratio reached the maximum of 68 % on 16 December, which is mainly ascribed to the highest temperature of 15 ∘C during the sampling period. The photochemical reactions are favourable for producing more OH radicals. In contrast, the ratio of SO2 oxidation by OH radicals decreased to the minimum of 38 % on 6 December due to the low temperature.
It is known that SO2 oxidation by H2O2 and Fe3+ O2 is the most important pathway during SO2 heterogeneous oxidation. It can be observed from Table 2 that the percentage of sulfate from SO2 oxidation by H2O2 in secondary sulfate from SO2 heterogeneous oxidation changed from 38.7 % to 81.2 %, with an average and standard deviation at 54.6 ± 15.7 %, indicating that SO2 oxidation by H2O2 predominated during SO2 heterogeneous oxidation. In addition, there was an obviously positive correlation between the ratios of SO2 oxidation by H2O2 and OH radicals, which was chiefly attributed to the photochemical reactions. The relatively strong solar irradiation on 16 December resulted in the maximum ratio of 81.2 % for H2O2 oxidation in SO2 heterogeneous reactions. The sampling site is close to Nanjing steel plant. As companion emitters, Fe3+ is present in much higher concentrations than that in other areas. It is believed that SO2 oxidation by O2 in the presence of Fe3+ was not negligent in the areas where the concentrations of SO2 and Fe3+ were high. This inevitably resulted in the high SO2 oxidation ratio by Fe3+ O2 in SO2 heterogeneous oxidation processes.
There was no serious PM2.5 pollution during the sampling period. The secondary sulfate constitutes from 79.9 % to 86.2 % of total sulfate in PM2.5. SO2 oxidation by O3 and NO2 played an insignificant role in sulfate formation. The secondary sulfate was mainly ascribed to SO2 homogeneous oxidation by OH radicals and heterogeneous oxidation by H2O2 and Fe3+ O2. Compared to homogeneous oxidation, SO2 heterogeneous oxidation was generally dominant, with an average contribution of 51.6 %. SO2 oxidation by H2O2 predominated in SO2 heterogeneous oxidation reactions, the average ratio of which reached 54.6 %. Consequently, sulfur and oxygen isotopes can be used to gain an insight into sulfate formation. The determination of sulfur isotopic compositions in SO2 and sulfate is advantageous in quantifying the contribution of SO2 homogeneous and heterogeneous oxidation. Combining field observations of oxygen isotope in the atmosphere with the linear relationships of δ18O values between H2O and sulfate from different SO2 oxidation processes can obtain an increased understanding of specific sulfate formation pathways. This study is favourable for deeply investigating the sulfur cycle in the atmosphere.
Data supporting this paper are available upon request from the corresponding author (guocumt@nuist.edu.cn).
ZiG carried out the experiment and wrote the original draft. KL designed the methodology and administrated the project. PQ and MX performed the data collection. ZhG instructed the experiment and revised the paper.
The contact author has declared that none of the authors has any competing interests.
Publisher’s note: Copernicus Publications remains neutral with regard to jurisdictional claims made in the text, published maps, institutional affiliations, or any other geographical representation in this paper. While Copernicus Publications makes every effort to include appropriate place names, the final responsibility lies with the authors.
We gratefully acknowledge the financial support from the National Natural Science Foundation of China (nos. 41873016, 51908294, and 21976006) and the National Science Fund for Distinguished Young Scholars (no. 22325601).
This research has been supported by the National Natural Science Foundation of China (grant nos. 41873016, 51908294, and 21976006) and the National Science Fund for Distinguished Young Scholars (grant no. 22325601).
This paper was edited by Zhibin Wang and reviewed by two anonymous referees.
Abbatt, J. P. D., Benz, S., Cziczo, D. J., Kanji, Z., Lohmann, U., and Mohler, O.: Solid ammonium sulfate aerosols as ice nuclei: a pathway for cirrus cloud formation, Science, 313, 1770–1773, https://doi.org/10.1126/science.1129726, 2006.
Ben, D. H., Romesh, K., and Paul, T. C.: Primary Sulfates in Atmospheric Sulfates: Estimation by Oxygen Isotope Ratio Measurements, Science, 217, 51–53, https://doi.org/10.1126/science.217.4554.51, 1982.
Brüggemann, M., Riva, M., Perrier, S., Poulain, L., George, C., and Herrmann, H.: Overestimation of Monoterpene Organosulfate Abundance in Aerosol Particles by Sampling in the Presence of SO2, Environ. Sci. Technol. Lett., 8, 206–211, https://doi.org/10.1021/acs.estlett.0c00814, 2021.
