the Creative Commons Attribution 4.0 License.
the Creative Commons Attribution 4.0 License.
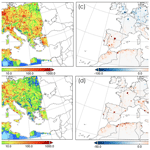
Isolating the climate change impacts on air-pollution-related-pathologies over central and southern Europe – a modelling approach on cases and costs
Patricia Tarín-Carrasco
María Morales-Suárez-Varela
Jørgen Brandt
Laura Palacios-Peña
Pedro Jiménez-Guerrero
Air pollution has important implications for human health and associated external costs to society and is closely related to climate change. This contribution tries to assess the impacts of present (1996–2015) and future (2071–2100 under RCP8.5) air pollution on several cardiovascular and respiratory pathologies and estimate the difference in the costs associated with these health impacts on the European population. For this, air quality data from the regional chemistry–climate modelling system of the Weather Research and Forecasting (WRF) model coupled with Chemistry (WRF-Chem) are used, together with some epidemiological information from the European Commission. The methodology considered relies on the Economic Valuation of Air Pollution (EVA) exposure–response functions and economic valuations (Brandt et al., 2013a, b). Several hypotheses have been established, in order to strictly isolate the effects of climate change on air pollution and health: constant present-day emission levels and population density in the whole of Europe. In general, the number of cases for the pathologies considered will increase in the future (chronic bronchitis, heart failure, lung cancer, premature deaths), increasing the overall cost associated from EUR 173 billion per year to over EUR 204 billion per year at the end of the present century. Premature deaths are the most important problem in the target area in terms of costs (EUR 158 billion per year, increasing by 17 % in the future RCP8.5 2071–2100 projection) and cases (418 700 cases per year, increasing by 94 900 cases per year in the future). The most affected areas are European megacities, the Ruhr Valley and several cities in eastern Europe (e.g. Chişinău, Bucharest). For the RCP8.5 scenario, cases and costs will increase over southern and eastern Europe, while central and northern Europe could benefit from climate change variations (decreasing both cases and costs for the studied pathologies).
- Article
(14563 KB) - Full-text XML
- BibTeX
- EndNote
Currently, air pollution is a serious environmental concern with a severe impact on population: on the one hand, by its close relationship with climate change, and on the other hand, because of its effects on human health and welfare. Air pollution is an environmental problem affecting the entire planet, either by local or transboundary pollution (Ravishankara et al., 2012). In 2012, 3.7 million premature deaths were caused by exposure to air pollution worldwide (WHO, 2013). In addition, indirectly, air pollution has external costs to society related to damage to human health. For this reason, the control of emissions of atmospheric pollutants and having reliable future air quality estimations can represent a good strategy for mitigating air-pollution-related pathologies (Lelieveld et al., 2015). However, European targets for emissions and air pollution are not being reached in southern Europe not only because of anthropic emissions but also because of natural causes (Pozzer et al., 2012), the Mediterranean Basin being the most affected area in terms of increases in air pollution in present and future climate scenarios (Colette et al., 2012; Jiménez-Guerrero et al., 2013a).
Quantifying premature deaths caused by air pollution is difficult for several reasons: first, the lack of monitoring stations, second, the variable toxicity of pollutants depending on their nature and third the spatio-temporal variability from local to global scales (Lelieveld et al., 2015). The effects of pollutants on the population also depend on the pollutants' composition, exposure time and the health condition of inhabitants. Lifestyle habits must be considered as well: for instance, Stieb et al. (2017) recommended reducing exposure time and physical activity outdoors under episodes of high concentrations of air pollutants. Moreover, the diverse combination of air pollutants can have additive or synergistic adverse effects on health (Curtis et al., 2006). Another factor hampering the attribution of deaths or different pathologies caused by air pollution is its association with high temperatures (Pearce et al., 2016). These authors indicate the relationship between high temperature short-term exposure and mortality risk. Therefore, heat extreme events are another aspect to take into account for population health under a changing climate.
Pollutants of largest concern for human health in Europe are particulate matter (PM) with a diameter lower than 2.5 µm (PM2.5), nitrogen oxides (NOx), sulfur dioxide (SO2), tropospheric ozone (O3) and carbon monoxide (CO) (Pozzer et al., 2012). Both O3 and PM are related with cardiorespiratory diseases and premature deaths. The most important pollutant for the mortality is PM, with a significant decrease in life expectancy projected for future scenarios (Héroux et al., 2015). PM exposure, especially to fine particles (PM2.5), may severely affect human health (Brook et al., 2010), piercing lungs or even pulmonary alveoli (Pope and Dockery, 2006). They cause cardiorespiratory symptoms and illnesses, increased asthma cases, heart attacks, strokes, and even premature deaths (Tagaris et al., 2010). Fine particles can cause damage, even in small concentrations (Beelen et al., 2014). Giannadaki et al. (2017) estimated premature deaths caused by PM2.5 to be 3.15 million per year in 2010 globally, while their estimation for Europe was around 173 000 premature deaths (about 5 % of the global rate). Gaseous pollutants like NOx or SO2 may enter the organism by inhalation and affect the respiratory system, irritating the respiratory system and inducing bronchoconstriction and asthma (Kampa and Castanas, 2007).
Recently, Im et al. (2018) estimated the health impacts of air pollution in Europe and the United States by using concentration inputs from different chemistry-transport models in the Economic Valuation of Air Pollution (EVA) system (Brandt et al., 2013a, b). In Europe, the total number of premature deaths (acute and chronic) and associated costs are calculated to be 414 000 and EUR 300 billion, respectively.
In addition, climate change alone may affect the concentration of these gaseous pollutants and particles through modifications in chemistry in the gaseous phase, transport, deposition and natural emissions (Jacob and Winner, 2009). Modelling approaches (together with remote sensing) may represent a good methodology to disentangle the role of climate change in air pollution and to obtain future projections of air quality (Jerrett et al., 2017).
Due to changes in future climatic conditions, air quality will significantly worsen, especially in southern Europe (Jiménez-Guerrero et al., 2013a). Several studies, combining atmospheric science, epidemiology, public health and economy, have tried to assess future air pollution, mitigation strategies and its relation with and repercussions on population health and associated costs. For instance, Geels et al. (2015) indicated that climate change effects together with a reduction of emissions will decrease premature deaths caused by air pollution. These authors estimated a reduction of acute mortality caused by PM of 36 %–64 % for the 2050s and 53 %–84 % for the 2080s and a decrease of 62 %–65 % and almost 80 % for the same future periods if chronic mortality is targeted. Short- and long-term exposure to pollutants can be reversible (Héroux et al., 2015). These authors suggest that mortality risk associated with air pollution can be reversible in as short a period as a year. The human body can partially recover when the exposure to the pollutant concentration ends. The cost for the health system of the impacts with lower severity and a larger number of inhabitants affected can exceed the impacts of more acute situations (higher concentration of pollutants) but a smaller number of the affected population (EEA, 2013).