Cheng, Y. F., Zheng, G. J., Wei, C., Mu, Q., Zheng, B., Wang, Z. B., Gao, M., Zhang, Q., He, K. B., Carmichael, G., Pöschl, U., and Su, H.: Reactive Nitrogen Chemistry in Aerosol Water as a Source of Sulfate during Haze Events in China, Sci. Adv., 2, e1601530, https://doi.org/10.1126/sciadv.1601530, 2016.
Gao, J., Wei, Y., Zhao, H., Liang, D., Feng, Y., and Shi, G.: The role of source emissions in sulfate formation pathways based on chemical thermodynamics and kinetics model, Sci. Total. Environ., 851, 158104, https://doi.org/10.1016/j.scitotenv.2022.158104, 2022.
Guo, Z. B., Shi, L., Chen, S. L., Jiang, W. J., Wei, Y., Rui, M. L., and Zeng, G.: Sulfur isotopic fractionation and source apportionment of PM2.5 in Nanjing region around the second session of the Youth Olympic Games, Atmos. Res., 174/175, 9–17, https://doi.org/10.1016/j.atmosres.2016.01.011, 2016.
Guo, Z. Y., Guo, Q. J., Chen, S. L., Zhu, B., Zhang, Y., Yu, J., and Guo, Z. B.: Study on pollution behavior and sulfate formation during the typical haze event in Nanjing with water soluble inorganic ions and sulfur isotopes, Atmos. Res., 217, 198–207, https://doi.org/10.1016/j.atmosres.2018.11.009, 2019.
Han, X. K., Guo, Q. J., Liu, C. Q., Fu, P. Q., Strauss, H., Yang, J., Jian, H., Wei, L., Hong, R., Peters, M., Wei, R. F., and Tian, L.: Using stable isotopes to trace sources and formation processes of sulfate aerosols from Beijing, China, Sci. Rep., 6, 29958, https://doi.org/10.1038/srep29958, 2016.
Han, X. K., Guo, Q. J., Strauss, H., Liu, C. Q., Hu, J., Guo, Z. B., Wei, R. F., Peters, M., Tian, L., and Kong, J.: Multiple Sulfur Isotope Constraints on Sources and Formation Processes of Sulfate in Beijing PM2.5 Aerosol, Environ. Sci. Technol., 51, 7794–7803, https://doi.org/10.1021/acs.est.7b00280, 2017.
Harris, E., Sinha, B., Hoppe, P., and Ono, S.: High-precision measurements of 33S and 34S fractionation during SO2 oxidation reveal causes of seasonality in SO2 and sulfate isotopic composition, Environ. Sci. Technol., 47, 12174–12183, https://doi.org/10.1021/es402824c, 2013.
Harris, E., Sinha, B., Hoppe, P., Crowley, J. N., Ono, S., and Foley, S.: Sulfur isotope fractionation during oxidation of sulfur dioxide: gas-phase oxidation by OH radicals and aqueous oxidation by H2O2, O3 and iron catalysis, Atmos. Chem. Phys., 12, 407–424, https://doi.org/10.5194/acp-12-407-2012, 2012.
He, P. Z., Alexander, B., Geng, L., Chi, X. Y., Fan, S. D., Zhan, H. C., Kang, H., Zheng, G. J., Cheng, Y. F., Su, H., Liu, C., and Xie, Z. Q.: Isotopic constraints on heterogeneous sulfate production in Beijing haze, Atmos. Chem. Phys., 18, 5515–5528, https://doi.org/10.5194/acp-18-5515-2018, 2018.
He, X., Wu, J. J., Ma, Z. C., Xi, X., and Zhang, Y. H.: NH3-promoted heterogeneous reaction of SO2 to sulfate on α-Fe2O3 particles with coexistence of NO2 under different relative humidities, Atmos. Environ., 262, 118622, https://doi.org/10.1016/j.atmosenv.2021.118622, 2021.
Holt, B. D. and Kumar, R.: Oxygen-18 study of high-temperature air oxidation of SO2, Atmos. Environ., 18, 2089–2094, https://doi.org/10.1016/0004-6981(84)90194-X, 1984.