Henceforth, this study focuses on the analysis of population health problems caused by regulatory pollutants in Europe and on their associated costs. First, the methodology followed aims to find the correlation (if any) between particles with a diameter under 10 µm (PM10) and total deaths and deaths caused by respiratory diseases over Europe using epidemiological data from the European Commission for the period 2001–2012. Then, in order to assess the impact of air pollution on health for present and future scenarios, data from a regional chemistry–climate model for a present climatology (1996–2015) will be used for estimating the cases and associated costs of some related pathologies and the differences found for a future climate scenario (2071–2100, RCP8.5).
2.1 Epidemiological study for present-day climate situation
According to Bashkaran et al. (2013), time series regression studies have been widely used in environmental epidemiology, notably in investigating the associations between exposures such as air pollution and health outcomes. Typically, for both exposure and outcome, data are available at regular time intervals. In our case, an epidemiological study for the present situation has been carried out, with data obtained from the European Commission (Eurostat; https://ec.europa.eu/eurostat/statistics-explained/index.php?title=Health, last access: 10 October 2018) corresponding to the years 2001–2012. Total deaths (TD) and deaths caused by respiratory diseases (DRD) have been analysed. The objective is to find the correlation between such mortalities and air pollution (in our study case, PM10, due to the short time series available for PM2.5). Although mortality data were available from 1994, the targeted period begins in 2001 due to the availability of PM10 data. As in Analitis et al. (2018), a first-order correlation structure was employed.
This study covers 25 European countries in total, with a non-homogeneous time coverage because of the different years of entry into the European Union. Taking into account the data availability, the countries selected for the epidemiology analysis were Austria, Belgium, Bulgaria, the Czech Republic, Denmark, Estonia, Finland, France, Germany, Hungary, Iceland, Ireland, Italy, Luxembourg, the Netherlands, Norway, Poland, Portugal, Romania, Slovakia, Slovenia, Spain, Sweden, Switzerland and the United Kingdom.
The correlation is not done directly on the raw data but the anomalies of mortality and PM10 series. These series are detrended in order to avoid spurious correlations. The detrending method is based on the first-time difference time series and is widely used in climate data analysis (e.g. Lobell and Field, 2007; Zhao et al., 2017). Linear regressions are performed with first differences in TD and DRD as the response variable and first differences of PM10 as the predictor variable. The regressions found have undergone a Mann–Kendall test in order to ensure their significance at 95 % confidence (p<0.05).
2.2 Regional chemistry–climate simulations
In addition, air quality model data are used in order to check the possible changes in pathologies and diseases between present and future scenarios of climate change. The simulations used for assessing air quality in this work span the period 1996–2015, as a present reference period, and 2071–2100 under the RCP8.5 scenario, as a future enhanced forcing scenario. This scenario is at the top of radiative forcing scenarios among all the Representative Concentration Pathways (Moss et al., 2010), and hence the largest effect on the concentration of air pollutants is expected. The differences between these two runs (present and RCP8.5) will provide the changes in future air quality.
The regional chemistry–climate model used was the Weather Research and Forecasting (WRF) model coupled with Chemistry (WRF-Chem; Grell et al., 2005). The spatial model configuration comprises a domain covering most of southern and central Europe with a resolution of 25 km. A total of 33 sigma levels are considered in the vertical, with the top of the atmosphere at 50 hPa. Historical simulations with WRF-Chem (1996–2015) were driven by the ERA-20C reanalysis (Poli et al., 2016), whose approximate resolution is 125 km, and the 200 km resolution CMIP5 experiment r1i1p1 MPI-ESM-LR (Taylor et al., 2012; Giorgetta et al., 2012a). No nudging was conducted in the experiments. The CMIP5 experiment RCP8.5-forced r1i1p1 MPI-ESM-LR run (Giorgetta et al., 2012b) was used for the scenario period (2071–2100).
Further information on the physico-chemical configuration of the model can be found in the scientific literature (Forkel et al., 2015; Palacios-Peña et al., 2017). A short description is presented here. The WRF-Chem setup used in these simulations includes the following options: the RADM2 chemical mechanism, the MADE/SORGAM aerosol module including some aqueous reactions, the Fast-J photolysis scheme, RRTMG shortwave and longwave radiation schemes and the Yonsei University (YSU) PBL scheme for the planetary boundary layer. Dry deposition follows the Wesely resistance approach, while wet deposition is divided into convective wet deposition and grid-scale wet deposition.
The modelling system for present-day climatologies has been extensively evaluated (Brunner et al., 2015). Despite the model skills with respect to air pollution modelling, data used for health estimations are widely discussed (Im et al., 2018). More information with respect to ozone and particulate matter (PM10) can be found in Im et al. (2015a, b).
In order to isolate the possible effects of climate change alone on air pollutants, unchanged anthropogenic emissions coming from the Atmospheric Chemistry and Climate Model Intercomparison Project (ACCMIP; Lamarque et al., 2010) are assumed. That allows the possible impacts to be anticipated if no mitigation strategies for regulatory pollutants are carried out and the climate penalty on air quality levels to be characterized. Natural emissions depend on climate conditions and therefore vary in present and future simulations. Hence, the effects of climate change on air pollution follow the methodology explained in Jiménez-Guerrero et al. (2013b), excluding possible changes in vegetation or land use.
2.3 Present and future impacts of air quality on pathologies
The impact of air quality on the following pathologies is investigated in this work: respiratory hospital admissions (RHA), cerebrovascular hospital admissions (CHA), congestive heart failure (CHF), chronic bronchitis (CB), lung cancer (LC) and premature deaths (PD). This last-mentioned pathology is related both to acute mortality and chronic mortality as defined in Brandt et al. (2013a, b).
For this, gridded population data were obtained from the SocioEconomic Data and Applications Center (SEDAC) of NASA (http://sedac.ciesin.columbia.edu, last access: 20 August 2018) at a resolution of 1 km2 and interpolated to the working grid. Since the time coverage of our analysis is 1996–2015, the Population Density v4 dataset for the year 2005 was used, based on counts consistent with national censuses and population registers. The population by cell is shown in Fig. 1. For the future scenario, the population has been kept constant in order to have an educated guess of the possible impacts due only to changes in air quality.
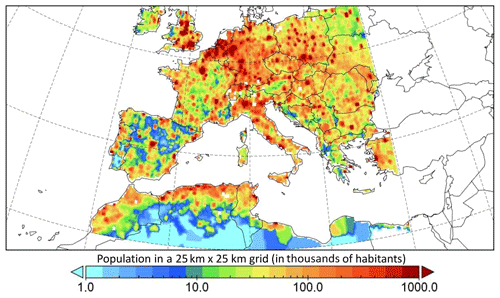
Figure 1Population in each grid cell (in thousands of habitants) (SEDAC population dataset for 2005).
In order to calculate the air quality impacts on the aforementioned pathologies, the methodology described in the EVA system (Brandt et al., 2013a, b, and references therein) has been used. In this work, we have utilized the population from SEDAC together with the WRF-Chem simulations and the exposure–response functions and economic valuations (taking the value of euros from the year 2006 as a basis) compiled in Brandt et al. (2013a) to estimate external costs of air pollution. The exposure–response coefficient and the valuation used are compiled in Table 1.
Table 1Pathology, exposure–response coefficients and economic valuation, taken from Brandt et al. (2013a). YOLL represents years of life lost. SOMO35 represents the sum of means over 35 ppb.
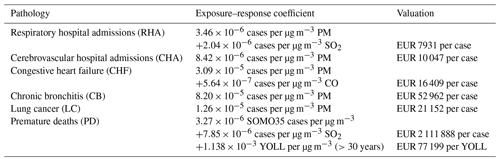
3.1 Statistical epidemiological study for the present situation
In the following section results from Eurostat data have been analysed. After detrending the data and calculating the anomalies for total deaths (TD), deaths by respiratory diseases (DRD) and PM10, correlations for each country between mortality and particles have been established. The results obtained are shown in Table 2; bold values indicate correlations significant at the 95 % confidence interval (p<0.05). Countries such as Germany, Hungary, Italy and Slovenia present a clear relation between such pollutants and both TD and DRD, with a high correlation in the anomaly series and high statistical significance. Meanwhile, countries such as the Czech Republic, Estonia and Switzerland present a notable statistically significant correlation only for TD–PM10 correlation. For the rest of the countries, mainly due to the short time series of data, either no significance (e.g. Bulgaria, Denmark, France, United Kingdom, Spain, Iceland) or a significantly low correlation (e.g. Austria, the Netherlands, Sweden) is obtained. The lack of correlation in some countries could be due to a large number of factors: among them, the high spatio-temporal variability of air pollution and mortality data (that is not taken into account in nationwide information) or some methodological limitations such as the large timescale of the data series or the limited number of years with data available hampering the significance of the series.
Table 2Correlation data between total deaths (TD) and PM10 (left column) and deaths by respiratory diseases (DRD) and PM10 (right column) for European countries. Bold values indicate a significant correlation (p<0.05).
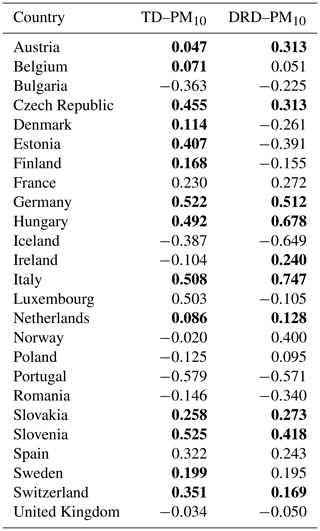
Hence, for several countries (despite the short data series) we can establish a relationship between mortality (especially TD) and atmospheric PM10 levels. Generally, the correlation TD–PM10 is higher than for DRD–PM10. As pointed out by several authors, the justification for these higher correlation values can be found in mortality by PM10 being caused by other pathologies that are not just respiratory, like cardiac or cerebrovascular issues. (Curtis et al., 2006; Tagaris et al., 2010; Pozzer et al., 2012).
3.2 Present and future scenario study on the pathologies and costs related to air pollution
This section discusses the results found for case distribution (number of people) with different pathologies caused by several air pollutants for a present climate situation (1996–2015) and the differences with a future RCP8.5 scenario (2071–2100). A summary of the global cases and associated costs is shown in Table 3.
Table 3Mean number of cases (in miles of cases) as associated costs (in millions of euros) per year for each pathology for present climate conditions (1996–2015) and variations in the future RCP8.5 scenario (2071–2100) for the entire domain of simulation.
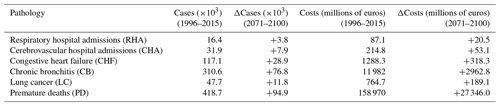
3.2.1 Respiratory hospital admissions (RHA)
The results for the European domain targeted (Table 3) indicate 16 400 cases of RHA per year in the 1996–2015 period, which will increase by 3800 cases in the RCP8.5 2071–2100 scenario. The external costs associated with this pathology represent EUR 87.1 million in the present climate, increasing in the future scenario by EUR 20.5 million (that is, an increase in cases and costs of +23 %).
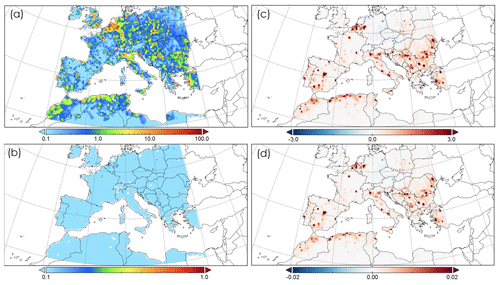
Figure 2(a) Present cases of respiratory hospital admissions (RHA) and (b) associated costs, in millions of euros. (c) Changes projected in RHA cases and (d) changes in costs (millions of euros) under the RCP8.5 scenario (2071–2100).
If local differences in RHA are established (Fig. 2), the highest number of present cases is found in the north of the study area, in countries such as Belgium and the Netherlands and in the western regions of Germany. Several hotspots appear in Paris and Bucharest, with an average of 200 cases per year and cell in 1996–2015. The cases with the lowest values are found in northern Spain and central France, with fewer than 0.25 cases per year and cell. With respect to the future (2071–2100) differences, and despite the global increase of the cases for Europe, strong differences appear between southern and northern Europe. Over central and northern Europe a slight decrease in RHA cases is projected (up to −4 cases per year cell) in localized cities located in Germany (Berlin) and Austria (Vienna). Meanwhile RHA cases may increase up to 122 per year and cell in southern and eastern Europe.
The costs follow the same spatial pattern as the cases, as expected. Despite the economic impacts on the society are limited, for several European megacities such as Paris, Cologne and Bucharest the present cost of RHA may reach up to EUR 1 million. For the future scenario, external costs are expected to increase in various European areas, especially in eastern Europe, an area which barely had costs associated with it in the present climatology, and in southern countries like Spain and Italy. In these last-mentioned areas, the costs increase by more than EUR 0.5 million per year and cell for 2071–2100 with respect to the 1996–2015 period.
RHA depends both on PM levels and SO2 (also included in the PD estimation). The areas with a larger impact on future RHA are those where power plants and other facilities of energy production are located. In that case, the impacts on eastern countries are higher due to the high sulfur-content fuels on which their economies are based (Colette et al., 2012; Pozzer et al., 2012; Geels et al., 2015). Henceforth, most hospital admissions occur in European megacities and eastern Europe, with poor air quality levels causing respiratory damage.