Huang, R. J., Zhang, Y., Bozzetti, C., Ho, K. F., Cao, J. J., Han,Y., Daellenbach, K. R., Slowik, J. G., Platt, S. M., Canonaco, F., Zotter, P., Wolf, R., Pieber, S. M., Bruns, E. A., Crippa, M., Ciarelli, G., Piazzalunga, A., Schwikowski, M., Abbaszade, G., Schnelle-Kreis, J., Zimmermann, R., An, Z., Szidat, S., Baltensperger, U., El Haddad, I., and Prevot, A. S.: High secondary aerosol contribution to particulate pollution during haze events in China, Nature, 514, 218–222, https://doi.org/10.1038/nature13774, 2014.
Kuang, B., Zhang, F., Shen, J., Shen, Y., Qu, F., Jin, L., Tang, Q., Tian, X., and Wang, Z.: Chemical characterization, formation mechanisms and source apportionment of PM2.5 in north Zhejiang Province: The importance of secondary formation and vehicle emission, Sci. Total. Environ., 851, 158206, https://doi.org/10.1016/j.scitotenv.2022.158206, 2022.
Li, J. H. Y., Zhang, Y. L., Cao, F., Zhang, W., and Michalski, G.: Stable Sulfur Isotopes Revealed a Major Role of Transition-Metal Ion-Catalyzed SO2 Oxidation in Haze Episodes, Environ. Sci. Technol., 54, 2626–2634, https://doi.org/10.1021/acs.est.9b07150, 2020.
Lin, Y. C., Yu, M., Xie, F., and Zhang, Y.: Anthropogenic Emission Sources of Sulfate Aerosols in Hangzhou, East China: Insights from Isotope Techniques with Consideration of Fractionation Effects between Gas-to-Particle Transformations, Environ. Sci. Technol., 56, 3905–3914, https://doi.org/10.1021/acs.est.1c05823, 2022.
Liu, M. X., Song, Y., Zhou, T., Xu, Z. Y., Yan, C. Q., Zheng, M., Wu, Z. J., Hu, M., Wu, Y. S., and Zhu, T.: Fine particle pH during severe haze episodes in northern China, Geophys. Res. Lett., 44, 5213–5221, https://doi.org/10.1002/2017GL073210, 2017.
Liu, T., Clegg, S. L., and Abbatt, J. P. D.: Fast oxidation of sulfur dioxide by hydrogen peroxide in deliquesced aerosol particles, P. Natl Acad. Sci. USA., 117, 1354–1359, https://doi.org/10.1073/pnas.1916401117, 2020.
Liu, Y. Y., Wang, T., Fang, X. Z., Deng, Y., Cheng, H. Y., Nabi, I., and Zhang, L.: Brown carbon: An underlying driving force for rapid atmospheric sulfate formation and haze event, Sci. Total. Environ., 734, 139415, https://doi.org/10.1016/j.scitotenv.2020.139415, 2020.
Meng, X., Hang, Y., Lin, X., Li, T. T., Wang, T. J., Cao, J. J., Fu, Q. Y., Dey, S., Huang, K., Liang, F. C., Kan, H. D., Shi, X. M., and Liu, Y.: A satellite-driven model to estimate long-term particulate sulfate levels and attributable mortality burden in China, Environ. Int., 171, 107740, https://doi.org/10.1016/j.envint.2023.107740, 2023.
Oh, S. H., Park, K., Park, M., Song, M., Jang, K. S., Schauer, J. J., Bae, G. N., and Bae, M. S.: Comparison of the sources and oxidative potential of PM2.5 during winter time in large cities in China and South Korea, Sci. Total. Environ., 859, 160369, https://doi.org/10.1016/j.scitotenv.2022.160369, 2023.
Ramanathan, V., Crutzen, P. J., Kiehl, J. T., and Rosenfeld, D.: Aerosols, climate, and the hydrological cycle, Science, 294, 2119–2124, https://doi.org/10.1126/science.1064034, 2001.
Seinfeld, J. H. and Pandis, S. N.: Atmospheric Chemistry and Physics: From Air Pollution to Climate Change, Phys. Today, 51, 88–90, https://doi.org/10.1063/1.882420, 1998.
Shao, J. Y., Chen, Q. J., Wang, Y. X., Lu, X., He, P. Z., Sun, Y. L., Shah, V., Martin, R. V., Philip, S., Song, S. J., Zhao, Y., Xie, Z. Q., Zhang, L., and Alexander, B.: Heterogeneous sulfate aerosol formation mechanisms during wintertime Chinese haze events: air quality model assessment using observations of sulfate oxygen isotopes in Beijing, Atmos. Chem. Phys., 19, 6107–6123, https://doi.org/10.5194/acp-19-6107-2019, 2019.