3.2.2 Cerebrovascular hospital admission (CHA)
Table 3 indicates a total number of 31 900 cases of RHA per year in the 1996–2015 period (external cost of EUR 214.8 million), with an associated increase for the whole domain of 7900 cases in the RCP8.5 2071–2100 scenario (increase in costs: EUR 53.1 million); that is, overall cases and costs will increase by +25 % at the end of the 21st century with respect to the present situation.
Regarding spatially distributed CHA, up to 400 cases per year are found in the city of Bucharest (Fig. 3), with an associated external cost of EUR 2.5 million. Many of the European megacities exceed the 100 cases for the present period. The countries with highest admission numbers are Belgium, the Netherlands and Germany. The northern half of Iberian Peninsula is the area least affected by CHA pathology. With respect to the differences with the 2071–2100 RCP8.5 scenario, the spatial pattern follows the same structure as for RHA cases shown in Fig. 2, as previously commented: a general increase in southern Europe (up to 270 cases per year and cell) and a light decrease mainly in cities of the Netherlands, Germany and Austria (up to 10 fewer cases per year and cell). The increase in costs in the future scenario can reach up to EUR +2 million in large cities such as Madrid, Bucharest and Paris, while the decrease in costs in areas with reduced CHA such as Berlin and Vienna does not exceed EUR −0.5 million.
3.2.3 Congestive heart failure (CHF)
As pointed out in Table 3, there are 117 100 cases of CHF per year over Europe in the 1996-2015 period, with an associated external cost of EUR 1.3 billion. Future climate change will increase the cases of CHF by +24 % (28 900 cases for the entire domain), also increasing the associated costs by the same percentage (increase of EUR 318.3 million in external costs for the period 2071–2100).
With respect to the spatial distribution of CHF (Fig. 4) within the target area, once again most of the CHF cases are located in Belgium and the Ruhr area; however outlying hotspots appear in the largest European cities (London, Paris, Madrid), with over 1000 cases per year in all the cities (costs > EUR 10 million). The highest number was found over the city of Bucharest (over 1500 cases per year in all the city, with an associated cost of over EUR 16 million). In this sense, CHF depends not only on particulate matter but also on CO levels; hence European megacities are the most important hotspots for this pathology due to their high vehicle traffic, which significantly contributes to CO and NOx emissions.
CHF cases are widely distributed throughout the study area, with values generally fewer than 10 cases per year and cell. The area with the lowest number of CHF cases for 1996–2015 is the northern Iberian Peninsula. For future projections, there is an increase of CHF close to 1000 cases in Bulgaria (Sofia and Craiova) and Moldova (Chişinău), with an associated increase in costs up to EUR 11 million. Once again, a decrease in cases (−36 cases per year cell) and associated costs (variations of EUR −0.4 million per year cell) is found in central Europe cities such as Vienna and Berlin for the 2071–2100 RCP8.5 scenario.
3.2.4 Chronic bronchitis (CB)
Table 3 indicates that CB cases exceed 310 600 cases per year in the whole target domain for the 1996–2015 period (cost EUR 12 billion), which will increase by 76 800 cases in the RCP8.5 scenario (2071–2100), also increasing the cost by EUR 3.0 billion in the whole of Europe covered by the simulation domain (+25 %).
The CB cases are unevenly distributed over Europe (Fig. 5). This pathology is distributed throughout the study area analogously to CHA (Fig. 3), since the exposure–response coefficient for CB and CHA only depends on the concentration of particulate matter. Cases exceed 1000 per year (over EUR 50 million) in cities such as Madrid, Paris, Brussels and Bucharest, with a maximum in the last-mentioned city of 3892 cases for the present period study (costs of EUR 150 million per year). Areas such as northern Italy and eastern Europe are largely affected in comparison with other pathologies previously mentioned. For the future scenario, the most affected areas are those reported for the present, with the exception of Chişinău (Moldova), Bucharest (Romania) and Sofia (Bulgaria), where future increases in CB cases may exceed 2600 (increase in external costs of over EUR 100 million). Meanwhile in some cities of Germany, Austria and the Czech Republic these pathology cases could decrease by up to almost 100 (EUR −4 million per year cell decrease in costs).
3.2.5 Lung cancer (LC)
Regarding LC, Table 3 estimates 47 700 cases per year in Europe for the present climatology, with an associated cost of EUR 765 million. The projected increase by 2071–2100 reaches +11 800 extra cases in the RCP8.5 scenario, with an increase in costs of EUR +189 million (+25 % of cases and cost increase).
The results for the spatial distribution of LC (Fig. 6) indicate that LC principally affects central and northern Europe, with widespread hotspots throughout the region. The maxima are found over European megacities (600 cases per year cell in the present climatology; costs over EUR 10 million). Countries with the widest affected areas are Belgium and the Netherlands, with a significant number of cases in Germany, northern Italy and southern Poland as well. An increase of over 400 LC cases per year and cell (associated increase in cost of EUR 6.5 million per year cell) is expected for the 2071–2100 period over southern Europe (Madrid, Rome, Bucharest, Sofia and Belgrade), with decreases of around 10 cases per year cell in cities of eastern Germany and eastern Austria. This decrease in LC (as also shown before for CB) found for more northern areas is strongly related to increases in precipitation found for the RCP8.5 scenario, which may reduce the levels of PM (Jiménez-Guerrero et al., 2013b).
3.2.6 Premature deaths (PD)
Finally, the variable PD covers chronic mortality and acute mortality as defined in Brandt et al. (2013a). Chronic mortality refers to mortality risks associated with long-term exposure and is quantified in years of life lost (YOLL, depending on PM2.5 concentration for population >30 years). Acute mortality depends on SO2 levels and SOMO35, which is estimated as the sum of means over 35 ppb for the daily maximum 8 h values of ozone.
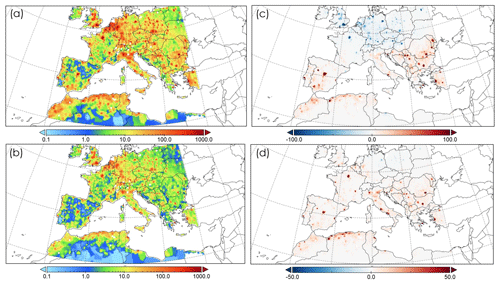
Figure 7(a) Present cases of premature deaths (PD) and (b) associated costs, in millions of euros. (c) Changes projected in PD and (d) changes in costs (millions of euros) under the RCP8.5 scenario (2071–2100).
Estimates of 418 700 cases per year in the target domain are provided in Table 3 for 1996–2015, with a huge associated cost (EUR 159 billion). The projected increase in the RCP8.5 for the years 2071–2100 reaches +94 900 extra cases, that is, an increase in costs of over EUR 27 billion (+17 % of cases and cost increase).
The dominant pathology over the entire domain (Fig. 7) is PD, especially over central Europe, Belgium, the Netherlands, Germany, Poland, Italy and Bulgaria. These countries have a high number of cases for the whole country. Hotspots are again located over large cities, exceeding 1000 cases per year cell and even reaching 4314 cases in several cities like Paris and London (associated external cost over EUR 700 million in these cities). For the future scenario (2071–2100) a clear difference between the northern half and the southern half of study area is depicted. While in southern European cities such as Madrid (Spain) and eastern European cities such as Belgrade (Serbia), Bucharest (Romania) and Sofia (Bulgaria) PD may increase up to 2400 cases per year (EUR +450 million in several megacities), in cities of countries such as Germany (Berlin, Hamburg), France (Paris) and the United Kingdom (London, Manchester, Newcastle) a decrease of more than 200 cases per year and cell is projected (reduction of costs over EUR 31.5 million per year cell).
This variation in premature deaths in southern Europe is mainly caused by the increase of O3 due to natural emissions, as a consequence of climate change alone and the accumulation in the Mediterranean of long-range transport of tropospheric ozone (and also particulate matter) (Jiménez-Guerrero et al., 2013a, b). Both pollutants are related with cardiorespiratory diseases and premature deaths (Tagaris et al., 2010; Geels et al., 2015). In contrast, more northern cities such as Berlin and Vienna will benefit from a better air quality in the future projections and a decrease in the number of cases and, in consequence, in the associated costs. This results from a large decrease in PM levels under an enhanced precipitation scenario (Jacob et al., 2018), as also detailed before for LC.
As proposed in the objectives of this contribution, a relationship was established between air pollutant levels and the impacts on several human pathologies. There are two reasons for us carrying out this study: (1) exceedances of the limit values regulated by the European directives or the World Health Organization for several pollutants over some European areas and (2) the scientific literature available showing a clear and increasing relationship between these exceedances and their impacts on human health (e.g. Brandt et al., 2013a, b; Im et al., 2018, whose results furthermore support the conclusions obtained in this work).
The statistical epidemiological study (corroborated later by the modelling results in this same contribution) identifies a clear relationship between pathologies and air pollution by PM, especially in central European countries. The highest coefficients of correlation in the epidemiological study are found for total deaths and particulate matter (TD–PM10) in Germany, Slovenia and the Czech Republic and for deaths caused by respiratory diseases (relationship DRD–PM10) in Hungary and Italy. The modelling study supports these conclusions, also highlighting that large cities and conurbations (especially in eastern Europe) are to be taken into account in order to analyse the impacts of air pollution on several pathologies and diseases. The pathologies considered significantly impact societal costs due to the damage to population health caused and are heterogeneously distributed over Europe, as are the impacts expected due to climate change. Several countries, such as Moldova and Bulgaria, which are not impacted by present air pollution in the modelled results, will strongly increase the cases and associated costs due to climate change alone.
Premature deaths are the most important pathology in the study area in terms of costs (EUR 158 billion per year, that will increase by 17 % in the future RCP8.5 2071–2100 projection) and cases (418 700 cases per year increased by 94 900 pear year in the future). This has been already stated by several authors (e.g. Héroux et al., 2015).
For the future scenario RCP8.5, we can conclude that, overall, all pathologies will increase in southern Europe (especially south-eastern Europe) because of the changes projected in PM and O3 (the latter is related to PD, which is expected to increase in RCP8.5 in the aforementioned areas). This scenario will be likely if no mitigation policies for anthropogenic regulatory pollutants are implemented in Europe. On the other hand, northern Europe will benefit from climate change through reduced levels of air pollution (mainly PM2.5), as also pointed out by Tagaris et al. (2010).
Finally, as a starting point for further studies, we should bear in mind that the ageing of the European population and the increase of city dwellers in future scenarios have not been taken into account in this study in order just to isolate the effect of climate change alone on the health of European citizens.
Epidemiological data can be freely obtained from the European Commission Eurostat page (https://ec.europa.eu/eurostat/statistics-explained/index.php?title=Health, Eurostat, 2018). The gridded population data were obtained from the SocioEconomic Data and Applications Center (SEDAC) of NASA (http://sedac.ciesin.columbia.edu, SEDAC, 2018). The modelling air quality data generated are accessible upon contact with the corresponding author (pedro.jimenezguerrero@um.es). MPI-ESM-LR historical experiment data are available at https://doi.org/10.1594/WDCC/RCM_CMIP5_historical-LR (Giorgetta et al., 2012a). CMIP5rcp85 data are available at https://doi.org/10.1594/WDCC/RCM_CMIP5_rcp85-LR (Giorgetta et al., 2012b).
PTC and PJG designed the analysis and wrote the manuscript with contributions from all co-authors. PTC and MMSV compiled the epidemiological data and conducted the statistical analysis. PJG conducted the numerical simulations. UI and JB provided access to the EVA model and, together with PTC and PJG, estimated cases and costs.
The authors declare that they have no conflict of interest.
The authors acknowledge the projects REPAIR-CGL2014-59677-R and ACEX-CGL2017-87921-R of the Spanish Ministry of the Economy and Competitiveness and the FEDER European program for support in conducting this research. The authors also acknowledge the support from the Air Quality Modelling Evaluation International Initiative (AQMEII).
This research has been supported by the Spanish Ministry of Economy and Competitiveness and the FEDER European program (grant no. ACEX-CGL2017-87921-R).
This paper was edited by Frank Dentener and reviewed by two anonymous referees.