Tanaka, N., Rye, D. M., Xiao, Y., and Lassaga, A. C.: Use of stable sulfur isotope systematic for evaluating oxidation reaction pathways and in-cloud scavenging of sulfur dioxide in the atmosphere, Geophys. Res. Lett., 21, 1519–1522, https://doi.org/10.1029/94GL00893, 1994.
Wang, G. H., Zhang, R. Y., Gomez, M. E., Yang, L. X., Levy Zamora, M., Hu, M., Lin, Y., Peng, J. F., Guo, S., Meng, J. J., Li, J. J., Cheng, C. L., Hu, T. F., Ren, Y. Q., Wang, Y. S., Gao, J., Cao, J. J., An, Z. S., Zhou, W. J., Li, G. H., Wang, J. Y., Tian, P. F., Marrero-Ortiz, W., Secrest, J., Du, Z. F., Zheng, J., Shang, D. J., Zeng, L. M., Shao, M., Wang, W. G., Huang, Y., Wang, Y., Zhu, Y. J., Li, Y. X., Hu, J. X., Pan, B., Cai, L., Cheng, Y. T., Ji, Y. M., Zhang, F., Rosenfeld, D., Liss, P. S., Duce, R. A., Kolb, C. E., and Molina, M. J.: Persistent sulfate formation from London Fog to Chinese haze, P. Natl. Acad. Sci. USA, 113, 13630–13635, https://doi.org/10.1073/pnas.1616540113, 2016.
Wang, W. G., Liu, M. Y., Wang, T. T., Song, Y., Zhou, L., Cao, J. J., Zhu, T., Tian, H. Z., and Ge, M. F.: Sulfate formation is dominated by manganese-catalyzed oxidation of SO2 on aerosol surfaces during haze events, Nat. Commun., 12, 1993, https://doi.org/10.1038/s41467-021-22091-6, 2021.
Xue, J., Yuan, Z., Yu, J. Z., and Lau, A. K. H.: An Observation-Based Model for Secondary Inorganic Aerosols, Aerosol Air Qual. Res., 14, 862–878, https://doi.org/10.4209/aaqr.2013.06.0188, 2014.
Xue, J., Yuan, Z., Griffith, S. M., Yu, X., Lau, A. K. H., and Yu, J. Z.: Sulfate Formation Enhanced by a Cocktail of High NOx, SO2, Particulate Matter, and Droplet pH during Haze-Fog Events in Megacities in China: An Observation-Based Modeling Investigation, Environ. Sci. Technol., 50, 7325–7334, https://doi.org/10.1021/acs.est.6b00768, 2016.
Yang, T., Xu, Y., Ye, Q., Ma, Y. J., Wang, Y. C., Yu, J. Z., Duan, Y. S., Li, C. X., Xiao, H. W., Li, Z. Y., Zhao, Y., and Xiao, H. Y.: Spatial and diurnal variations of aerosol organosulfates in summertime Shanghai, China: potential influence of photochemical processes and anthropogenic sulfate pollution, Atmos. Chem. Phys., 23, 13433–13450, https://doi.org/10.5194/acp-23-13433-2023, 2023.
Ye, C., Liu, P. F., Ma, Z. B., Xue, C. Y., Zhang, C. L., Zhang, Y. Y., Liu, J. F., Liu, C. T., Sun, X., and Mu, Y. J.: High H2O2 Concentrations Observed during Haze Periods during the Winter in Beijing: Importance of H2O2 Oxidation in Sulfate Formation, Environ. Sci. Technol. Lett., 5, 757–763, https://doi.org/10.1021/acs.estlett.8b00579, 2018.
Zhang, R., Sun, X. S., Shi, A. J., Huang, Y. H., Yan, J., Nie, T., Yan, X., and Li, X.: Secondary inorganic aerosols formation during haze episodes at an urban site in Beijing, China, Atmos. Environ., 177, 275–282, https://doi.org/10.1016/j.jes.2022.01.008, 2018.
Zhang, Y., Bao, F. X., Li, M., Xia, H. L., Huang, D., Chen C. C., and Zhao, J. C.: Photoinduced Uptake and Oxidation of SO2 on Beijing Urban PM2.5, Environ. Sci. Technol., 54, 14868–14876, https://doi.org/10.1021/acs.est.0c01532, 2020.