Analitis, A., De Donato, F., Scortichini, M., Lanki, T., Basagana, X., Ballester, F., Astrom, C., Paldy, A., Pascal, M., Gasparrini, A., Michelozzi, P., and Katsouyanni, K.: Synergistic Effects of Ambient Temperature and Air Pollution on Health in Europe: Results from the PHASE Project, Int. J. Environ. Res. Pub. He., 15, 1856, https://doi.org/10.3390/ijerph15091856, 2018. a
Bashkaran, K., Gasparrini, A., Hajat, S., Smeeth, L., and Armstrong, B.: Time series regression studies in environmental epidemiology, Int. J. Epidemiol., 42, 1187–1195, https://doi.org/10.1093/ije/dyt092, 2013. a
Beelen, R., Raaschou-Nielsen, O., Stafoggia, M., Andersen, Z. J., Weinmayr, G., Hoffmann, B., Wolf, K., Samoli, E., Fischer, P., Nieuwenhuijsen, M., Vineis, P., Xun, W. W., Katsouyanni, K., Dimakopoulou, K., Oudin, A., Forsberg, B., Modig, L., Havulinna, A. S., Lanki, T., Turunen, A., Oftedal, B., Nystad, W., Nafstad, P., De Faire, U., Pedersen, N. L., Östenson, C.-G., Fratiglioni, L, Penell, J., Korek, M., Pershagen, G., Eriksen, K. T., Overvad, K., Ellermann, T., Eeftens, M., Peeters, P. H., Meliefste, K., Wang, M., Bueno-de-Mesquita, B., Sugiri, D., Krämer, U., Heinrich, J., de Hoogh, K., Key, T., Peters, A., Hampel, R., Concin, H., Nagel, G., Ineichen, A., Schaffner, E., Probst-Hensch, N., Künzli, N., Schindler, C., Schikowski, T., Adam, M., Phuleria, H., Vilier, A., Clavel-Chapelon, F., Declercq, C., Grioni, S., Krogh, V., Tsai, M.-Y., Ricceri, F., Sacerdote, C., Galassi, C., Migliore, E., Ranzi, A., Cesaroni, G., Badaloni, C., Forastiere, F., Tamayo, I., Amiano, P., Dorronsoro, M., Katsoulis, M., Trichopoulou, A., Brunekreef, B., and Hoek, G.: Effects of long-term exposure to air pollution on natural-cause mortality: an analysis of 22 European cohorts within the multicentre ESCAPE Project, Lancet, 383, 785–795, https://doi.org/10.1016/S0140-6736(13)62158-3, 2014. a
Brandt, J., Silver, J. D., Christensen, J. H., Andersen, M. S., Bønløkke, J. H., Sigsgaard, T., Geels, C., Gross, A., Hansen, A. B., Hansen, K. M., Hedegaard, G. B., Kaas, E., and Frohn, L. M.: Contribution from the ten major emission sectors in Europe and Denmark to the health-cost externalities of air pollution using the EVA model system – an integrated modelling approach, Atmos. Chem. Phys., 13, 7725–7746, https://doi.org/10.5194/acp-13-7725-2013, 2013a. a, b, c, d, e, f, g, h
Brandt, J., Silver, J. D., Christensen, J. H., Andersen, M. S., Bønløkke, J. H., Sigsgaard, T., Geels, C., Gross, A., Hansen, A. B., Hansen, K. M., Hedegaard, G. B., Kaas, E., and Frohn, L. M.: Assessment of past, present and future health-cost externalities of air pollution in Europe and the contribution from international ship traffic using the EVA model system, Atmos. Chem. Phys., 13, 7747–7764, https://doi.org/10.5194/acp-13-7747-2013, 2013b. a, b, c, d, e
Brook, R. D., Rajagopalan, S., Pope, C., Brook, J. R., Bhatnagar, A., Diez-Roux, A., Holguin, F., Hong, Y., Luepker, R. V., Mittleman, M. A., Peters, A., Siscovick, D., Smith S. C., Whitsel, L., and Kaufman, J. D.: Particulate Matter Air Pollution and Cardiovascular Disease: An Update to the Scientific Statement from the American Heart Association, Circulation, 121, 2331–2378, https://doi.org/10.1161/CIR.0b013e3181dbece1, 2010. a
Brunner, D., Savage, N., Jorba, O., Eder, B., Giordano, L., Badia, A., Balzarini, A., Baró, R., Bianconi, R., Chemel, C., Curci, G., Forkel, R., Jiménez-Guerrero, P., Hirtl, M., Hodzic, A., Honzak, L., Im, U., Knote, C., Makar, P., Manders-Groot, A., van Meijgaard, E., Neal, L., Pérez, J. L., Pirovano, G., José, R. S., Schroder, W., Sokhi, R. S., Syrakov, D., Torian, A., Tuccella, P., Werhahn, J., Wolke, R., Yahya, K., Zabkar, R., Zhang, Y., Hogrefe, C., and Galmarini, S.: Comparative analysis of meteorological performance of coupled chemistry-meteorology models in the context of AQMEII phase 2, Atmos. Environ., 115, 470–498, https://doi.org/10.1016/j.atmosenv.2014.12.032, 2015. a
Colette, A., Granier, C., Hodnebrog, Ø., Jakobs, H., Maurizi, A., Nyiri, A., Rao, S., Amann, M., Bessagnet, B., D'Angiola, A., Gauss, M., Heyes, C., Klimont, Z., Meleux, F., Memmesheimer, M., Mieville, A., Rouïl, L., Russo, F., Schucht, S., Simpson, D., Stordal, F., Tampieri, F., and Vrac, M.: Future air quality in Europe: a multi-model assessment of projected exposure to ozone, Atmos. Chem. Phys., 12, 10613–10630, https://doi.org/10.5194/acp-12-10613-2012, 2012. a, b
Curtis, L., Rea, W., Smith-Willis, P., Fenyves, E., and Pan, Y.: Adverse health effects of outdoor air pollutants, Environ. Int., 32, 815–830, https://doi.org/10.1016/j.envint.2006.03.012, 2006. a, b
EEA. European Environment Agency. Environment and human health, Joint EEA-JRC report Nr 5. Report EUR 25933 EN, available at: https://www.eea.europa.eu/publications/environment-and-human-health/ (last access: 18 October 2018), 112 pp., 2019. a
Eurostat: Health. Eurostat statistics explained, available at https://ec.europa.eu/eurostat/statistics-explained/index.php?title=Health, last access: 20 October 2018. a
Forkel, R., Balzarini, A., Baró, R., Bianconi, R., Curci, G., Jiménez-Guerrero, P., Hirtl, M., Honzak, L., Lorenz, C., Im, U., Pérez, J.L., Pirovano, G., San José, R., Tuccella, P., Werhahn, J., and Zabkar, R.: Analysis of the WRF-Chem contributions to AQMEII phase2 with respect to aerosol radiative feedbacks on meteorology and pollutant distributions, Atmos. Environ., 115, 630–645, https://doi.org/10.1016/j.atmosenv.2014.10.056, 2015. a
Geels, C., Andersson, C., Hänninen, O., Lansø, A., Schwarze, P., Skjøth, C., and Brandt, J.: Future premature mortality due to O3, secondary inorganic aerosols and primary PM in Europe–sensitivity to changes in climate, anthropogenic emissions, population and building stock, Int. J. Env. Res. Pub. He., 12, 2837–2869, https://doi.org/10.3390/ijerph120302837, 2015. a, b, c
Giannadaki, D., Lelieveld, J., and Pozzer, A.: The Impact of Fine Particulate Outdoor Air Pollution to Premature Mortality, in: Perspectives on Atmospheric Sciences, edited by: Karacostas, T., Bais, A., and Nastos, P., Springer Atmospheric Sciences, Springer, Cham, 1021–1026, https://doi.org/10.1007/978-3-319-35095-0_146, 2017. a
Giorgetta, M., Jungclaus, J., Reick, C., Legutke, S., Brovkin, V., Crueger, T., Esch, M., Fieg, K., Glushak, K., Gayler, V., Haak, H., Hollweg, H.-D., Kinne, S., Kornblueh, L., Matei, D., Mauritsen, T., Mikolajewicz, U., Müller, W., Notz, D., Raddatz, T., Rast, S., Roeckner, E., Salzmann, M., Schmidt, H., Schnur, R., Segschneider, J., Six, K., Stockhause, M., Wegner, J., Widmann, H., Wieners, K.-H., Claussen, M., Marotzke, J., and Stevens, B.: Forcing Data for Regional Climate Models Based on the MPI-ESM-LR Model of the Max Planck Institute for Meteorology (MPI-M): The CMIP5 Historical Experiment, World Data Center for Climate (WDCC) at DKRZ, https://doi.org/10.1594/WDCC/RCM_CMIP5_historical-LR, 2012a. a, b
Giorgetta, M., Jungclaus, J., Reick, C., Legutke, S., Brovkin, V., Crueger, T., Esch, M., Fieg, K., Glushak, K., Gayler, V., Haak, H., Hollweg, H.-D., Kinne, S., Kornblueh, L., Matei, D., Mauritsen, T., Mikolajewicz, U., Müller, W., Notz, D., Raddatz, T., Rast, S., Roeckner, E., Salzmann, M., Schmidt, H., Schnur, R., Segschneider, J., Six, K., Stockhause, M., Wegner, J., Widmann, H., Wieners, K.-H., Claussen, M., Marotzke, J., and Stevens, B.: Forcing data for Regional Climate Models Based on the MPI-ESM-LR model of the Max Planck Institute for Meteorology (MPI-M): The CMIP5rcp85 experiment, World Data Center for Climate (WDCC) at DKRZ, https://doi.org/10.1594/WDCC/RCM_CMIP5_rcp85-LR, 2012b. a, b
Grell, G. A., Peckham, S. E., Schmitz, R., McKeen, S. A., Frost, G., Skamarock, W. C., and Eder, B.: Fully coupled online chemistry within the WRF model, Atmos. Environ., 39, 6957–6975, https://doi.org/10.1016/j.atmosenv.2005.04.027, 2005. a
Héroux, M.-E., Anderson H. R., Atkinson, R., Brunekreef, B., Cohen, A., Forastiere, F., Hurley, F., Katsouyanni, K., Krewski, D., Krzyzanowski, M., Künzli, N., Mills, I., Querol, X., Ostro, B., and Walton, H.: Quantifying the health impacts of ambient air pollutants: recommendations of a WHO/Europe Project, Int. J. Public Health, 60, 619–627, https://doi.org/10.1007/s00038-015-0690-y, 2015. a, b, c
Im, U., Bianconi, R., Solazzo, E., Kioutsioukis, I., Badia, A., Balzarini, A., Baró, R., Bellasio, R., Brunner, D., Chemel, C., Curci, G., Flemming, J., Forkel, R., Giordano, L., Jiménez-Guerrero, P., Hirtl, M., Hodzic, A., Honzak, L., Jorba, O., Knote, C., Kuenen, J. J., Makar, P. A., Manders-Groot, A., Neal, L., Pérez, J. L., Pirovano, G., Pouliot, G., Jose, R. S., Savage, N., Schroder, W., Sokhi, R. S., Syrakov, D., Torian, A., Tuccella, P., Werhahn, J., Wolke, R., Yahya, K., Zabkar, R., Zhang, Y., Zhang, J., Hogrefe, C., and Galmarini, S.: Evaluation of operational on-line-coupled regional air quality models over Europe and North America in the context of AQMEII phase 2. Part I: Ozone, Atmos. Environ., 115, 404–420, https://doi.org/10.1016/j.atmosenv.2014.09.042, 2015a. a
Im, U., Bianconi, R., Solazzo, E., Kioutsioukis, I., Badia, A., Balzarini, A., Baró, R., Bellasio, R., Brunner, D., Chemel, C., Curci, G., van der Gon, H. D., Flemming, J., Forkel, R., Giordano, L., Jiménez-Guerrero, P., Hirtl, M., Hodzic, A., Honzak, L., Jorba, O., Knote, C., Makar, P. A., Manders-Groot, A., Neal, L., Pérez, J. L., Pirovano, G., Pouliot, G., Jose, R. S., Savage, N., Schroder, W., Sokhi, R. S., Syrakov, D., Torian, A., Tuccella, P., Wang, K., Werhahn, J., Wolke, R., Zabkar, R., Zhang, Y., Zhang, J., Hogrefe, C., and Galmarini, S.: Evaluation of operational online-coupled regional air quality models over Europe and North America in the context of AQMEII phase 2. Part II: Particulate matter, Atmos. Environ., 115, 421–441, https://doi.org/10.1016/j.atmosenv.2014.08.072, 2015b. a
Im, U., Brandt, J., Geels, C., Hansen, K. M., Christensen, J. H., Andersen, M. S., Solazzo, E., Kioutsioukis, I., Alyuz, U., Balzarini, A., Baro, R., Bellasio, R., Bianconi, R., Bieser, J., Colette, A., Curci, G., Farrow, A., Flemming, J., Fraser, A., Jimenez-Guerrero, P., Kitwiroon, N., Liang, C.-K., Nopmongcol, U., Pirovano, G., Pozzoli, L., Prank, M., Rose, R., Sokhi, R., Tuccella, P., Unal, A., Vivanco, M. G., West, J., Yarwood, G., Hogrefe, C., and Galmarini, S.: Assessment and economic valuation of air pollution impacts on human health over Europe and the United States as calculated by a multi-model ensemble in the framework of AQMEII3, Atmos. Chem. Phys., 18, 5967–5989, https://doi.org/10.5194/acp-18-5967-2018, 2018. a, b, c
Jacob, D. J. and Winner, D. A.: Effect of climate change on air quality, Atmos. Environ., 43, 51–63, https://doi.org/10.1016/j.atmosenv.2008.09.051, 2009. a
Jacob, D., Kotova, L., Teichmann, C., Sobolowski, S. P., Vautard, R., Donnelly, C., Koutroulis, A. G., Grillakis, M. G., Tsanis, I. K., Damm, A., Sakalli, A., and van Vliet, M. T. H.: Climate Impacts in Europe Under +1.5 ∘C Global Warming, Earth's Future, 6, 264–285, https://doi.org/10.1002/2017EF000710, 2018. a
Jerrett, M., Turner, M., Beckerman, B., Pope, C., van Donkelaar, A., Martin, R., Serre, M., Crouse, D., Gapstur, S., Krewski, D., Diver, W. R., Coogan, P., Thurston, G. D., and Burnett, R. T.: Comparing the Health Effects of Ambient Particulate Matter Estimated Using Ground-Based versus Remote Sensing Exposure Estimates, Environ. Health Persp., 125, 552–559, https://doi.org/10.1289/EHP575, 2017. a
Jiménez-Guerrero, P., Jerez, S., Montávez, J. P., and Trigo, R. M.: Uncertainties in future ozone and PM10 projections over Europe from a regional climate multi physics ensemble, Geophys. Res. Lett., 40, 5764–5769, https://doi.org/10.1002/2013GL057403, 2013a. a, b, c
Jiménez-Guerrero, P., Gómez-Navarro, J. J., Baró, R., Lorente, R., Ratola, N., and Montávez, J. P.: Is there a common pattern of future gas-phase air pollution in Europe under diverse climate change scenarios?, Climatic Change, 121, 661–671, https://doi.org/10.1007/s10584-013-0944-8, 2013b. a, b, c
Kampa, M. and Castanas, E.: Human health effects of air pollution, Environ. Pollut., 151, 362–367, https://doi.org/10.1016/j.envpol.2007.06.012, 2008. a
Lamarque, J.-F., Bond, T. C., Eyring, V., Granier, C., Heil, A., Klimont, Z., Lee, D., Liousse, C., Mieville, A., Owen, B., Schultz, M. G., Shindell, D., Smith, S. J., Stehfest, E., Van Aardenne, J., Cooper, O. R., Kainuma, M., Mahowald, N., McConnell, J. R., Naik, V., Riahi, K., and van Vuuren, D. P.: Historical (1850–2000) gridded anthropogenic and biomass burning emissions of reactive gases and aerosols: methodology and application, Atmos. Chem. Phys., 10, 7017–7039, https://doi.org/10.5194/acp-10-7017-2010, 2010. a
Lelieveld, J., Evans, J. S., Fnais, M., Giannadaki, D., and Pozzer, A.: The contribution of outdoor air pollution sources to premature mortality on a global scale, Nature, 525, 367–371, https://doi.org/10.1038/nature15371, 2015. a, b
Lobell, D. B. and Field, C. B.: Global scale climate-crop yield relationships and the impacts of recent warming, Environ. Res. Lett., 2, 014002, https://doi.org/10.1088/1748-9326/2/1/014002, 2007. a
Moss, R. H., Edmonds, J. A., Hibbard, K. A., Manning, M. R., Rose, S. K., Van Vuuren, D. P., Carter, T. R., Emori, S., Kainuma, M., Kram, T., and Meehl, G. A.: The next generation of scenarios for climate change research and assessment, Nature, 463, 747–756, https://doi.org/10.1038/nature08823, 2010. a
Palacios-Peña, L., Baró, R., Guerrero-Rascado, J. L., Alados-Arboledas, L., Brunner, D., and Jiménez-Guerrero, P.: Evaluating the representation of aerosol optical properties using an online coupled model over the Iberian Peninsula, Atmos. Chem. Phys., 17, 277–296, https://doi.org/10.5194/acp-17-277-2017, 2017. a
Pearce, J. L., Hyer, M., Hyndman, R. J., Loughnan, M., Dennekamp, M., and Nicholls, N.: Exploring the influence of short-term temperature patterns on temperature-related mortality: a case-study of Melbourne, Australia, Environ. Health, 15, 107, https://doi.org/10.1186/s12940-016-0193-1, 2016. a
Poli, P., Hersbach, H., and Dee, D.P.: ERA-20C: An Atmospheric Reanalysis of the Twentieth Century, J. Climate, 29, 4083–4097, https://doi.org/10.1175/JCLI-D-15-0556.1, 2016. a
Pope, C. A., Burnett, R., Krewski, D., Jerrett, M., Shi, Y., Calle, E., and Thun, M.: Cardiovascular mortality and exposure to airborne fine particulate matter and cigarette smoke: shape of the exposure-response relationship, Circulation, 120, 941–948, https://doi.org/10.1161/CIRCULATIONAHA.109.857888, 2009.
Pope, C. A. and Dockery, D. W.: Health effects of fine particulate air pollution: lines that connect, J. Air Waste Manage., 56, 709–742, https://doi.org/10.1080/10473289.2006.10464485, 2006. a
Pozzer, A., Zimmermann, P., Doering, U. M., van Aardenne, J., Tost, H., Dentener, F., Janssens-Maenhout, G., and Lelieveld, J.: Effects of business-as-usual anthropogenic emissions on air quality, Atmos. Chem. Phys., 12, 6915–6937, https://doi.org/10.5194/acp-12-6915-2012, 2012. a, b, c, d
Ravishankara, A. R., Dawson, J. P., and Winner, D. A.: New Directions: Adapting air quality management to climate change: A must for planning, Atmos. Environ., 50, 387–389, https://doi.org/10.1016/j.atmosenv.2011.12.048, 2012. a
SEDAC: SocioEconomic Data and Applications Center (SEDAC, NASA, available at: http://sedac.ciesin.columbia.edu, last access: 15 November 2018. a
Stieb, D., Shutt, R., Kauri, L., Mason, S., Chen, L., Szyszkowicz, M., Dobbin, A., Rigden, M., Jovic, B., Mulholland, M., Green, M., Liu, L., Pelletier, G., Weichenthal, S., Dales, R., and Luginaah, I.: Cardio-Respiratory Effects of Air Pollution in a Panel Study of Outdoor Physical Activity and Health in Rural Older Adults, J. Occup. Environ. Med., 59, 356–364, https://doi.org/10.1097/JOM.0000000000000954, 2017. a
Tagaris, E., Liao, K., DeLucia, A. J., Deck, L., Amar, P., and Russell, A. G.: Sensitivity of Air Pollution-Induced Premature Mortality to Precursor Emissions under the Influence of Climate Change, Int. J. Environ. Res. Pub. He., 7, 2222–2237, https://doi.org/10.3390/ijerph7052222, 2010. a, b, c, d
Taylor, K. E., Stouffer, R. J., and Meehl, G. A.: An overview of CMIP5 and the experiment design, B. Am. Meteorol. Soc., 93, 485–498, https://doi.org/10.1175/BAMS-D-11-00094.1, 2012. a
WHO, World Health Organization: Review of evidence on health aspects of air pollution. World Health Organization, REVIHAAP Project Technical Report, Copenhagen, Denmark, available at: http://www.euro.who.int/en/health-topics/environment-and-health/air-quality/publications/ 2013/review-of-evidence-on-health-aspects-of-air-pollution-revihaap-project-final-technical-report (last access: 20 October 2018), 302 pp., 2013. a
Zhao, C., Liu, B., Piao, S., Wang, X., Lobell, D., Huang, Y., Huang, M., Yao, Y., Bassu, S., Ciais, P., Durand, J.-L., Elliott, J., Ewert, F., Janssens, I.A., Li, T., Lin, E., Liu, Q., Martre, P., Muller, C., Peng, S., Peñuelas, J., Ruane, A.C., Wallach, D., Wang, T., Wu, D., Liu, Z., Zhu, Y., Zhu, Z., and Asseng, S.: Temperature increase reduces global yields of major crops in four independent estimates, P. Natl. Acad. Sci. USA, 114, 9326–9331, https://doi.org/10.1073/pnas.1701762114, 2017. a